Introduction
The incidence of breast cancer is continuously increasing worldwide and is the most common cause of cancer related death in female. Chemically induced breast cancer in rats by 7, 12-dimethylbenz[a]anthracene (DMBA) is most widely used model because it resembles the dietary intake of carcinogens in humans (1).
A large number of studies are in progress to identify environmental factors including diet that can block the initiation of carcinogenesis or suppress the promotion of tumor development. Turmeric belongs to the genus Curcuma and consists of plants that possessrhizoma and underground root like stems. Turmeric is of special importance to human diet without demonstrating excessive toxicity and has many pharmacologic activitiesi ncluding anti-inflammatory, antioxidant, and cancer-preventive properties (2). Turmeric and curcumin have been shown to significantly reduce forestomach tumors (3-5), duodena tumors (5), skin tumors (3), colon carcinogenesis in mice (5) and rats (6,7), and mammary tumors in rats (8). Several reports have described the anticarcinogenic activity of curcumin in breast cancer cell lines (9-11) and several in vivo studies have established the chemopreventive effect of curcumin against breast cancer (6,8).
However, most of studies describing anticarcinogenic effect have been done with curcumin, not whole turmeric. Turmeric contains three analogs of curcumin diferuloylmethane, also called curcumin, demethoxycurcumin (DMC), and bisdemethoxycurcumin (BDMC) (12). Although, curcumin was found to be the most potent of the three analogs in most system, some data showed that the mixture of three is more potent than either one alone (13). Furthermore, little is known of chemopreventive mechanisms of turmeric during in vivo mammary carcinogenesis.
In the present study, we investigated the effects of turmeric on DMBA-induced mammary carcinogenesis in rats to further evaluate turmeric for possible use of chemopreventive agents and also aimed to understand chemopreventive mechanisms of turmeric by measuring in vivo tumor cell proliferation and histological grade of tumors.
Materials and Methods
7,12-dimethylbenz(a)anthracene (DMBA), 5-Bromo-2-deoxyuridine (BrdU), streptavidin, 3,3’-diamino-benzidinehydrochloride (DAB), hematoxylin and eosin were purchased from Sigma-Aldrich (St. Louis, MO, USA). All other compounds were readily available commercial products. Anti-BrdU primary antibody was purchased from Dako (Carpenteria, CA, USA). Biotinylated horse anti-mouse secondary antibody was purchased from Vector (Burlingame, CA, USA). Permount was purchased from Fisher (FairLawn, NJ, USA).
Turmeric powder (50 g) was immersed in 5 L of 50% ethanol at room temperature for 24 hours, and this procedure was repeated three times. The extract was concentrated using a rotary evaporator (Daesin machine industry, Korea) at 60℃ and then freeze-dried. The yield obtained by ethanol extraction was 15.6%.
21 day old female Sprague Dawley rats were purchased from Central Laboratory Animal Inc. (Seocho-ku, Seoul, Korea). Rats were housed in a climate-controlled room (22± 2℃, 50±10% relative humidity) under a 12 h light/dark cycle and provided diet and water adlibitum. The rats were acclimated to a pellet diet for a week and then divided into three groups. Two groups were fed a modified AIN-93G control diet (NC, DC), the other with diet containing ethanol extract of turmeric (1.5% modified AIN 93G) (DT) until the end of experiment (Table 1). Food consumption and weight gain were measured every week.
AIN-93G Mineral mixture; Calcium carbonate anhydrous (40.04% Ca) 357 g, Potassium phosphate monobasic (22.76% P, 28.73% K) 196 g, Potassium citrate,tripotassium monohydrate (36.16% K) 70.78 g, Sodium chloride (39.34% Na, 60.66% CI) 74 g, Potassium sulfate (44.87% K, 18.39% S) 46.6 g, Magnesium oxide (60.32% Mg) 24 g, Ferric citrate (16.5% Fe) 6.06 g, Zinc carbonate (52.14% Zn) 1.65 g, Sodium meta-silicater 9H2O (9.88% Si) 1.45 g, Manganous carbonate (47.79% Mn) 0.63 g, Cupric carbonate (57.47% Cu) 0.30 g, Chromium potassium sulfater 12H2O (10.42% Cr) 0.275 g, Boric acid (17.5% B) 81.5 mg, Sodium fluoride (45.24% F) 63.5 mg, Nickel carbonate (45% Ni) 31.8 mg, Lithium chloride (16.38% Li) 17.4 mg, Sodium selenate anhydrous (41.79% Se) 10.25 mg, Potassium iodate (59.3% I) 10.0 mg, Ammonium vanadate (43.55% V) 6.6 mg, Ammonium paramolybdater 4H2O (54.34% Mo) 7.95 mg, Powdered sucrose 221.026 g. AIN-93G Vitamin mixture (22): Nicotinic acid 3 g, Ca pantothenate 1 g, Pyridoxine-HCl 0.7 g, Thiamin-HCl 0.6 g, Riboflavin 0.6 g, Folic acid 0.2 g, Biotin 0.02 g, Vitamin B-12 (cyanocobalamin) (0.1% in mannitol) 2.5 g, Vitamin E (all-rac-a-tocopheryl acetate) (500 IU/g) 15 g, Vitamin A (all-trans-retinyl palmitate) (500,000 IU/g) 0.8 g, Vitamin D-3 (cholecalciferol) (400,000 IU/g) 0.25 g, Vitamin K-1 (phylloquinone) 0.075 g, Powdered sucrose 974.655 g.
At 7 weeks of age, each animal in the DC and DT groups was administered DMBA (50 mg/Kg of body weight) in corn oil by oral gavage. Corn oil was administered to rats in the NC group. Beginning 1 week thereafter, rats were carefully palpated for mammary tumor weekly, and the number of tumors was recorded.
Rats were injected with BrdU (50 mg/Kg of body weight, i.p.) 2 h before being sacrificed by decapitation(14). Mammary tumors were removed and fixed in 10% neutral buffered formalin for 24 h, embedded into paraffin blocks, cut into 5 ㎛ sections, and then placed on SuperfrostPlus microscope slides (Fisher). The following day, the sections were placed in xylene in order to remove the paraffin and then rehydrated using a gradient consisting of decreasing concentrations of alcohol. The slides were then placed in 3.5 N HCl for 20 min and then trypsinized in 0.01% trypsin solution in phosphate-buffered saline. Next, the slides were placed in solution composed of 3% aqueous H2O2 to quench endogenous peroxidase activity. Sections were blocked using 10 or 5% horse serum. The sections were then incubated at 37℃ with anti-BrdU primary antibody diluted 1:200 for 30 min, followed by incubation with biotinylated horse anti-mouse secondary antibody diluted 1:250 for 20 min at room temperature. Detection was performed using streptavidin, and the color was developed using 3,3’-diaminobenzidine tetrahydrochloride (DAB) for 14 min. 2-Hematoxylin was used as a counterstain, after which the slides were preserved using Permount. The proliferation index was calculated as the percentage of BrdU-positive tumor cells.
A portion of the mammary tumor from each animal was fixed overnight in neutral buffered formalin (pH 7.5) and then embedded in paraffin. Five micron sections were stained with hematoxylin and eosin for light microscopy studies. The slides were examined and scored. In this study, we applied the Bloom and Richardson histological grading system as modified by Elston and Ellis (15). The grading was based on tubule formation, nuclear pleomorphism, and mitotic figure per high power field. Tubule formation was given a score of 1 when an overall evaluation of the tumor showed formation of tubules with visible lumens in a majority (>75%) of the lesion. When solid areas of tumor growth were admixed with a moderate degree of the tubular arrangements (10-75%), the tumor was given a score of 2. When the tumor showed minimal or no tubule formation (<10%), it received 3 points. In the assessment of nuclear pleomorphism, variation in the size and shape of the tumor nuclei was evaluated. A score of 1 was given to tumors with uniform or regular, small nuclei and those exhibiting minimal variation. Tumors with a moderate degree of variation in nuclear size and shape and occasional nucleoli were given 2 points. Those with marked variation in nuclear size and containing bizarre nuclei, often with one or more prominent nucleoli, were given 3 points. In determination of mitotic rate, at least 10 fields in the periphery or the most mitotically active part of the tumor were evaluated. The most mitotically active part of the tumor was evaluated. The tumor was scored 1-3 depending on the number of mitotic figures per 10 high power field.
All data are expressed as the mean±SD. Statistical analyses were performed using the SPSS program (SPSS Inc Version 17, Chicago, IL, USA). Group comparisons were carried out using variance analysis followed by Duncan's multiple range test. Statistical significance was considered at p<0.05.
Results and Discussion
Fig. 1 and 2 showed body weight and food intake respectively. DMBA treatment did not affect either body weight or food intake during the experimental period. However, turmeric significantly decreased bodyweight gain.
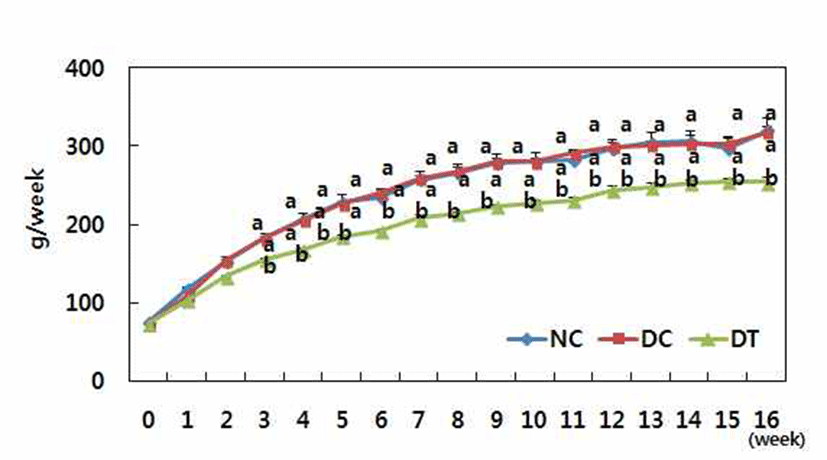
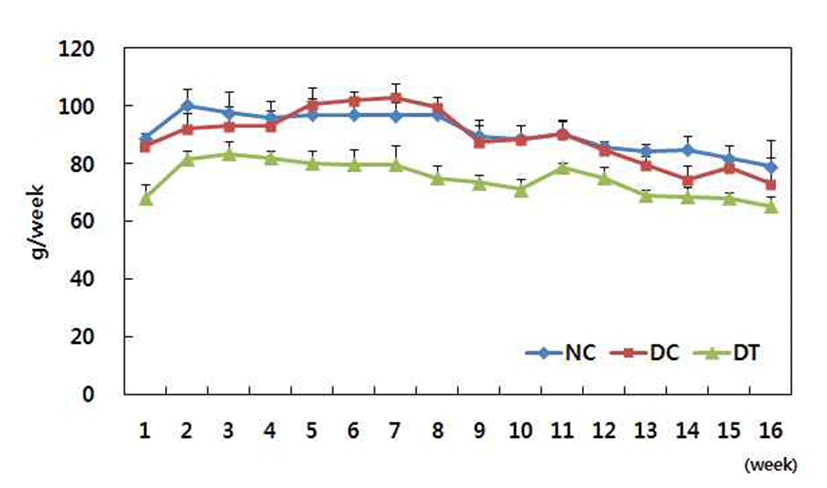
After 7 weeks of DMBA treatment, we observed tumors in the rats fed control diet, but tumors were observed after 8 weeks of DMBA treatment in the rats fed turmeric ethanol extract diet. 83.33 and 58.33% of the rats fed control diet and ethanol extract had tumors, respectively (Fig. 3). These results show that ethanol extract of turmeric delayed tumor onset and decreased tumor incidence. Many in vivo studies have established the chemopreventive effect of curcumin (diferuloylmethane), a major pigment of turmeric, against breastcancer. However, administration route and dose can affect the chemopreventive effect of curcumin. Intraperitoneal administration of curcumin at 100 and 200 mg/Kg doses prevented the development of the number of palpable mammary tumors (8). However, 2.0% curcuminin the diet had no effecton DMBA-induced mammary tumors (16). Another study showed that 1.6% curcumin diet was weakly effective in DMBA-induced mammary carcinogenesis (6). It was also reported that curcumin was highly effective as a chemopreventive agent in the colon model, whereas it was only weakly effective in the mammary model. It suggests that curcumin exhibits significant target organ specificity (6). The results of present study show that the progression of existing precancerous lesions and the growth of tumors in the mammary gland were retarded by dietary administration of turmeric, which is not very effective route for curcumin to prevent mammary carcinogenesis.
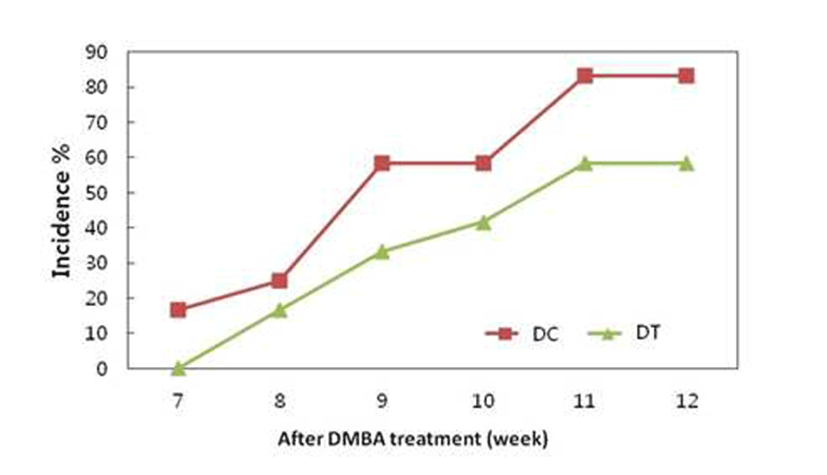
The number of tumors in each rat was recorded weekly after the first tumor appeared. Fig. 4 shows the inhibitory effect of turmeric extract on tumor multiplication. Tumor multiplication was significantly inhibited in rats fed ethanol extract compared to rats fed the control diet. It is known that number of tumors per rat may reflect the number of carcinogen initiated cells (17,18). Therefore, it is possible that turmeric ethanol extract inhibited the initiation of mammary carcinogenesis by DMBA. The inhibitory effects on initiation phase observed in the present study are in agreement with the inhibitory effects observed in DMBA induced mammary tumors in rats (19). Turmeric significantly decreased body weight in this study (Fig. 1). It has been suggested that a reduction in obesity could decrease breast cancer incidence (20,21). Several biological mechanisms have been postulated to explain the association of obesity with breast cancer risk. One of potential physiological mediators is hyperinsulinemia induced by obesity. We reported that turmeric prevented fat accumulation and insulin resistance in the rats and db/db mice fed high fat diet (22). Therefore anti-obesity effect of turmeric would associate with anticarcinogenic effect in the present study.
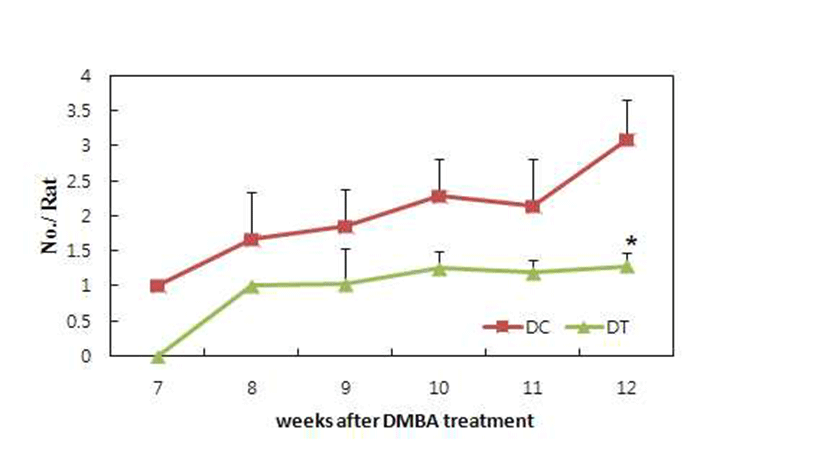
Turmeric extract decreased number of BrdU-positive cells in the tumors induced by DMBA treatment, which means tumor cell proliferation was inhibited by turmeric ethanol extract (Fig. 5). BrdU, a thymidine analog, incorporates itself into DNA during S phase of the cell cycle and BrdU incorporation is used as an index of cell proliferation (23, 24). Turmeric ethanol extract significantly decreased BrdU incorporation in the tumors compared to control diet. This result suggests that turmeric ethanol extract has inhibitory effects on tumor cell proliferation and this is one of mechanism for chemopreventive effect of turmeric. Huang et al. (16) reported that curcumin inhibited TPA-induced tumor promotion and suggested that the effect of curcumin might be linked to its strong inhibitory action on DNA and RAN synthesis. Limtrakul et al. (25) also reported an inhibitory effect of curcumin on mouse skin carcinogenesis and suggested that the effect of curcumin might be linked to its strong inhibitory action on DNA and RNA synthesis. The inhibitory effect of curcumin on DNA and RNA synthesis might contribute to the inhibition of tumor cell proliferation of turmeric.
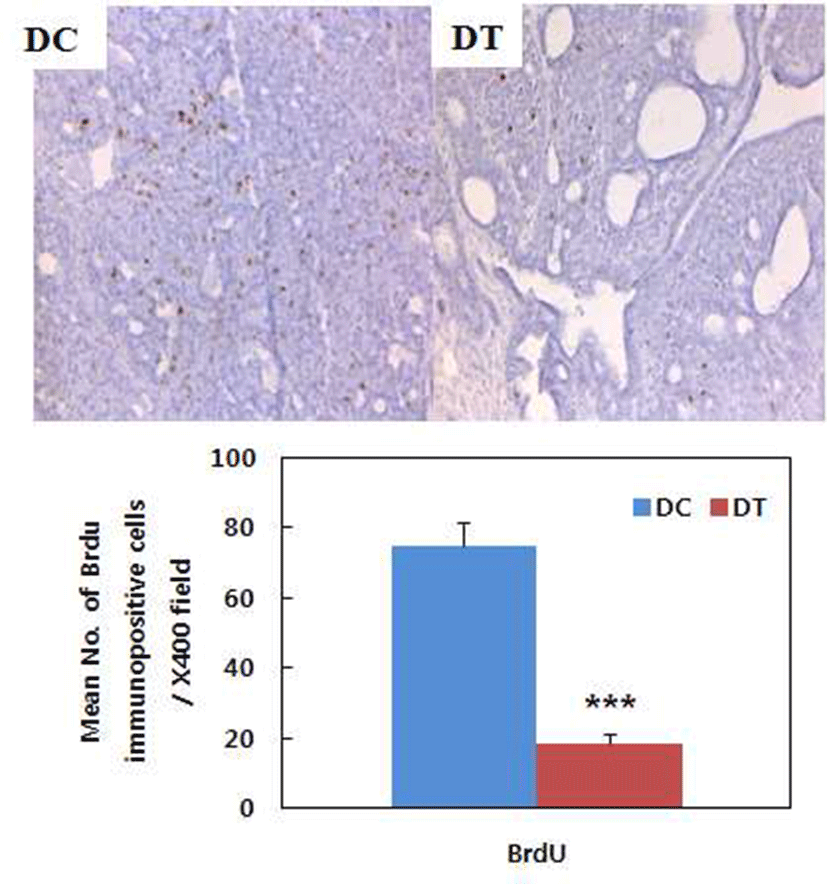
We examined the histological features of mammary tumors (Fig. 6). Tumors were graded based on tubule formation within the neoplasm, nuclear pleomorphism, and mitotic count per high power field (Table 2). Turmeric extracts significantly decreased tumor grades based on the degree of tubule formation. Mitotic rate of tumors in turmeric extract diet group was higher than that of tumors in control diet group, but the difference was not significant. Only tumor grade based on tubule formation was significantly decreased by turmeric ethanol extract.
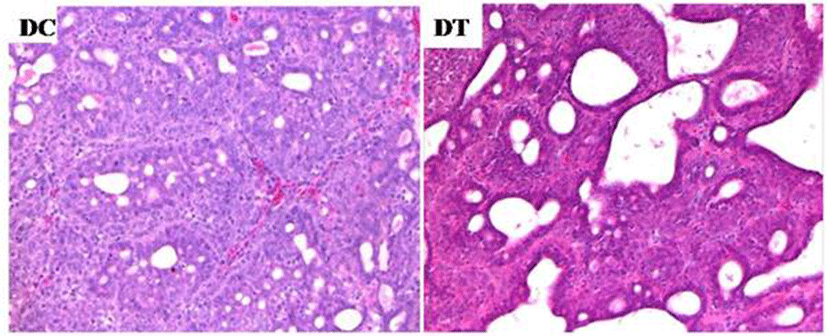
Tubule formation | pleomorphism Nuclear | Mitotic rate | |
---|---|---|---|
DC | 2.94±0.06 | 2.88±0.08 | 2.75±0.16 |
DT | 2.28±0.10** | 2.78±0.10 | 2.57±0.30 |