Introduction
Increased reactive oxygen species and inflammation constitute the leading causes of the soaring rates of metabolic diseases such as atherosclerosis and diabetes (Yuan et al., 2019). The search for safe and efficacious approaches to combat continuously expanding oxidative stress, inflammation, and diabetes from natural products has emerged as a crucial strategy because they contain diverse biologically active compounds (Kammoun et al., 2018; Tong et al., 2015). Seaweeds, a promising marine resource, have been exploited and utilized traditionally as food supplements and for various medicinal purposes in Korea, China, Japan, and other Asian countries. Over the past two decades, researches on seaweeds in academia and industry around the world have made significant advances, and these plants have been shown to contain a variety of compounds with some of them exerting diverse physiological effects (Kellogg et al., 2014; Kellogg et al., 2015; Shanura Fernando et al., 2018).
Ulva lactuca, also known as sea lettuce, is characterized by a high content of carbohydrates, proteins, lipids, fiber, minerals, chlorophyll b, carotenoids, and other nutrients (Kammoun et al., 2018) and is greatly consumed in East Asia. It has good adaptability to different climates, high growth rates, high biomass per acre yield, and negligible requirements for fresh water (Mhatre et al., 2019). This marine seaweed is reported to have several pharmacological properties, including chemopreventive, hypolipidemic, and cardioprotective effects (Delgado-Roche et al., 2019; Hassan et al., 2011; Kammoun et al., 2018). For instance, the aqueous-ethanolic extract of U. lactuca inhibits the activity and expression of hepatic cytochrome P450 1A1 in mice exposed to benzo(a)pyrene (Delgado-Roche et al., 2019). Treating high-fat diet-fed rats with polysaccharide extracted from U. lactuca leads to a decrease in the levels of serum triglycerides and cholesterol (Hassan et al., 2011). In addition, the supplementation of mice fed cholesterol-rich diet with the U. lactuca extract effectively ameliorates hypercholesterolemia development and cardiac injury (Kammoun et al., 2018).
Phenolic compounds represent an important group of secondary metabolites in a large number of plant resources (Lu et al., 2018; Tong et al., 2019). Seaweed and its derived products are also considered valuable sources of phenolic compounds with significant beneficial effects for human health including antioxidant, antimicrobial, and anti-inflammatory activities (Kellogg et al., 2015; Kosanic et al., 2015). In the present study, the in vitro antioxidant and antiinflammatory activities as well as α-amylase- and α-glucosidase- inhibitory potentials of the U. lactuca extract were systematically investigated.
Material and methods
Ascorbic acid, bovine serum albumin, 3,5-dinitrosalicylic acid, dimethyl sulfoxide, 2,2-diphenyl-1-picrylhydrazyl (DPPH), ferric chloride, Folin & Ciocalteu’s phenol reagent, ferrous sulfate, gallic acid, hydrogen peroxide, lipopolysaccharides (LPS), nicotinamide adenine dinucleotide-reduced (NADH), nitro blue tetrazolium, phenazine methosulphate, trichloroacetic acid, α-amylase from human saliva (Enzyme Commission Number: 3.2.1.1), α-glucosidase from Saccharomyces cerevisiae (Enzyme Commission Number: 3.2.1.20), pnitrophenyl α-d-glucopyranoside, phosphate buffered saline, sulfanilamide, sodium salicylate, thiazolyl blue tetrazolium bromide, and N-(1-naphthyl) ethylenediamine dihydrochloride were purchased from Sigma-Aldrich Chemical Co. (St. Louis, MO, USA). Dulbecco’s modified Eagle medium, fetal bovine serum, and penicillin-streptomycin were purchased from Thermo Fisher Scientific Inc. (Waltham, MA, USA). Acarbose hydrate was purchased from Tokyo Chemical Industry Co., Ltd. (Tokyo, Japan). Viscozyme® L and Flavourzyme® 1,000 L were purchased from Novo Co. (Novozyme Nordisk, Bagsvaerd, Denmark).
The dried U. lactuca was obtained from Jindo, Jeonnam, South Korea, and was ground in a grinder (DA505 lon power, Daesungartlon Co., Seoul, Korea). The powder samples were defatted twice with n-hexane at a 1:5 ratio (w/v) for 30 min using a magnetic stirrer. The defatted seaweed samples were air-dried for 12 h at 35°C and stored at −20°C until subsequent extraction.
For the preparation of the U. lactuca extract, the defatted seaweed sample (1 g) was mixed with distilled water (35 mL) in 50 mL Falcon tubes. The tube was then placed into a thermostatic digital water bath and incubated for 5 min. The pH of the mixture was adjusted using 1 N NaOH or 1N HCl to the optimal pH for the enzymes used. The enzymes, viscozyme and flavourzyme (100 μL/g dry seaweed weight, respectively), were added to the solution and the enzymolysis reactions were performed for 134 min in a thermostatic digital water bath. Next, ethanol was added and the tube was transferred to an ultrasonic cleaning bath (JAC 4020P, KODO Technical Research Co., Hwaseong, Korea) for ultrasonic extraction. The ultrasonic procedure was conducted at a frequency of 40 kHz and an ultrasonic power of 300 W at 70°C for 50 min. After extraction, the supernatant was collected in a separate bottle by centrifugation (4,000 rpm, 10 min) and the residue was again subjected to ultrasonic extraction under the same conditions. The collected supernatant was combined and concentrated with a rotary agitation (CCA-1100, Sunileyela co., Seoul, Korea) at 50°C (yield of the U. lactuca extract: 85.44%).
The TPC was determined by the Folin-Ciocalteu method as described by Singleton and Rossi (1965). The U. lactuca extract (50 μL) was made to 4 mL with distilled water in a tube and then mixed thoroughly with Folin-Ciocalteu’s phenol reagent (250 μL, 1 N) using a vortex mixer. After 8 min, 750 μL of 15% sodium carbonate solution was added and the reaction mixture was allowed to stand at 40°C in the dark for 30 min. The absorbance was measured at 765 nm using a spectrophotometer (Model 8453, Agilent Technologies, Inc., Palo Alto, CA, USA). The total phenolic content of the extract was determined using an equation obtained from gallic acid calibration curve (y=0.8054x+ 0.0056, R2=0.9997) and was expressed as mg of gallic acid equivalents/g of extract.
The DPPH radical scavenging capacity of the extract was measured based on the method described by Shimada et al. (1992) with slight modifications. DPPH solution (500 μL, 0.1 mM) in 95% ethanol was mixed with the U. lactuca extract solution (500 μL) and the mixture was incubated in the dark at ambient temperature for 30 min. The absorbance of the mixture was measured at 517 nm using a spectrophotometer (Model 8453, Agilent Technologies, Inc.). Ascorbic acid was used as a reference antioxidant. The DPPH scavenging capacity of the U. lactuca extract was obtained from the formula (1):
Abs0: absorbance without samples
Abs1: absorbance in the presence of the samples
The hydroxyl radical scavenging activity was assayed according to the method of Smirnoff and Cumbes (1989). The reaction mixture contained 500 μL of 1.5 mM ferrous sulfate, 150 μL of 20 mM sodium salicylate, 500 μL of the U. lactuca extract solution, and 350 μL of 6 mM hydrogen peroxide. After incubation for 1 h at 37°C in a water bath, the absorbance of the reaction mixture was determined at 562 nm using a spectrophotometer (Model 8453, Agilent Technologies, Inc.). The scavenging activity of the U. lactuca extract on hydroxyl radical was obtained using formula (1).
The reducing power of U. lactuca extract was determined using the method described by Yen and Chen (1995). The U. lactuca extract (100 μL) was mixed with 2.5 mL of 0.2 M phosphate buffer (pH 6.6) and 1% potassium ferricyanide. After incubation at 50°C for 20 min, 2.5 mL of 1% trichloroacetic acid was added to the mixture; then the mixture was centrifuged at 3,000 rpm for 10 min. Next, 2.5 mL of distilled water and 0.5 mL of 0.1% ferric chloride were added to 2.5 mL of supernatant. The absorbance of the mixture was measured at 700 nm after mixing thoroughly.
The method described by Nishikimi et al. was used to determine the superoxide anion scavenging activity of the U. lactuca extract (Nishikimi et al., 1972). Here, 1.5 mL of the U. lactuca extract solution was mixed with 0.5 mL of NADH (468 μM) and 0.5 mL of nitro blue tetrazolium (300 μM). After vigorous shaking, 0.5 mL of phenazine methosulphate (60 μM) was added, and the reaction mixture was allowed to stand at room temperature for 5 min. The absorbance was then determined at 560 nm after 5 min. The superoxide anion radical scavenging capacity of the U. lactuca extract was calculated using formula (1).
α-Amylase inhibition activity was determined according to a previously described method (Bernfeld, 1955), in which 1% soluble starch solution prepared with sodium phosphate buffer (0.02 M, pH 6.9) containing NaCl (6.7 mM) was used as substrate. A reaction mixture consisting of 1.0 mL of substrate, 0.1 mL of distilled water, 0.2 mL of the U. lactuca extract solution, and 0.7 mL of enzyme solution, was incubated at 25°C for 20 min. One milliliter of 3,5-dinitrosalicylic acid was added and the reaction mixtures were boiled for 15 min. After cooling in an ice bath, 9 mL of distilled water was added; the absorbance was then determined at 540 nm. Acarbose (0.1 and 0.2 mg/mL) and water were used as a positive and negative control, respectively. The percentage of α-amylase inhibition was calculated using formula (1).
The inhibitory activity of the U. lactuca extract against α-glucosidase activity was evaluated as described previously (Tong et al., 2014). The U. lactuca extract solution (0.1 mL) was mixed with 0.79 mL of sodium phosphate buffer (pH 7.0) containing 0.2% bovine serum albumin, 0.02% sodium azide, and 10 μL of enzyme solution. After a 5-min pre-incubation at 37°C, p-nitrophenyl α-d-glucopyranoside solution (0.1 mL, 5 mM) was added and the mixture was incubated at 37°C for 10 min. The reaction was terminated by the addition of Na2CO3 (1 mL, 0.1 M) and the absorbance was then determined at 405 nm. The percentage of α-glucosidase inhibition was calculated using formula (1).
The murine macrophage RAW 264.7 (KCTC, KCLB 40071, Seoul, Korea) cell line was cultured in Dulbecco’s modified Eagle medium supplemented with 10% fetal bovine serum and 1% penicillin-streptomycin at 37°C in a 5% CO2 incubator (Model CCL170B8FD, Esco Technologies, Inc., Louis, MO, USA). For the cell viability assays (Tong et al., 2019), RAW 264.7 cells were seeded in 96-well plates at a starting density of 4×104 cells/well and cultured for 24-48 h. The cells were treated with various concentrations of extract solution for 24-48 h and MTT was then added to a final concentration of 0.1 mg/mL. After incubation for 4 h, the supernatant was removed; then the formazan crystals were dissolved in dimethyl sulfoxide. Absorbance was measured at 540 nm using a microplate reader (Epoch 2, BioTek Instruments, Inc., Winooski, VT, USA).
The RAW 264.7 cells were seeded at a density of 4×104 cells/well in 96-well plates and cultured at 37°C and 5% CO2. Culturing medium was replaced with fresh media after 24-48 h and the cells were treated with various concentrations of extract solution or LPS. After incubation for 24-48 h, 100 μL of cell culture medium was mixed with 100 μL of Griess reagent in wells of a 96-well plate. The absorbance was measured at 540 nm using a microplate reader (Epoch 2, BioTek Instruments, Inc.). The amount of nitrite in the media was calculated from a sodium nitrite (NaNO2) standard curve (Y=0.0115X+0.0386, R2=0.9995).
Data are expressed as mean±SD. Differences between the groups were determined using one-way ANOVA (Duncan’s Multiple Range Test) and p values<0.05 were considered statistically significant. Data were analyzed using IBM SPSS (version 21.0 for windows, SPSS Inc., CO, USA). EC50 values were obtained through regression analysis using Origin Pro 8.5 (Origin Lab Corporation, Northampton, MA, USA).
Results and discussion
In this study, to obtain the phenolic-enriched extract, the lipids in the U. lactuca sample were removed before enzymatic hydrolysis. It has been reported that the application of enzymes during the extraction process can enhance the release of secondary plant metabolites and preserve the bioactive properties of the extracts (Wijesinghe and Jeon, 2012). Enzymatic extraction also increases the extractability of bioactive compounds from seaweeds. The cell walldegrading enzymes help to disrupt or weaken the cell wall, breaking down complex interior storage materials, resulting in the release of the intracellular bioactive compounds (Siriwardhana et al., 2008). In the present study, the TPC value of the U. lactuca extract was 7.72±0.30 mg of gallic acid equivalents/g of extract. The U. lactuca extract obtained here had a relatively lower TPC value compared to that reported in previous studies on U. lactuca methanol-water (4:1) extracts (Aslan et al., 2019). Differences in extraction methods might partly account for the differences in TPC values among different studies. The positive and direct correlation between the TPC of seaweed extracts and their antioxidant activities is well documented (Gonzalez-Ballesteros et al., 2019; Kosanic et al., 2015; Siriwardhana et al., 2003; Tong et al., 2014). Therefore, we next determined the in vitro antioxidant effects of the U. lactuca extract using various in vitro antioxidant bioassay systems, specifically, the scavenging capacities of the U. lactuca extract against DPPH, hydroxyl, and superoxide anion radicals as well as reducing power.
DPPH, a stable organic free radical, has been viewed as a useful reagent to measure the free radical scavenging capacity of antioxidant materials. As seen in Fig. 1A, the DPPH radical scavenging capacity initially increased as the concentration of the U. Lactuca extract increased and then consistently plateaued. The EC50 (the effective concentration of 50% inhibition) value of the U. lactuca extract was 0.52 mg/mL. It was noted that the U. lactuca extract obtained here had a relatively stronger DPPH radical scavenging activity compared to that reported in previous studies where aqueous or 96% ethanolic extracts were used (Gonzalez-Ballesteros et al., 2019; Sabeena Farvin and Jacobsen, 2013). The aqueous extract of U. lactuca was previously found to have an EC50 value of 153.8 mg/mL (Gonzalez-Ballesteros et al., 2019). Further, Sabeena Farvin and Jacobsen (2013) reported an EC50 of 1,266.7 μg/mL for a 96% ethanolic extract of U. lactuca.
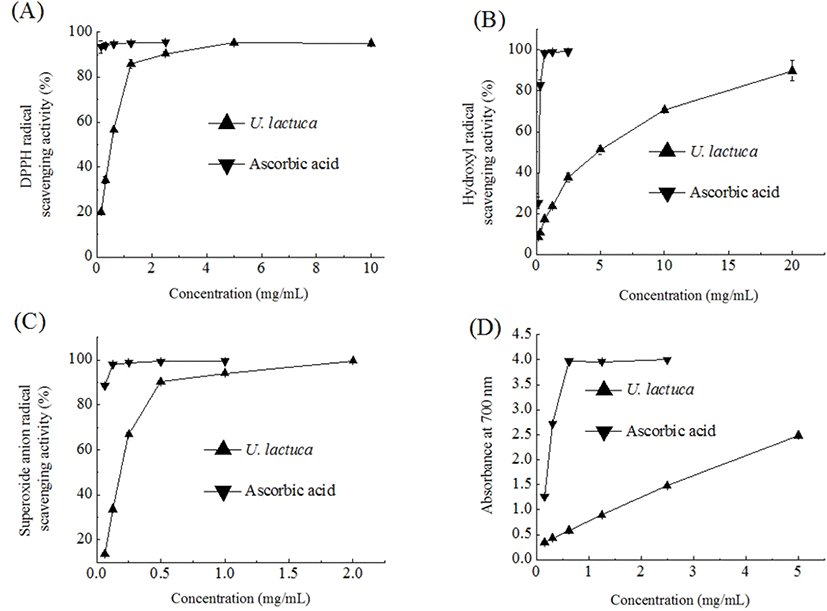
Among the reactive oxygen species, the hydroxyl radical is known to have the strongest chemical activity and cause damage to nearly all types of biomolecules including proteins, DNA, and nucleic acids (Aruoma, 1998). In the present study, we found that the U. lactuca extract scavenged hydroxyl radicals in a concentration-dependent manner (Fig. 1B). The EC50 value of the U. lactuca extract was 4.63 mg/mL. The hydroxyl radical scavenging activity of the U. lactuca extract was comparable to that of an aqueous extract of Capsosiphon fulvescens, a filamentous green seaweed traditionally consumed in the southwestern regions of South Korea. Tong et al. (2014) previously reported that the Capsosiphon fulvescens water extract has a moderate hydroxyl radical scavenging activity; its EC50 value was determined to be 4.85 mg/mL.
Although the superoxide anion radical is not highly reactive, it can produce hydrogen peroxide and hydroxyl radical through dismutation and other types of reaction (Macdonald et al., 2003). In the present study, the trend for the superoxide anion radical scavenging activity of the U. lactuca extract was similar to that for DPPH radical scavenging activity. The superoxide anion radical scavenging capacity of the U. lactuca extract was intermediate (46%) at 0.3 mg/mL and reached a plateau of 91% at a concentration of 0.6 mg/mL (Fig. 1C). The EC50 value of the U. lactuca extract was 0.18 mg/mL. The U. lactuca acetone extract was previously found to have moderate superoxide anion scavenging activity and its EC50 value was 785 μg/mL (Kosanic et al., 2015).
Reducing capacity is a significant indicator of the potential antioxidant activity of a compound or sample. In the reducing power assay, the presence of antioxidants in the extract leads to the reduction of the Fe3+/ferricyanide complex to its ferrous form (Zhang et al., 2012). The reducing power of the U. lactuca extract, in terms of absorbance values at 700 nm, increased with an increase in the extract concentration (Fig. 1D). Further, the EC50 value (the concentration providing 0.5 absorbance) of the reducing power values for U. lactuca was 0.44 mg/mL. Kosanić et al. (2015) also reported that a U. lactuca acetone extract has effective ferric reducing power and that this effect is dose-dependent.
Inflammation is known to occur as a consequence of many different stimuli, such as irritants, physical damage, and cellular trauma. It was established that macrophages act as major mediators of the inflammatory response induced by exogenous pathogens (Li et al., 2012). NO produced in LPSstimulated macrophages is a pro-inflammatory molecule that plays an important part in the inflammatory response. It has been reported that some phenolic-enriched natural extracts exhibit anti-inflammatory activity via decreasing NO levels in RAW 264.7 cells, a widely used macrophage cell line (Lu et al., 2018). Here, we cultured RAW 264.7 cells to evaluate the anti-inflammatory activity of the obtained U. lactuca extract.
The effect of the U. lactuca extract on cell viability was investigated in 3-(4,5-dimethylthiazol-2-yl)-2,5-diphenyltetrazolium bromide (MTT) assays. In the MTT assay, no significant inhibitory effect on cell growth was observed after 24 h of treatment with the U. lactuca extract at a concentration of 25 or 50 μg/mL (data not shown). We then tested whether the extract could alleviate LPS-stimulated NO production in RAW 264.7 cells. U. lactuca extract treatment caused a decline in LPS-induced NO production in RAW 264.7 cells, and this effect appeared to be dose-independent (Fig. 2). This result highlights the biological potential of the U. lactuca extract, which could be explored as a natural anti-inflammatory ingredient. Consistent with our results, previous studies on crude Alaskan U. lactuca extract revealed that the n-hexane, ethyl acetate, 1-butanol, and aqueous fractions of the U. lactuca extract could reduce the mRNA expression of inflammatory biomarkers in RAW 264.7 cells, although the capacity was limited (Kellogg et al., 2015).
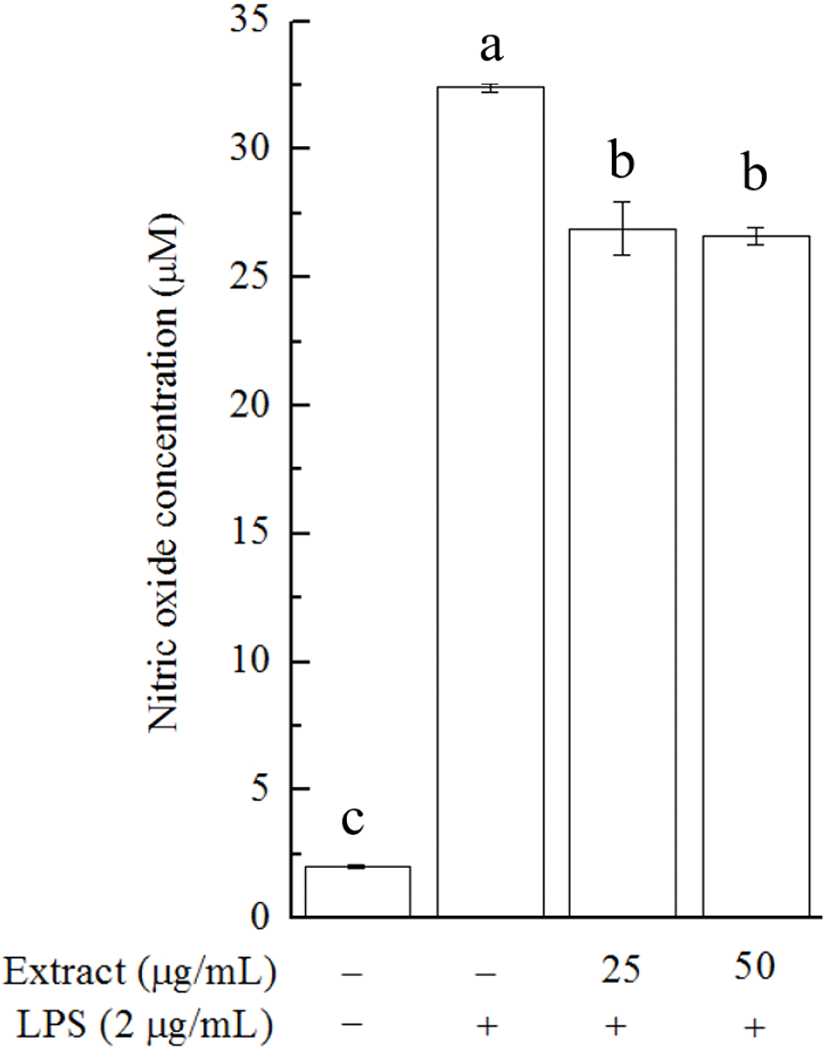
Diabetes mellitus is a common and debilitating condition that results from defects in insulin secretion, insulin action, or both; it is characterized by postprandial hyperglycemia (Jang et al., 2018). One strategy to prevent diabetes is to blunt the absorption of glucose via suppressing the enzymes involved in the digestion of carbohydrates in the digestive system (McDougall et al., 2008). It is known that one particularly interesting feature of the TPC-enriched seaweed extracts is their inhibitory potential against enzymes relevant to hyperglycemia such as α-amylase and α-glucosidase (Tong et al., 2014). In the present study, we found that the U. lactuca extract dose-dependently inhibited α-amylase activity and was able to suppress 50% of α-amylase activity at a concentration of 50 mg/mL (Fig. 3). As expected, acarbose significantly inhibited the α-amylase activity at concentration of 0.1 and 0.2 mg/mL (48.16%±0.42 and 65.64%±0.52 inhibition, respectively). In contrast, the U. lactuca extract showed no obvious inhibitory effect on α-glucosidase at the concentration tested (data not shown). Kellopgg et al. (2014) evaluated the inhibitory effect of U. lactuca collected in Alaska and found that, at 4 mg/mL, the 80% aqueous methanol crude extract had no significant inhibitory potential against both α-glucosidase and α-amylase. These differences in the inhibitory activity of the U. lactuca extract against α-amylase appear to be related to the variations in extraction processes.
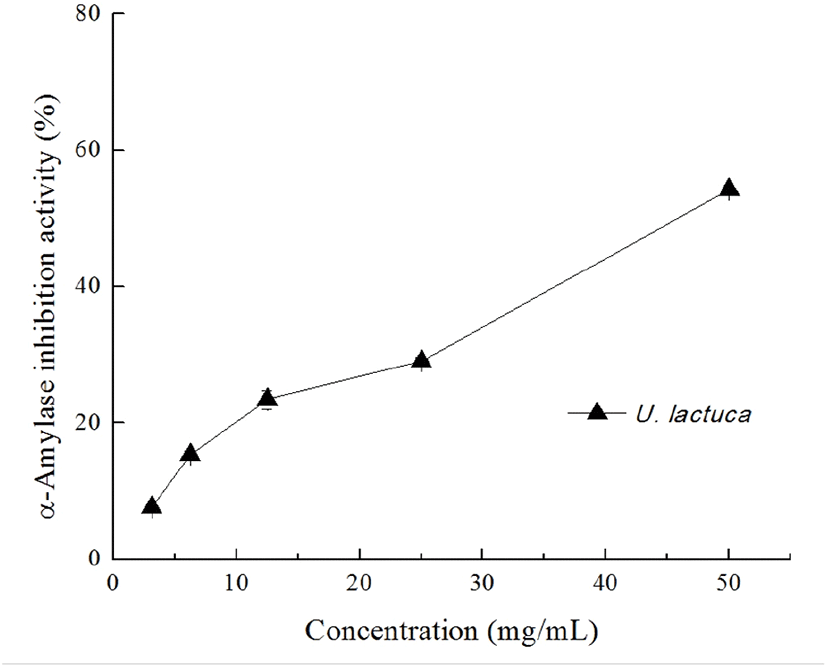
To conclude, this in vitro biological study suggested that the U. lactuca extract has satisfactory antioxidant activity and moderate anti-inflammatory effects as well as inhibitory potential against α-amylase. We speculate that the U. lactuca extract could be investigated as a potential nutritional and pharmaceutical ingredient with special functional activities.