Introduction
The cherry tomato (Lycopersicon esculentum var. TY high Q) is an important crop, cultivated in the tropical and sub-tropical regions in the world because of its nutritional and economic value. Several bioactive compounds present in cherry tomatoes, including lycopene, phenolics, vitamin C, and small amounts of vitamin E, have been offered as part of a health-promoting diet (Giudice et al., 2015). Although the characteristic of tomatoes, such as a short life span, high yields, and year-round productivity, render them profitable to farmers (Noonari et al., 2015), they are prone to high post-harvest losses due to perishability, and so under unfavorable conditions, the ripe tomatoes exhibit a poor flavor and shortened storage life (Malundo et al., 1995). As a result, several post-harvest technologies, such as refrigeration storage (Batu and Thompson, 1998), modified atmosphere packaging (Park et al., 2012), heat treatment (Lurie and Klein, 1992), 1-methylcyclopropene application (1-MCP) (Cliff et al., 2009), calcium chloride (CaCl2) application (Senevirathna and Daundasekera, 2010), and UV-C irradiation (Mansourbahmani et al., 2017), have been developed to maintain the quality and shelf-life of tomatoes (Arah et al., 2016). However, the influences of intrinsic and extrinsic factors on tomato crops have yet to be examined in detail in this context (Luengwilai et al., 2012).
Fresh market cherry tomatoes are known to be of their best quality when the fruit is harvested at nearly the full-ripening stage (Roberts et al., 2002), however fully-ripe fruits rapidly soften, thereby leading to a shortened shelf-life (Krumbein, 2004; Kader et al., 1978). The ripening process of tomatoes is associated with exogenous or endogenous sources of ethylene (Blankenship and Dole, 2002), and so controlling ethylene biosynthesis, the freshness of harvested cherry tomatoes can be extended. More specifically, ethylene biosynthesis can be regulated through the application of 1-MCP, which binds to ethylene receptors and inhibits the various ripening-related biochemical changes, such as those attributed to cell wall degradation enzymes (Wills and Ku, 2002; Mostofi et al., 2003), lycopene accumulation (Njoroge et al., 1998; Opiyo and Ying, 2005), reducing the level of expansin mRNA (Hoeberichts et al., 2002), and phytochemical oxidation (Watkins, 2006). Thus 1-MCP has the ability to extend the storage life of cherry tomatoes. Moreover, the efficacy of application is higher at the pre-climacteric stage rather than in the climacteric stage. Consequently, the function of 1-MCP, which interrupts the progress of ripening, is as a temporary treatment during the advanced stages of ripening (Mir et al., 2004). Although it is widely used in horticultural industries due to its odorless and nontoxic nature in addition to a high efficacy at low dosages (Cliff et al., 2009; Blankenship and Dole, 2003), the required concentration and length of exposure must be varied depending on the type of species and variety to control the storage quality, shelf-life, and injury.
Recently, CaCl2 treatment has also been used to preserve horticultural crops and delay their softening, ripening, and deterioration, as well as for its role in sanitizing the food (Genanew, 2013). Calcium serves as a strong chelator in the linkage of cell wall constructions (Shiri et al., 2014), and as an inhibitor of cell wall degradation enzymes (Awang et al., 2013; Mansourbahmani et al., 2017). Moreover, calcium is an important mineral that influences the softness of fruit, its quality, post-harvest decay, and physiological disorders (Lurie, 2009).
To date, studies into the potency of exogenous chemicals on fruit have focused on the dosage procedures, such as concentration, treatment time, and fruit maturity, due to the differences in fruit response after treatment. In addition, the efficacy of separately employing CaC2 and 1-MCP on the storage-life and post-harvest quality of tomatoes has been proven by a number of studies (Watkins, 2006; Wills and Ku, 2002; Njoroje et al., 1998). However, the comparative or complementary effects of 1-MCP and CaCl2 on the physiological, biochemical, and cell wall metabolism responses of fully-ripe cherry tomatoes have received little attention. The objective of this study is therefore to evaluate the individual and synergistic effects of 1-MCP and CaCl2 on these factors in fully-ripe cherry tomatoes.
Materials and methods
“TY high Q” cherry tomato, a plant material obtained from Jeongeup APC (agricultural product processing center) was used and screened based on uniformity and freshness. The cherry tomato was sanitized using 100ppm of sodium hypochlorite for 2 min and then, directly washed using tap water. In order to optimize the concentration of 1-MCP and CaCl2 in cherry tomato, three doses (10, 100, 500 ppb) for 1-MCP, and three concentrations (0.5, 2.0, 6.0%) for CaCl2 treatments were investigated. The preliminary results showed that 500 ppb for 1-MCP fumigation and 2% for CaCl2 dipping were effective in delaying the discoloration and softening of cherry tomato. Thus, we choose the 500 ppb of 1-MCP and 2% of CaCl2 for this study.
Next, the fruits were randomly divided into four groups. Each was treated with one of the following pretreatment: (1) dipping into 2% CaCl2 solution for 2 min, (2) fumigating in 500ppb of 1-MCP (AgroFresh Solutions Inc., PA, USA) for 14 h in a 0.35 m3 airtight chamber at room temperature, (3) applying both 2% CaCl2 and 500 ppb of 1-MCP, and (4) control (no treatment). All treated fruits and control sample were placed in a plastic container (15 fruits/ container), and were stored in 10±1°C cold storage for 17 days, then transferred to 20°C chamber for additional four days. For parameter monitoring, three fruit containers were randomly selected every four days from each treatment unit. After sampling, half of the samples were used for physicochemical factors assessment, while the other half was prepared as the freeze sample for antioxidant activity, lycopene content and insoluble pectin content determination.
The respiration and ethylene production rates were measured using a gas chromatograph (GC, Bruker 450-GC, Bruker Crop., Billerica, MA, USA) equipped with a flame ionization detector (FID) to measure ethylene production (μg C2H4/kg°h) and a thermal conductivity detector (TCD) to measure carbon dioxide production (mL CO2/kg°h). Ten fruits from each treatment group were enclosed in 1 L air-tight container for 1 h. One milliliter of headspace gas was used for injection into the GC system. During the operation, the injector and column oven were set at 110 and 70°C, respectively, while the FID and TCD were set at 250 and 150°C.
The surface color was determined by using a colorimeter (Minolta CR-400, Osaka, Japan) after calibration with a white tile. CIE L, a, and b values were taken on fruit equatorial axes and calculated the hue angle. The firmness of fruit was measured by using a texture analyzer (TA Plus, Lloyd Instruments, Hamshire, UK) at a rate of 2 mm/sec with 5 mm diameter plunger, and expressed as Newton (N). The rate of weight loss was determined at two-days interval and expressed as a percentage of the initial weight. The squeezed tomato juice from each treatment was used for total soluble solid (TSS) determination with a refractometer (Pocket refractometer PAL-1, Atago, Tokyo, Japan), and titratable acidity (TA) was assessed by titration of 2 mL juice with 0.1 N sodium hydroxide to an endpoint of pH 8.2 using an auto pH titrator (Titroline easy, SCHOTT Instruments, Mainz, Germany). The ratio between TSS and TA was also used to evaluate the effect of treatments upon cherry tomato.
The frozen sample (2 g) was mixed with 70% methanol (10 mL) and extracted using an incubator shaker at 30°C for 1 h. After extraction, the methanol layer of each sample tube was separated through centrifugation at 12,000 rpm for 15 min at 4°C. The supernatant layer was then collected and filtered through a 2 μm of PTEF syringe filter, and employed for determination of the antioxidant activity using the 2,2-diphenyl-1-picrylhydrazyl (DPPH) assay, the 2,2’-azinobis3-ethylbenzothiazoline-6-sulfonic acid (ABTS) radical scavenging activity, and the total phenol content (TPC).
The free radical-scavenging activity of the hydrophilic tomato extract was evaluated using the stable DPPH radical, according to the method of Blosis (1958). The absorbance of the sample was monitored at 515 nm using a microplate spectrophotometer (Epcho 2, BioTek Instrument, Inc., Winooski, VT, USA) with methanol as a reference. A Trolox calibration curve (0-1,000 μmol μL-1; y = −0.0012x + 0.0429; r2= 0.992) was used to determine the number of μmol of Trolox per gram of fresh sample.
The ABTS radical scavenging activity of each sample was then determined according to a previous literature procedure (Re et al., 1999) with some modifications. For this purpose, the scavenging capacity was measured at 734 nm using a microplate spectrophotometer. A Trolox calibration curve (0-1,000 μmol/μL; y = −0.0013x + 0.0128; r2= 0.999) was used to determine the number of μmol of Trolox per gram of fresh sample.
According to the method of Swine and Hillis (1959), the TPCs were determined using the Folin-Ciocalteu reagent, whereby the sample absorbance was measured at 726 nm using a microplate spectrophotometer. The TPCs of the fruit samples were expressed as milligrams of gallic acid equivalents (GAE) per fresh weight gram of sample using a gallic acid calibration curve (0-500 μmol/μL; y = 0.0076x − 0.0844; r2 = 0.9956).
Lycopene extraction was performed on the method of Barba et al. (2006) with a slight modification. Frozen fine sample (0.6 g) was homogenized with 30 mL of hexane/ acetone/ethanol (1:1:1, v/v) solution and was placed on shaker for 30 min. The extracts were centrifuged at 3,000 ×g and 4°C for 10 min, then added with 15 mL of distilled water, and shaken for 5 min. The upper layer was transferred to new tube with wrapping aluminum foil. Finally, the concentration of lycopene at 503 nm was measured from lycopene extract using microplate spectrophotometer. Extraction solvent was used as blank and the amount of lycopene was expressed as mg/kg fresh weight of sample.
According to the method of Chatkaew and Kim (2013), crude cell wall was extracted step by step from 2 g of freeze-dried samples with 80% ethanol, chloroform: methanol (1:1, v/v), acetone, and finally 20 mM HEPESNaOH (pH 7.0) solution containing 1 unit/mL of α-amylase. These extracted crude cell walls were used for the insoluble pectin (polyuronide) assay. For polyuronide assay, 10 mg of crude cell wall was treated with 2 mL of cold sulphuric acid and incubated at room temperature for 12 hours. After incubation, the volume was made up to 25 mL with distilled water and vortexed.
Based on the methods of Gross and Wang (1984), the mixture of 0.4 mL of the diluted sample, 0.04 mL of potassium sulphamate/sulpamic acid solution (pH 1.6), and 2.4 mL of sulphuric acid containing 75 mM sodium tetraborate was boiled at 100°C for 20 min. After cooling it at room temperature for 10 min, 0.08 mL of 0.15% (v/v) m-phenylpnenol in 0.5% sodium hydroxide was added, then it was incubated 20 min at room temperature. After incubation, the extract was vortexed and used to determine the content of polyuronide with multimode microplate spectrophotometer at 525 nm. The content of polyuronide was expressed as mg/kg dry weight of sample by using calibration curve of galacturonic acid (0-400 μg/mL; y=0.1406x-0.1907; r2=0.9486).
This experiment was arranged with a completely randomized design, consisting of three pretreatment units and control. All the parameters were assessed in triplicate, and presented as means±standard deviation (SD). Data analysis was performed with SAS program version 9.1 (SAS Institute Inc., Cary, NC, USA). Significant differences among the treatments were taken at p<0.05 by using one way ANOVA, followed by Duncan’s multiple range tests as a post work.
Results and discussion
In this study, 1-MCP and CaCl2 treatments had no significant changes of the respiration rate and ethylene production in cherry tomatoes. The patterns of respiration rate and ethylene production in cherry tomatoes had just passed the peak of the climacteric phase since the initial storage (Fig. 1A) because the cherry tomatoes have reached its maturity. In observing the pattern of respiration, the rates in all treatments were similar and declined at 10°C storage period, except when all samples were transferred to room temperature storage and, the rates remarkably increased (Fig. 1A). Arah et al. (2015) suggested that ambient temperature promotes metabolic activities and it affects the respiration rate. Interestingly, even when the respiration rates of all cherry tomatoes had reached the climacteric phase, 1-MCP showed a suppression effect in the expression of respiration rate within 5 days after treatment (Fig. 1).
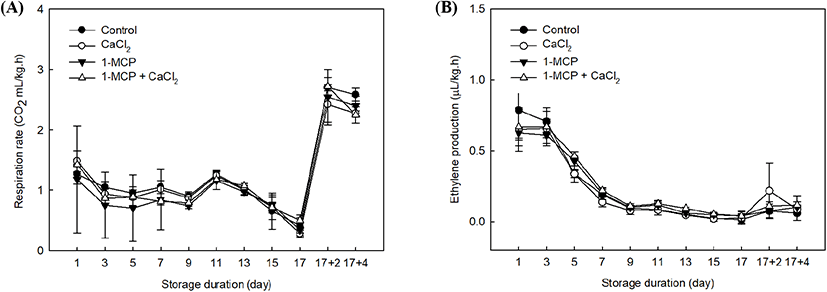
The ethylene productions in all treatment were gradually decreased until 17 days of storage because the full-ripe fruit were already passed a climacteric respiratory phase (Fig. 1B). However, the groups treated with 1-MCP or a combination of 1-MCP and CaCl2 contained lower ethylene concentrations than the control and the CaCl2-treated group until 9 days after treatment. We note that 1-MCP is a competitive inhibitor, which binds to the ethylene receptor instead of ethylene (Blankenship and Dole, 2003). In addition, exogenous 1-MCP application has been found to result in various post-harvest effects depending on the sample maturity, but its key role is as a competitive inhibitor, as mentioned above (Guillén et al., 2006; Opiyo and Ying, 2005). 1-MCP treatment of fully-ripe cherry tomatoes is therefore expected to inhibit the ethylene autocatalytic reaction.
The 1-MCP with CaCl2 treatment showed a slight suppression of respiration rate and ethylene production rate, especially when the treated samples were exposed to room temperature. Therefore, the expression levels of ethylene receptors after the climacteric phase can be examined further in a different study by using a combination treatment. The elevated ethylene climacteric peaks among treatments were finished because the plant material approached its full ripening stage (Fig. 1B).
The weight loss of each tomato sample undergoing the different treatments was measured at 2 days intervals, as outlined in Fig. 2. In the case of the treatments that preserved the fruit samples, an average weight loss of 1.46±0.04% was recorded, which is lower than that measured for the control (1.74±0.16%). More specifically, effective treatments for weight loss prevention were 1-MCP (1.44±0.06%) and CaCl2 (1.44±0.04%). Although Wills and Ku (2002) reported that 1-MCP treatment had no effect on weight loss in ripened “Clarion” tomatoes, we found that 1-MCP treatment did indeed prevent weight loss in the fully-ripe cherry tomatoes examined herein. In addition, the ripening response to 1-MCP in cherry tomatoes has previously been found to depend on the pretreatment conditions, such as the concentration, treatment time, and fruit maturity, and also on the subsequent storage conditions (Mir et al., 2004; Pristijono et al., 2017). Overall, previous studies have indicated that 1-MCP offers a beneficial effect in full-ripe tomato cultivars through the inhibition of ethylene production, lycopene degradation, and softening (Guillen et al., 2006; Opiyo and Ying, 2005). Similarly, CaCl2 treatment was also successful in limiting weight loss during storage due to the effects of calcium on the membrane functionality and integrity, whereby the loss of phospholipids and proteins is limited, and ion leakage is reduced (Lester and Grusak, 1999).
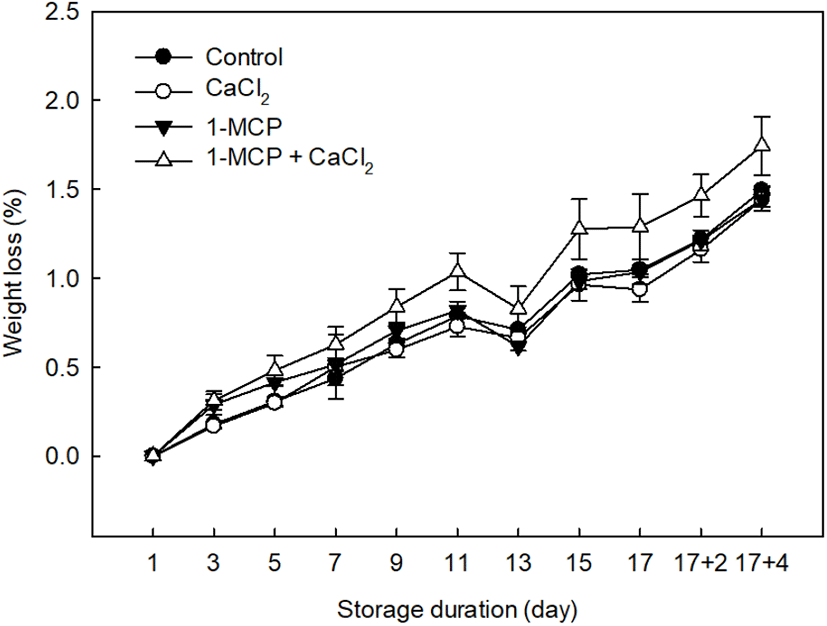
In Fig. 3, the firmness of cherry tomatoes under treatments was CaCl2: 7.62±0.16 N, 1-MCP: 7.42±0.33 N, 1-MCP with CaCl2: 7.24±0.33, and control: 6.66±0.25 N, respectively. The results indicate that all chemical treatments in cherry tomato had a higher firmness characteristic during storage. The maintenance of firmness in CaCl2 treatment was the best, and the possible factor is function of Ca2+ ion which binds together the strands of pectin that helps to maintain fruit firmness (Aghdama et al., 2012; Vicente et al., 2009). Therefore, CaCl2, and 1-MCP with CaCl2 treatments have calcium properties that maintain the cell wall composition of fruits compared with other treatments like 1-MCP. In general, pectin is a major component of cell wall in cherry tomato (Wakabayashi, 2000), and we evaluated the treatment performance upon the firmness through the assay of insoluble pectin in this study.
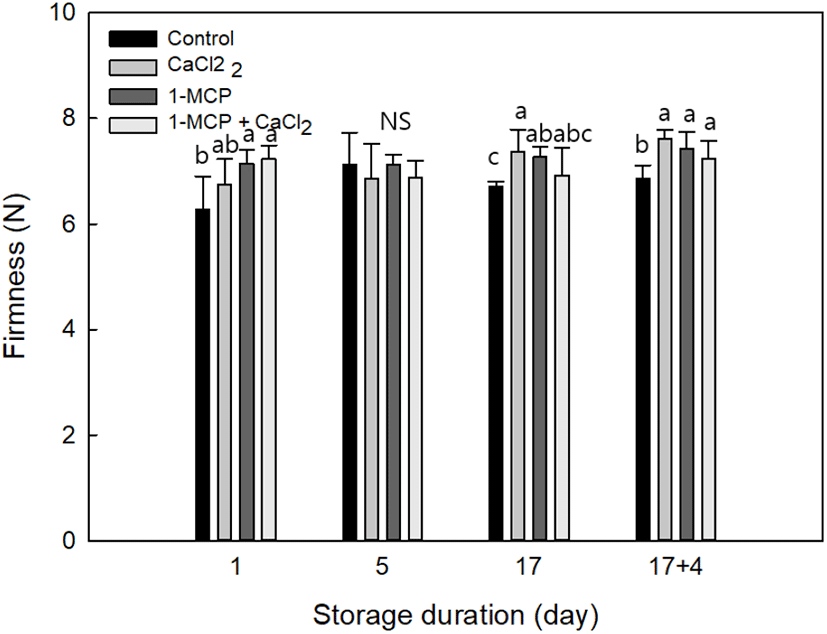
The contents of TSS and TA in the fruit remained relatively stable during storage (Table 1). In terms of the TSS contents, no significant differences were observed among the treated and control groups. The changes of TSS in control and all treatments had no significant differences. However, the treatments of 1-MCP and 1-MCP with CaCl2 in cherry tomatoes showed a lower reduction of TA content during storage whereas the TA contents of control and CaCl2 treated fruit were drastically declined within 5 days and 9 days after storage, respectively. It has been elucidated that the degradation of respiratory substrates, including organic acids and soluble sugars, is inhibited by exogenous 1-MCP at various ripening stages of tomato cultivars (Guillén et. Al., 2006; Opiyo and Ying, 2005). During the fruit ripening process, an increase in the TSS and decrease in organic acid content by activation of respiration metabolisms including glycolysis and citric acid cycle (TCA cycle), thus the (TSS/TA) ratio become an indicator of fruit maturity (Will et al., 1981). In this study, we also found that the inhibition of decrease of TA in full-ripe cherry tomatoes were affected by 1-MCP with CaCl2 as well as only 1-MCP treatment. Additionally, the TSS/TA ratio showed significant differences between control and all treatments. The lowest and highest TSS/TA ratios in fruits were observed in 1-MCP with CaCl2 treatment (4.02±0.32) and control (5.29±0.26), respectively. Interestingly, the 1-MCP with CaCl2 treatment showed a reducing effect of the TSS/TA ratio in full-ripe cherry tomato during storage (Table 1).
The external characteristic used to assess the ripening index is the change in skin color from green to red (Lopez Camelo and Gomez, 2004). As shown in Fig. 4, all treatments exhibited the similar patterns as an increase the level of a* value during storage period. The initial level of a* value (16.8±0.8) in control was immediately approached to the highest level (21.0±1.4) within five days of initial storage (Fig. 4B). In CaCl2 treatment, the level of a* value was also increased and reached the high level (17.8±2.6) at 9 days after storage. However, 1-MCP, and 1-MCP with CaCl2 treated fruit were still maintained the light red color until 17 days after storage with maximum a* value as 16.8±1.9 and 15.4±2.1, respectively. In these observations after storage at 10°C, 1-MCP with CaCl2 treatment showed more effective in delaying the discoloration as dark red color (Fig. 4A). The result value of a* in fruit during the storage of 10°C and 20°C was similar to the result of hue angle. Lowest level of hue angle along the storage duration was found in the control (Fig. 4B). In this study, when the control was approached to over-ripe stages, the value of a* were recorded more than 20 (Fig. 4). The value of a* in control fruit was gradually increased until 21.5 value at 5 days after treatment, but the value in 1-MCP with CaCl2 treated fruit was increased until 15.1 value for whole storage. Thus, 1-MCP with CaCl2 might be maintained the preference color of cherry tomato as the full-ripe fruit more than 12 days compared to control fruit. In this study, the combination treatment using 1-MCP and CaCl2 might be revealed a positive effect on postharvest quality by showing the lowest changes of weight loss, TSS/TA ratio, firmness, and color in full-ripe cherry tomatoes.
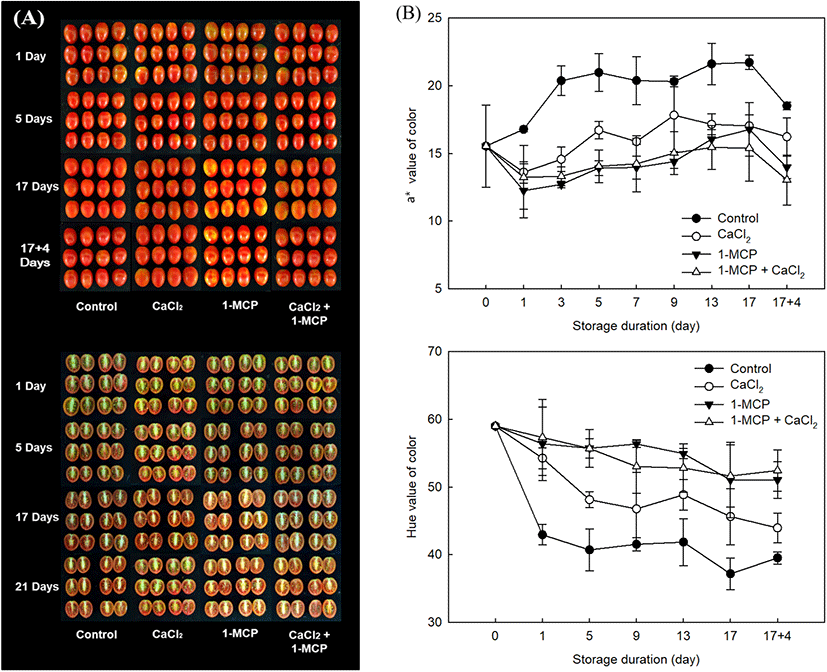
According to the analysis of variance, the positive effect of treatments upon the antioxidant activities of full-ripe cherry tomato was significant (p<0.05). Overall, increases in the antioxidant activities and TPCs were observed for all treatments (Tables 2 and 3). In this study, the 2% CaCl2 treated fruit were maintained the increase of antioxidant activities in comparison with 1-MCP, and 1-MCP with CaCl2 treated fruits during storage (Table 2). Mansourbahmani et al. (2017) also found that 2% of CaCl2 had a positive preservation effect of phenolic compound during storage. Furthermore, the TPCs of the control and the CaCl2 treatment in cherry tomatoes were found to be positively correlated to the DPPH radical scavenging activity (r = 0.894 for CaCl2 treatment, 0.833 for the control) and ABTS (r = 0.725 for CaCl2 treatment, 0.778 for the control, Table 3). Normally, an increase in the antioxidant activity during cold storage could be attributed to ripening and the metabolism of phenolic compounds, mainly flavonoids (Javanmardi and Kubota, 2006; Shahidi and Naczk, 1995).
In general, the antioxidant activity is significantly influenced by the maturity stage of the fruit, although hydrophilic antioxidant activities tend to remain relatively stable over the advanced ripening stages of tomatoes (Cano et al., 2003; Martinez-Valverde et al., 2002). Although Ilic et al. (2013) also reported that 1-MCP treatment reduced the lipophilic antioxidant activity of the pink and light red tomato fruit, but the hydrophilic antioxidant activity remained similar to condition before treatment. In this study, the antioxidant activities in the 1-MCP, and 1-MCP with CaCl2 treated fruit remained more stable than that of control fruit, even when the cherry tomatoes as the plant material were already approached to full-ripe maturity before treatment. These results therefore suggest that post-harvest treatment with 1-MCP, and 1-MCP with CaCl2 could be considered effective for prolonging the shelf lives of cherry tomatoes at the commercial mature stage.
In this study, the cherry tomatoes tended to have reached the nearly red maturity stage prior to treatment, with an average hue angle of 59.7±1.33. Therefore, the rapidly increasing rate of lycopene content in each treatment was found at the initial day of storage, and the rate changed and became slower at five days after storage. A significant difference in lycopene content was observed among treatments, particularly in the control and treatments. The highest lycopene content was found in control fruit and its value of lycopene content (34.9±4.0 mg/g fresh weight) was higher than CaCl2 (29.3±3.2 mg/g fresh weight), 1-MCP (27.9±2.4 mg/g fresh weight) and 1-MCP with CaCl2 treated fruit (25.1±4.6 mg/g fresh weight) at the end of storage. For 9 days after storage, the lycopene contents in control and CaCl2 treated fruit were drastically expressed more than 1-MCP and 1-MCP 1ith CaCl2 treated fruit (Fig. 5). The 1-MCP with CaCl2 showed the best-inhibited effect in the accumulation of lycopene content, followed by 1-MCP in second place (Fig. 5). The 1-MCP with CaCl2 treatment under the 10°C storage condition is an effective method to maintain the discoloration of fruit pericarp because that treatment inhibited the accumulation of lycopene content, and lengthened the ripening process of tomato (Fig. 4 and 5). It has also been reported in the literature that the accumulation of lycopene requires a continuous ethylene supply, and that CaCl2 and 1-MCP inhibit the accumulation of lycopene indirectly by preventing the build-up of ACC oxides, ethylene receptors, and the mRNA responsible for the expression of ACC synthase in tomato fruits (Hoeberichts et al., 2002; Lee et al., 2010; Opiyo and Ying, 2005). Indeed, CaCl2 has been previously employed to maintain the firmness of apples and oranges, among other fruits (Vicente et al., 2009; Aghdama et al., 2012). Our results therefore indicate that the treatment of fully-ripe cherry tomatoes with the combination of 1-MCP and CaCl2 can block the degradation of lycopene and other pigments.
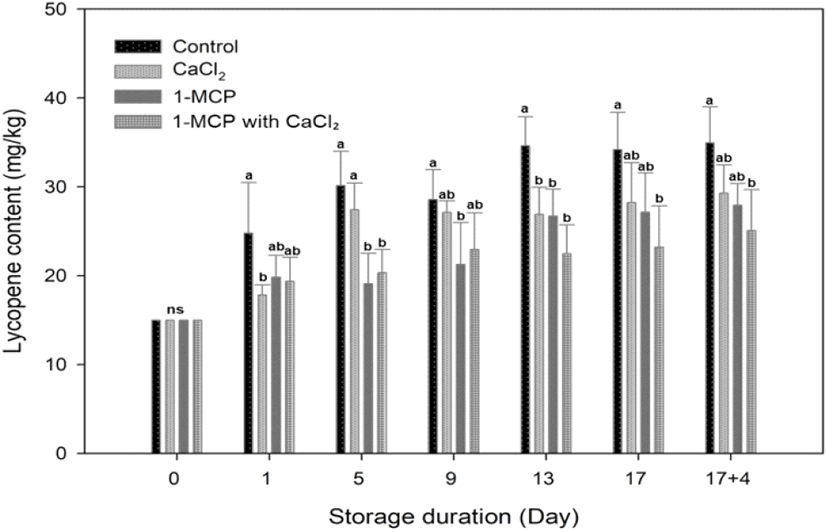
The redness level of a* value correlated positively with the lycopene content in this studied samples (r2=0.615). At 10°C stored fruits, the pattern of color a* value changes among treatments was similar to the accumulation of lycopene content, direction of a* value changes (Green to red) and pattern of lycopene accumulation oriented appositively; color value turned downward and lycopene content continued upward, during the additional four days of 20°C storage (Fig. 4 and 5). That result indicated that the changes along the green to red axis (a*) are so big that mask other two parameters changes and lead to misleading results under normal ripening condition (Lopez Camelo and Gomez, 2004). At the beginning of storage, the synthesis of lycopene builds up rapidly, and the rate becomes stable after 13 days storage to until the end of storage period (Fig. 5). Although lycopene synthesis is predominant between 12 and 32°C (Leoni, 1992), there was no significant difference of lycopene content from each treatment during changes in storage temperature from 10 to 20°C. Because all stored fruits proceed according to the ripening process and reach the fully ripened stage, thus a synthesis of lycopene condition became a stationary state. However, Choi et al. (2010) mentioned that lycopene content decreases in over-ripe tomatoes.
Pectin is major components in cherry tomato, and its level decreases as the ripening process progresses (Wakabayashi, 2000). During the storage, concentration of insoluble pectin level in control decreased gradually and a clear significant difference between treated fruit and control fruit was found at 5 days after storage (Fig. 6). Both CaCl2 and 1-MCP reagents were effective in maintaining the insoluble pectin concentration.
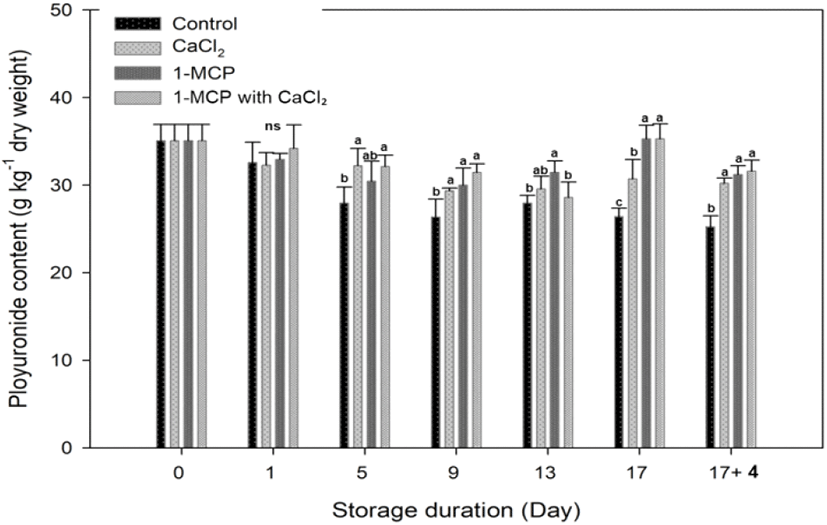
In CaCl2 treatment, a function of calcium is to link uronic acid groups through Ca2+ ions to strengthen the cell wall integrity and cell to cell cohesion (Lionetti et al., 2010; Sams et al., 1993), and to maintain the insoluble pectin concentration. The polyuronides solubilization during the ripening is correlated with the polygalacturonase (PG) activity that corresponds to the softening of fruit (Huber,1983; Smith et al.,1990; Wakabayashi et al., 2000). Brummell and Labavitch (1997) reported that PG-mediated polyuronide depolymerization oriented in reducing the fruit integrity and firmness at the advanced stage of ripening. As a 1-MCP treatment, 1-MCP directly inhibits the ethylene-mediated cell wall degrading enzyme activity like a PG (Mostofi et al., 2003). Therefore, 1-MCP with CaCl2 treatment maintained high polyuronide content at the 9, 17 and 17+4 days after storage, but there was no significant difference with other treatments.
In conclusion, overall, 1-MCP and 1-MCP with CaCl2 treated fruits were more effective in inhibiting the pericarp discoloration, maintaining the antioxidant activities and bioactive compound content, and insoluble pectin content compared to control and CaCl2 treated fruits. There was no significant different in respiration and ethylene production rate among treatments, but the 1-MCP with CaCl2 treatment showed a slight suppression of respiration rate and ethylene production at 20°C. Especially, 1-MCP with CaCl2 treatment had a synergic effect to retract the color and antioxidant activities, reduce the physicochemical, inhibit the lycopene accumulation, and delay the disruption of cell wall structure. In further, we should to conduct microbial analysis of cherry tomatoes after chemical treatments above mentioned.