Introduction
Red chicory (Cichorium intybus L.) has received attention for containing phytochemicals, such as phenolic acids, flavonoids, and anthocyanins, which are beneficial to health (Bais and Ravishankar, 2001). The high anthocyanin content in red chicory, making red chicory leaves red, accounted for 50-55% of the total phenolic compounds and show high antioxidant activity (Francesca et al., 2020).
In vivo, polyphenols prevent the production of reactive oxygen species (ROS), which is related to the initiation and progression of many pathological processes (Diplock, 1998; Elstner, 1991). In addition, phenolic compounds in leafy vegetables exert antioxidant activity, protecting against peroxyl radicals generated by lipid peroxidation (Rossetto et al., 2005).
Alzheimer’s disease (AD) is a typical brain disease. Worldwide, the number of dementia patients in 2015 was 50 million, and this number is expected to more than triple by 2050 (Alzheimer’s Association, 2016). AD may shorten the life expectancy of elderly people and cause serious public problems and economic burden (Alzheimer’s Association, 2016).
Reactive oxygen species (ROS), which are generated during diverse metabolic activity in the body, also contribute to the pathophysiology of brain neurological diseases such as AD (Froelich and Riederer, 1995). In a previously reported study, the cerebrospinal fluid of Alzheimer’s patients showed oxidative damage from the formation of oxidative and nitrate proteins, lipid peroxides, and DNA fragments and a collapse of the antioxidant defense system (Martin et al., 2019). In the collapsed antioxidant defense system, the levels of glutathione (GSH), an antioxidant enzyme in the brain, were decreased (Saharan and Mandal, 2014). Additionally, glutathione S-transferase (GST), which is one of the main antioxidant enzymes maintaining the antioxidant defense system and regulating GSH, leads to cellular detoxification and inhibits the activation of signals in cellular apoptosis (Raza, 2011).
Scopolamine increases the activity of acetylcholinesterase, which breaks down acetylcholine in the cholinergic nervous system, decreasing memory ability for a short time (Bartus, 2000). In this regard, scopolamine has been widely used to examine the effect of memory improvement in short-term memory loss-induced animal studies (Ebert and Kirch, 1998). In the scopolamine-induced memory impairment model, the increase in free radicals aggravated the generation of lipid peroxidation related to malondialdehyde and damaged the cellular antioxidant enzymes, including catalase, glutathione peroxidase (GPx), and glutathione reductase (GR), and altered superoxide dismutase (SOD) (Tang, 2019).
Therefore, in this study, we investigated whether red chicory extracted with methanol or ethyl acetate possessed antioxidant activity and whether the antioxidant effect of red chicory extract could improve cognitive dysfunction and behavioral deficits in scopolamine-induced memory-impaired mice.
Materials and methods
Red chicory (593 g) was obtained from S&D Inc. (Yeon-gi, Korea). Dried red chicory (52 g) was extracted at room temperature in a sonicator (Power Sonic 420, Hwashin Co., Seoul, Korea) for one day with 80% methanol (1 L). The mixture was filtered through No.3 filter paper (Whatman International Limited, Kent, UK) three times and concentrated to dryness (Evaporator, MG-2100, Buchi, Switzerland). The 80% methanol extract (40 g) was dissolved in distilled water. The water and fractionate solution was fractionated in a separatory funnel with an equivalent amount of ethyl acetate and the extracted of ethyl acetate was separated and evaporated. The ethyl acetate extraction (yield 3 g) was used in the present study.
Six-week-old male ICR mice were purchased from Raon Bio Company (Daejeon, Korea). The mice were raised at constant temperature (24.0±2.0°C) and humidity (55±10%) with a 12 h:12 h light/dark cycle in an animal house with free access to chow (Purina, Cheongju, Korea) and water. The mice were weighed once a week. The animal experiments were conducted in compliance with the Guide for the Care and Use of Laboratory Animals of the National Institutes of Health (Registration No: CNU-00549).
After a one-week adjustment period, the mice were randomly divided into five groups (6 mice/group) and treated. The procedures are shown in Table 1. Briefly, 2 mg per kg of scopolamine (scopolamine hydrobromide, Sigma Chemical Co., St. Louis, MO, USA) dissolved in saline was injected intravenously (i.v) daily for four weeks. Red chicory or tacrine (9-amino-1,2,3,4-tetrahydroacridine hydrochloride hydrate, Sigma Chemical Co.) dissolved in saline was given by oral gavage daily 30 min after scopolamine injection for three weeks. Tacrine is used as an inhibitor of scopolamine (Baek et al., 2020). In the 4th week, red chicory or tacrine was given by oral gavage 1 h before the water maze task and the passive avoidance test. Then, scopolamine was injected 30 min before the water maze task and the passive avoidance test.
This experiment were selected according to our preliminary experiment (Baek and Kim, 2020). Mouse hippocampus derived cell, HT-22 cells, were maintained in Dulbecco’s modified Eagle’s medium (DMEM) with 10% fetal bovine serum (FBS), 100 units/mL penicillin and 100 μg/mL streptomycin at 37°C with 5% CO2.
Cell viability was assessed using the EZ-Cytox Cell viability Kit (Daeil Lab, Seoul, Korea) according to the manufacturer’s instructions. HT-22 cells were plated into 96-well plates at a density of 3×103 cells/well for 24 h, with each well containing 100 μL of medium. The cells were treated with 5 mM glutamate. After 11 h of incubation, 10 μL of the EZ-Cytox solution was added to each well, and the plates were incubated at 37°C for 2 h. The absorbance was read with an Emax Precision Microplate Reader (Molecular Devices, Sunnyvale, CA, USA) at 450 nm. The percentage of surviving cells was determined relative to the control group.
The level of intracellular reactive oxygen species (ROS) was measured using 2’,7’-dichlorofluorescein diacetate (DCFDA) (Invitrogen, CA, USA) as described previously. Twelve hours after glutamate treatment, the cells were stained with 10 μM DCFDA in Hanks’ balanced salt solution (HBSS) for 30 min in the dark, and the fluorescence was monitored using a microplate reader (Beckman Coulter DTX 880 Multimode Detector, Brea, CA, USA) at an excitation wavelength of 485 nm and an emission wavelength of 525 nm. Finally, the measured value were calculated and marked as % of control group.
Radical scavenging ability was determined by adding 300 μL of 1.5×10−4 mM DPPH solution to 900 μL of sample with different concentrations of red chicory (10, 5, 2.5, 1.25, 0.625, 0.3125, 0.125, and 0.0625 mg/mL). The reacted solution were placed in the dark at room temperature and after 30 min, the OD value was measured at 515 nm. The blank contained 300 μL of methanol and 900 μL of DPPH solution. Vitamin C was used as a positive control. DPPH radical scavenging activity was determined by the following equation:
AC: 900 μL 1.5×10−4 mM DPPH + 300 μL MeOH.
AS: 900 μL 1.5×10−4 mM DPPH + 300 μL sample in different concentrations
The Morris water maze (MWM) test was conducted for seven consecutive days before the end of the experiment. RCE or tacrine was given by oral gavage 1 h before, then scopolamine at 2 mg/kg body weight was injected 30 min before the water maze task.
The MWM test for mice consisted of a circular pool with an escape platform with a diameter of 10 cm installed in a water tank with a width of 60 cm and a depth of 150 cm. The water tank was divided into quartiles and the escape platform was located in one quartile. The mice were placed anywhere in the remaining quartiles. On the first day of the test, no escape platform was set up and the mice swam freely for two min, then cognitive adaptation training was conducted using the escape platform in different quadrants every trial day. When the animal reached the escape platform, it was allowed to stay on the escape platform for 10 sec, and if the escape platform was not found within 120 sec, the mouse was placed on the escape platform for 10 sec to remember the escape platform. On the fifth day of the experiment, the escape platform was removed to measure the working memory, and the latency time for visiting the escape platform was recorded.
Nocturnal animals have a habit of going from a bright place to a dark place. Utilizing this behavior, when a mouse entered a dark room, it was given an electric shock. Finally, the animal equated an electric shock with a dark room through training. Using a partition, the animals were placed in a box divided into two compartments and an electric shock of 0.5 mA was applied to the stainless grid floor for 5 sec immediately after the animals moved to the dark compartment. The time from the entrance to the illuminated compartment was measured. To confirm the effect of the sample on the long-term memory of the animal, we measured the time it took to enter the dark compartment when placed again in the bright area after 24 h. After each experiment was completed, the box was wiped clean with 70% alcohol to erase the traces of the immediately preceding experimental animal and not affect the following experiments.
The animals were euthanized, brain tissue was homogenized with a tissue homogenizer (Hoverlabs, Haryana, India) in a buffer solution (12.5 mM sodium phosphate buffer, pH 7.0, and 400 mM NaCl), centrifuged at 1,000 rpm at 4°C for 10 min, and the supernatant was collected. The supernatant was stored at −70°C until use.
The levels of total GSH were determined by mixing with 0.1 M potassium phosphate buffer, 10 mM DTNB, and 5 mM NADPH, equilibrated for 1 min by adding 1 unit of GR, and the absorbance was measured at 412 nm using a spectrophotometer. GSH (0-0.04 mM) was used for the standard curve.
GST activity was measured as described previously using 1-chloro 2,4-dinitrobenzene as the substrate (Habig et al., 1974). The standard assay mixture contained 1 mM 1-chloro 2,4-dinitrobenzene (CDNB), 1 mM reduced GSH, and 100 mM potassium phosphate buffer (pH 6.5) in a volume of 1 mL. The reaction was followed at 340 nm.
GPx activity was measured by reacting with 0.1 M sodium phosphate butter, 5 mM NADPH, 100 mM GSH, and 1 unit of GR. Three minutes later, 100 mM cumene-OOH was added. Absorbance was measured at 340 nm with a spectrophotometer.
GR activity was measured in a reaction mixture containing 0.1 M phosphate buffer, 1 M GSSG, and 5 mM NADPH, and the absorbance was measured with a spectrophotometer at 340 nm.
All results are expressed as means±SEM. Statistical analyses were performed using IBM SPSS 24 software (Statistical Package for Social Science. SPSS Inc., Chicago IL, USA). Differences between the groups were compared by one-way ANOVA followed by the least significant difference (LSD) test to further analyze the differences between the two groups. A p-value of <0.05 was used to indicate statistical significance.
Results and discussion
HT-22 cells were exposed to glutamate (5 mM) for 12 h with or without RCE. As shown in Fig. 1, cell viability was remarkedly reduced by about 50% (p<0.001) in the glutamate-only treated group compared to the control group. Treatment with RCE dose-dependently increased cell viability. In the group treated with RCE 100 μg/mL, cell viability was the same as the control group. These results showed that RCE exerted a neuroprotective effect against glutamate-induced oxidative stress in HT-22 cells. An extract possessing a high antioxidant effect showed cell protective activity against cell death in HT-22 cells by reducing glutamate-induced oxidative stress (Baek and Kim, 2020).
To confirm the effect of RCE on the level of ROS, a marker of oxidative stress, The HT-22 cells were exposed to glutamate, and measured by adding DCFDA reagents. When DCFDA of non-fluorescent DCF, which represents ROS generation. As shown in Fig. 2, treatment with 5 mM glutamate significantly increased ROS production 3-fold compared to the untreated control group. ROS generation in the RCE -treated group was reduced in a dose-dependent manner. Also, ROS production by treatment with 100 to 250 μg/mL of RCE was effectively reduced to the level of the control group (p<0.001).
Studies have shown that abundant polyphenols can prevent and treat neurodegenerative diseases. Mulberry fruit extract (Shin et al., 2019) showed a nerve cell protective effect against oxidative stress in HT-22 cells.
Substances that react with ROS are considered antioxidants, so are considered radical scavengers (Seyed et al., 2012). DPPH scavenging analysis was used to demonstrate the antioxidant capacity of RCE, and the results are shown in Fig. 3. The IC50 value, which is the concentration at which the radical scavenging activity is 50%, was calculated and obtained (Blois, 1958). The IC50 value of RCE was 37.9 mg/mL compared to an IC50 in the vitamin C positive control of 0.03 mg/mL.
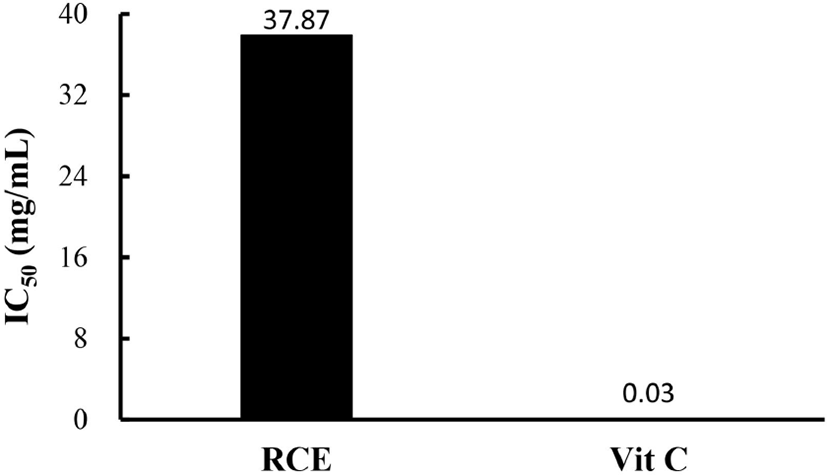
Mice in the scopolamine-treated group showed memory impairment, with a prolonged latency time during seven days of training. Scopolamine-treatment did not decrease the escape latency time to locate the platform in the water maze test. Many dementia model experiments that caused memory damage with scopolamine confirmed that long-term memory damage was induced normally (Rispoli et al., 2004; Lu et al., 2007). Mice treated with scopolamine and RCE showed significantly decreased latency time (Fig. 4A). As shown in Fig. 4B, mice treated with RCE showed significant memory enhancement with decreased escape latency time compared to the scopolamine-alone group. The escape latency time was decreased dose-dependently by treatment with RCE. In addition, the platform crossing numbers in the SRCE 300 group were significantly increased than those treated with tacrine as well as the scopolamine group (Fig. 4C). Through animal behavior experiments, we confirmed the effect of RCE on memory impairment.
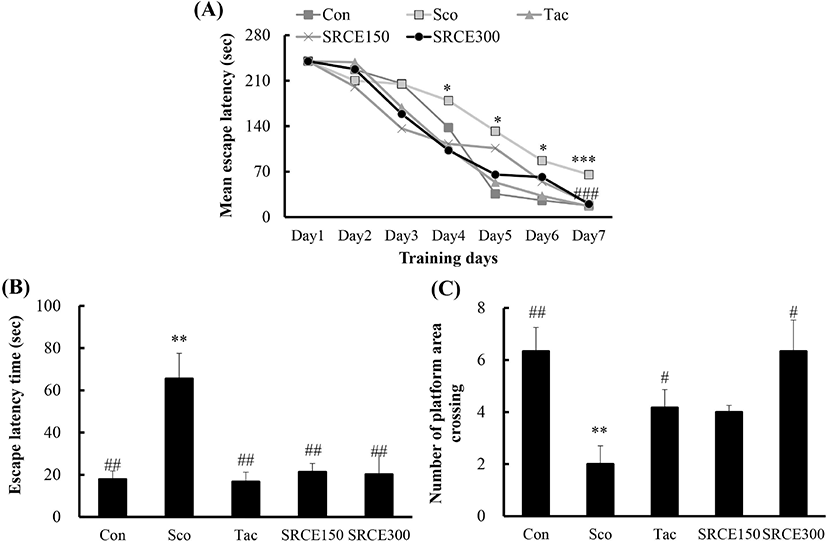
The passive avoidance task was also carried out to determine the effect of RCE on scopolamine-induced memory impairment. As shown in Fig. 5, the scopolamine-treated group showed a significant decrease in latency time compared to the negative control group. Both the tacrine-treated and SRCE 150 groups showed significant memory enhancement by longer latency time compared to the scopolamine-only treated group. Therefore, this result suggests that RCE had a protective effect on scopolamine-induced cognitive impairment.
Glutamate is an excitatory neurotransmitter that regulates the growth and migration of neurons, which play a critical role in learning and memory formation. High concentrations of glutamate reduce the amount of glutathione in the cell by inhibiting cysteine in the cell membrane through the cystine/glutamate transport system (Fukui et al, 2009). In addition, it increases the production of calcium ions (Ca2+) and ROS in cells, causing oxidative stress.
This study measured GSH, GST, GPx, and GR activity to evaluate the effects of RCE. As shown in Fig. 6A, decreased GSH levels were seen in the scopolamine-only treated group, and GSH in the SRCE 150 group was increased to levels in the control group. In addition, the level in the SRCE 300 group was significantly increased over that in the control group. Also, the level of GST in both the SRCE 150 and SRCE 300 groups showed increased activity compared to tacrine group (Fig. 6B).
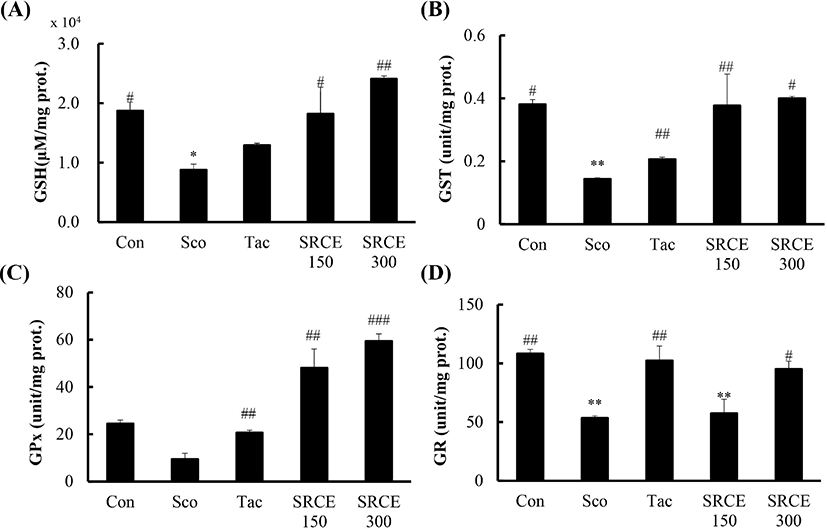
RCE treatment showed the largest difference in GPx levels compared to the other antioxidant enzymes. The results showed that both the SRCE 150 and SRCE 300 groups had activity twice as high as that of the controls (Fig. 6C).
However, the GR results were slightly different than those of the previous indicators. The SRCE 300 group was significantly different from the scopolamine group but showed lower activity than the control and tacrine groups (Fig. 6D). RCE treatment showed the largest difference in GPx levels compared to the other antioxidant enzymes. The results showed that both the SRCE 150 and SRCE 300 groups had activity twice that of the controls (Fig. 6C).