Introduction
‘Fuji’ is an apple cultivar that has been increasingly cultivated worldwide (Mannucci et al., 2017). During development, many young fruits are removed from the trees in a process referred to as thinning, which helps to enhance the size and quality of the mature fruits (Chun et al., 2012). Only a small portion of thinned young apples are used for the production of functional compounds, such as polyphenols, and processed foods, with the vast majority being discarded (Zheng et al., 2012). Unlike mature apples, young apples have a high starch content and can therefore function as valuable starch sources (Song et al., 2003). Unfortunately, little is known about the starch properties of thinned young ‘Fuji’ apples, limiting their general and industrial applications.
Starch is a polysaccharide composed of glucose monomers connected by glycosidic bonds and is the primary carbohydrate stored in plants (Singh et al., 2003). The granular form of starch is typically composed of two types of molecules: linear amylose and branched amylopectin (Sarka and Dvoracek, 2017). Depending on its physicochemical characteristics, starch is industrially used as a raw material for malting and fermentation, and for the production of thickeners, colloidal stabilizers, gelling agents, bulking agents, water retention agents, and adhesives (Singh et al., 2003). Starches from different botanical sources and different cultivars of the same crops have been reported to possess distinct physicochemical characteristics (de Barros Mesquita et al., 2016; Singh et al., 2003). Therefore, it is crucial to identify the unique properties of the starches from different sources before their application. Starches from corn and potatoes have been characterized and used commercially (Singh et al., 2003). Unlike these starches, the physicochemical properties of apple starch, particularly that from ‘Fuji’ apples, have not been well characterized and no practical use has been found for them. Therefore, it is necessary to investigate the properties of apple starch and to compare them with the properties of well-known potato and corn starches.
It was found that there are significant differences in the structure, amylose content, and pasting properties of apple starches from different cultivars, such as ‘Gala’, ‘Golden Delicious’, ‘Granny Smith’, ‘Jersey Mac’, ‘Jonagold’, and ‘Royal Gala’ (Stevenson et al., 2006). Previous studies reported that the ‘Fuji’ apple starch content is the highest at 70 days after full bloom (Song, 2003) and rapidly decreases as the fruit matures (Fan et al., 1995). The starch contents of ‘Golden Delicious’ and ‘Cox's Orange Pippin’ apples decreased almost to zero during storage (Gorin et al., 1978). The morphological properties of starch from the ‘Jonagold’ (Ohmiya and Kakiuchi, 1990) and ‘Mutsu’ (Kovács and Eads, 1999) apple cultivars have also been reported. However, the phosphorous content, swelling power, solubility, digestibility, and syneresis of apple starch have not yet been described. The above reports indicate that the apple starch properties are dependent on the cultivar; therefore, we expect that ‘Fuji’ apple starch will differ from other apple starches and corn and potato starches.
The objective of this study was to investigate the chemical, morphological, pasting, digestive, and syneresis properties of the starch isolated from thinned young ‘Fuji’ apples, and compare them to those of potato and corn starches. The results of this study are expected to provide a better understanding of the starch obtained from thinned young apples for their effective utilization.
Materials and methods
Young ‘Fuji’ apples (Malus domestica Borkh.) thinned 60 days after full bloom were collected from a commercial farm in the Miryang region of Gyeongsangnam-do, South Korea, immediately transported to the laboratory and subjected to starch isolation. The average weight of the fruit was 25.81±8.05 g. Corn and potato starches were purchased from Samchun Pure Chemical Co. (Pyeongtaek, South Korea). Amylose from potato (A0512), amylopectin from maize (10120), pancreatin from porcine pancreas (P7545), amyloglucosidase from Aspergillus niger (A7095), and a glucose assay kit (GAGO20) were purchased from Sigma- Aldrich (St. Louis MO, USA). All other chemicals used for the analyses were obtained from commercial sources and were of high purity grade.
Whole apples were crushed and filtered through non- woven fabrics. The filtrate was centrifuged at 3,000 rpm for 10 min to precipitate the starch. The precipitate was collected, dispersed in distilled water, and passed through a 0.147 mm sieve. The purification process involving the discarding of supernatant and dispersing the precipitate in distilled water was repeated several times. The purified starch was then dried in an oven at 35°C for 24 h, ground, and passed through a 0.147 mm sieve.
The apparent amylose content of starch was determined according to the procedure described by Williams et al. (1970). Briefly, the starch sample (20 mg) was placed in a beaker and 0.5 N KOH (10 mL) was added and dispersed for 10 min. The mixture was diluted to a final volume of 100 mL using distilled water, and 20 mL of the diluted solution was collected and neutralized with 0.1 N HCl (10 mL). Then, the neutralized sample was treated with an iodine solution (1 mL, 0.2% I2+2% KI) and diluted to 100 mL using distilled water. After reacting for 20 min, the absorbance of the solution was measured at 680 nm using a spectrophotometer (UV-1800, Shimadzu, Kyoto, Japan). A standard curve was prepared using amylose and amylopectin standards mixed at known ratios.
The phosphorous content of starch was determined using the method described by Noda et al. (2004). Briefly, the starch sample (0.2 g) was digested using HNO3. The phosphorus content in the digestion was measured as inorganic phosphorus, using the vanado-molybdate method For the assay, 2 mL of the starch digestion solution, 3 mL of distilled water, 0.5 mL of 60% perchloric acid, 1.5 mL of 20 mM ammonium vanadate solution containing 2.4% perchloric acid, and 3.0 mL of 3.53% ammonium molybdate solution were mixed together and kept for 30 min. Finally, the absorbance was measured at 420 nm using a spectrophotometer (UV-1800, Shimadzu).
Starch morphology was examined using scanning electron microscopy (SEM, S-3500N, Hitachi Co., Tokyo, Japan). Briefly, the starch sample was spread onto a carbon tape attached to the top of the stub and coated with gold under vacuum using a SEM ion sputter coater. The coated starch was visualized between 500 and 5,000× magnification at 15 kV. The starch particle size was measured using a laser diffraction particle size analyzer (LS13 320, Beckman Coulter Inc., Fullerton, CA, USA). For this, starch (0.5 g) was dispersed in isopropyl alcohol (10 mL), and the average particle diameter was measured in the range of 0.04 to 2,000 μm.
The X-ray diffraction pattern of the starch was obtained using an X-ray diffractometer (Empyrean Series 2, Malvern Panalytical, Almelo, Netherlands) with the following specifications: Cu Kα, voltage=40 kV, current=30 mA, filter =Ni, scanning speed=0.017°/min, and diffraction angle=4-40°.
The swelling power and solubility of the starch were measured at 40, 50, 60, 70, 80, and 90°C as proposed by Schoch (1964). Suspensions of starch (0.5 g) in distilled water (30 mL) were incubated at the specified temperature in a water bath for 30 min with shaking at 150 rpm. The samples were then cooled to room temperature, centrifuged (4°C, 6,000 rpm, 30 min), and the supernatant and sediment were isolated for further analysis. The weight of the sediment was measured immediately. The supernatant was dried at 105°C to obtain the weight of soluble starch. The swelling power and solubility were calculated using the following equation:
The starch pasting properties were determined using a Rapid Visco-Analyzer (RVA-4, Newport Scientific, Warriewood, NSW, Australia). Starch suspensions were prepared for analysis by adding distilled water (25 mL) to starch (3 g) and stirring at 960 rpm. The suspensions were transferred to an RVA set at 50°C and stirred for 1 min at 160 rpm using a plastic paddle. The heating program was as follows: heated to 95°C at a rate of 12°C/min, maintained at 95°C for 2.5 min, cooled to 50°C at a rate of 12°C/min, and maintained at 50°C for 1 min.
The In vitro digestibility of starch was measured using the method described by Englyst et al. (1992). Digestive enzymes were produced by mixing a pancreatin solution (2.7 mL) and amyloglucosidase (0.4 mL). Briefly, pancreatin from the porcine pancreas (0.45 g) was dispersed in distilled water (4 mL) for 10 min, centrifuged (4°C, 10,000 rpm, 10 min), and the supernatant was collected. An amyloglucosidase solution was prepared by mixing amyloglucosidase from A. niger (0.32 mL) with distilled water (0.4 mL). For the analysis, starch (100 mg), sodium acetate buffer (4 mL, pH 5.2), digestive enzymes (1 mL), and 10 glass beads (4 mm in diameter) were stirred for specific times (0, 20, 60, 120, and 180 min) in a shaking water bath at 37°C and 170 rpm. The digested starch solution (0.1 mL) was mixed with an 80% ethanol solution to stop the reaction, centrifuged (4°C, 3,000 rpm, 10 min), and used for the analysis. The glucose content of the hydrolyzed starch sample solution was measured using a glucose assay kit. The rapidly digestible starch (RDS) content was calculated from the quantity of glucose generated within 20 min, and the slowly digestible starch (SDS) content was calculated from the quantity of glucose generated between 20 and 120 min. The resistant starch (RS) was calculated by subtracting the RDS and the SDS content from 100.
The syneresis of starch was measured by repeating the freeze-thaw cycle of the starch gel. Briefly, starch gels were prepared by dispersing a 10% starch suspension at 25°C for 10 min, heating in a shaking water bath (95°C, 150 rpm, 30 min), followed by cooling at 4°C for 1 h. The starch gels were frozen for 20 h at -20°C and then thawed for 3 h at 30°C. This constituted one freeze-thaw cycle, after which the sample was centrifuged (4°C, 3,000 rpm, 1 h). Subsequently, the water that had separated from the gel was removed, and the gel was weighed to calculate the syneresis by using the following equation:
After the measurement, the gels were subjected to up to five freeze-thaw cycles, and the cumulative syneresis was obtained.
The results were calculated using IBM SPSS statistics (23.0, IBM Corp., Armonk, NY, USA) to determine the means and standard deviations. The significance values between samples were analyzed using one-way analysis of variance and Duncan’s multiple range tests at a significance level of p<0.05.
Results and discussion
The amylose content plays a central role in the physicochemical parameters of starch (de Barros Mesquita et al., 2016). It can vary between botanical sources and within the same botanical variety owing to differences in the geographical origin and culture conditions (Alcazar-Alay and Meireles, 2015). The apparent amylose contents of the ‘Fuji’ apple, corn, and potato starches are listed in Table 1. The apparent amylose content of apple starch (33.6%) was significantly higher than that of corn starch (29.8%) (p<0.05); however, there was no significant difference between that of apple starch and that of potato starch (33.6-33.8%) (p>0.05). The apparent amylose content of ‘Fuji’ apples is lower than the reported apparent amylose content of the starches isolated from other apple varieties, including ‘Gala’, ‘Golden Delicious’, ‘Granny Smith’, ‘Jersey Mac’, ‘Jonagold’, and ‘Royal Gala’ apple (39.8% to 48.1%) (Stevenson et al., 2006), which may be due to differences in apple varieties, fruit maturity, and the measurement techniques used (Duan et al., 2012; Fan et al., 1995; Stevenson et al., 2006).
Phosphorus is one of the minerals present in starch granules and significantly affects its functional characteristics (Alcazar-Alay and Meireles, 2015). The phosphorus contents of the apple, corn, and potato starches are listed in Table 1. The phosphorous content of the apple starch was 13.0 mg/100 g, which was significantly lower than that of potato starch (49.2 mg/100 g) (p<0.05), but higher than that of corn starch (11.3 mg/100 g). It has been reported that potato starch has a higher concentration of phosphorous than starches from other botanical sources, and the phosphorus content has been found to have a significantly positive correlation with starch viscosity (Noda et al., 2004). The phosphorous content of other apple varieties has not yet been reported.
‘Fuji’ apple starch granules were spherical, hemispherical, and broken spherical in shape (Fig. 1), resembling the starches obtained from ‘Gala’, ‘Golden Delicious’, ‘Granny Smith’, ‘Jersey Mac’, ‘Jonagold’, and ‘Royal Gala’ apples (Stevenson et al., 2006). These shapes were distinct from those of the starch granules of corn (angled polyhedron with grooves) and potato (smooth and round) (Fig. 1). Interestingly, the irregular shape of the starch granules from ‘Mutsu’ apples was found to shift to a gradually smaller and rounder shape as they were degraded during maturity and increased storage periods (Kovacs and Eads, 1999).
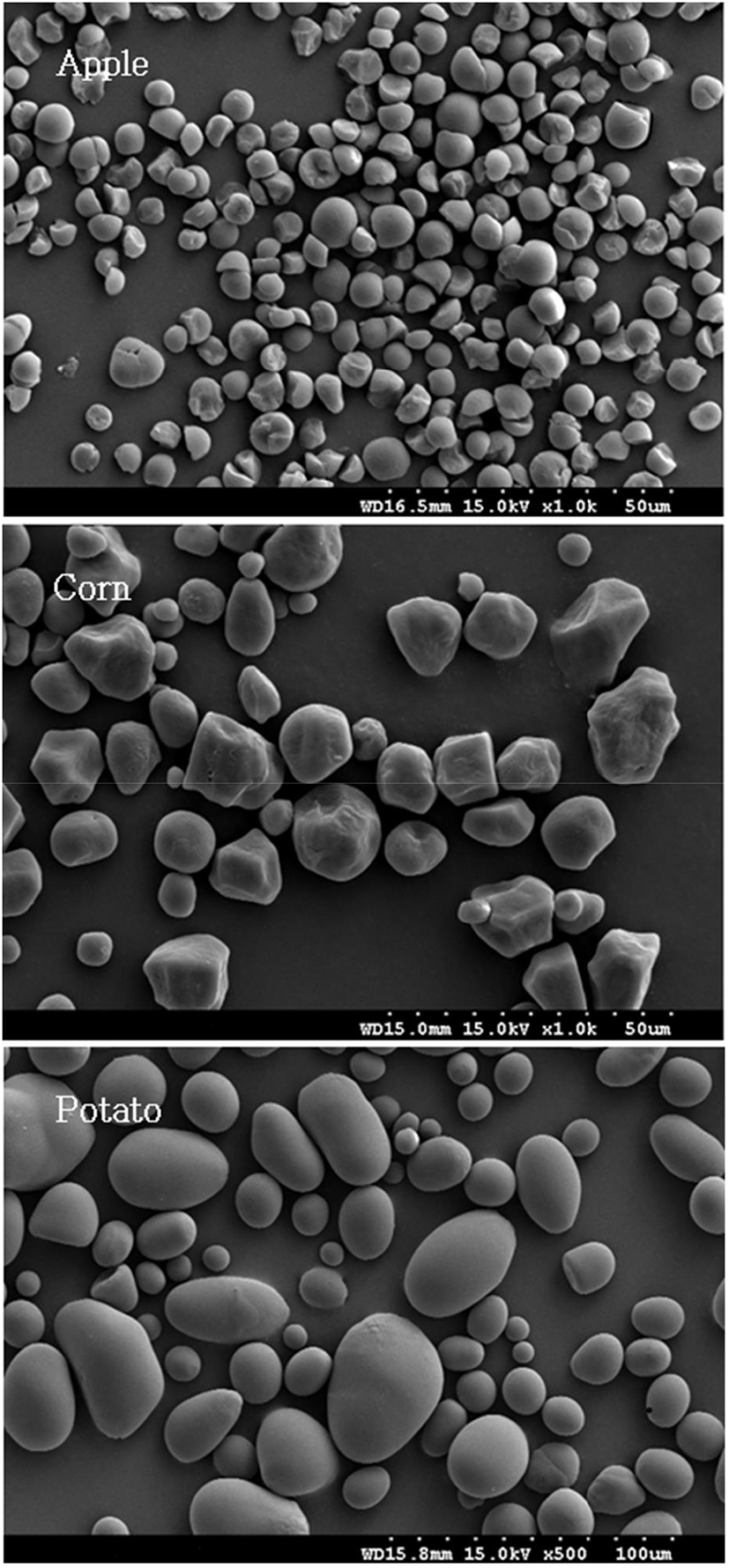
Similar to the chemical components, the starch granule size influences its functional properties (Singh et al., 2003). The granule size of the apple, corn, and potato starches are listed in Table 1. The mean starch granule size was the smallest for the ‘Fuji’ apple starch (7.5 μm) and the largest for the potato starch (35.2 μm). The mean granule size of ‘Fuji’ apple starch was approximately midway between those of ‘Gala’, ‘Golden Delicious’, ‘Granny Smith’, ‘Jersey Mac’, ‘Jonagold’, and ‘Royal Gala’ apple starches (2 μm to 12 μm) (Stevenson et al., 2006). The granule size of unripe and ripe ‘Mutsu’ apple starches was reported to be 15-40 μm, indicating that the area and perimeter of the apple starch granules decreases with an increasing storage period or maturity (Kovacs and Eads, 1999). In addition to apple variety, maturity, and storage period, differences in granule size may be observed due to the use of different methods for measuring the size of the particles, which include laser diffraction particle size analysis, SEM (Stevenson et al., 2006), and image analyses (Kovacs and Eads, 1999).
X-ray diffractometry was used to investigate the presence and characteristics of the crystalline structure of the starch granules (Singh et al., 2003). The X-ray diffraction pattern of ‘Fuji’ apple starch shows characteristic peaks of both A-type (2θ=15.36° and 23.11°) and B-type (2θ=5° and 17.28°) starch. This A/B-type mixture, a C-type X-ray diffraction pattern, observed for 'Fuji' apple starch has also been observed for starch obtained from ‘Gala’, ‘Golden Delicious’, ‘Granny Smith’, ‘Jersey Mac’, ‘Jonagold’, and ‘Royal Gala’ apples (Stevenson et al., 2006). Corn starch showed a typical A-type X-ray diffraction pattern, with strong peaks at 15.37 and 23.09°, while potato starch showed a typical B-type X-ray diffraction pattern, with weak peaks at 5.6 and 22-23°, and a strong peak at 17° (Fig. 2). It is well known that cereal starches show a typical A-type X-ray diffraction pattern, whereas tuber starches show a B-type X-ray pattern (Singh et al., 2003).
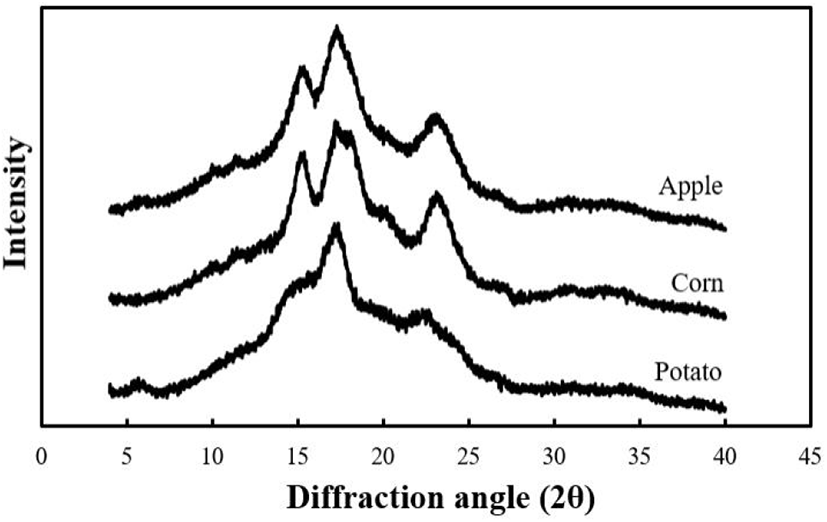
The swelling power and solubility of starch are temperature-dependent properties that increase with an increasing temperature because of the weakening of the internal associative forces maintaining its granular structure (Kong et al., 2015). The swelling powers of potato, apple, and corn starches were relatively steady between 40°C and 50°C but were increased at 60°C. The swelling powers increased in the order of potato > apple > corn starch (Fig. 3). This increase in swelling power results from the decrystallization of the starch granule crystalline region and is closely linked to the gelatinization temperature. As the temperature increases, the binding forces decrease, enhancing the mobility of starch granules (Jyothi et al., 2010; Leach et al., 1959). Tester and Morrison (1990) reported that the starch swelling powers increase with increasing amylopectin content and the size of the amorphous region of starch. Potato starch is reported to have high swelling power, which is likely associated with the high content of phosphate groups on its amylopectin (Singh et al., 2003). The results of this experiment were similar to the phosphorus content results (Table 1). The observed increase in swelling power resulted from the decrystallization of the starch granule crystalline structures, which led to an increased mobility of the starch granules due to the reduced binding forces associated with the rising temperatures, which was related to their gelatinization temperatures (Jyothi et al., 2010; Leach et al., 1959).
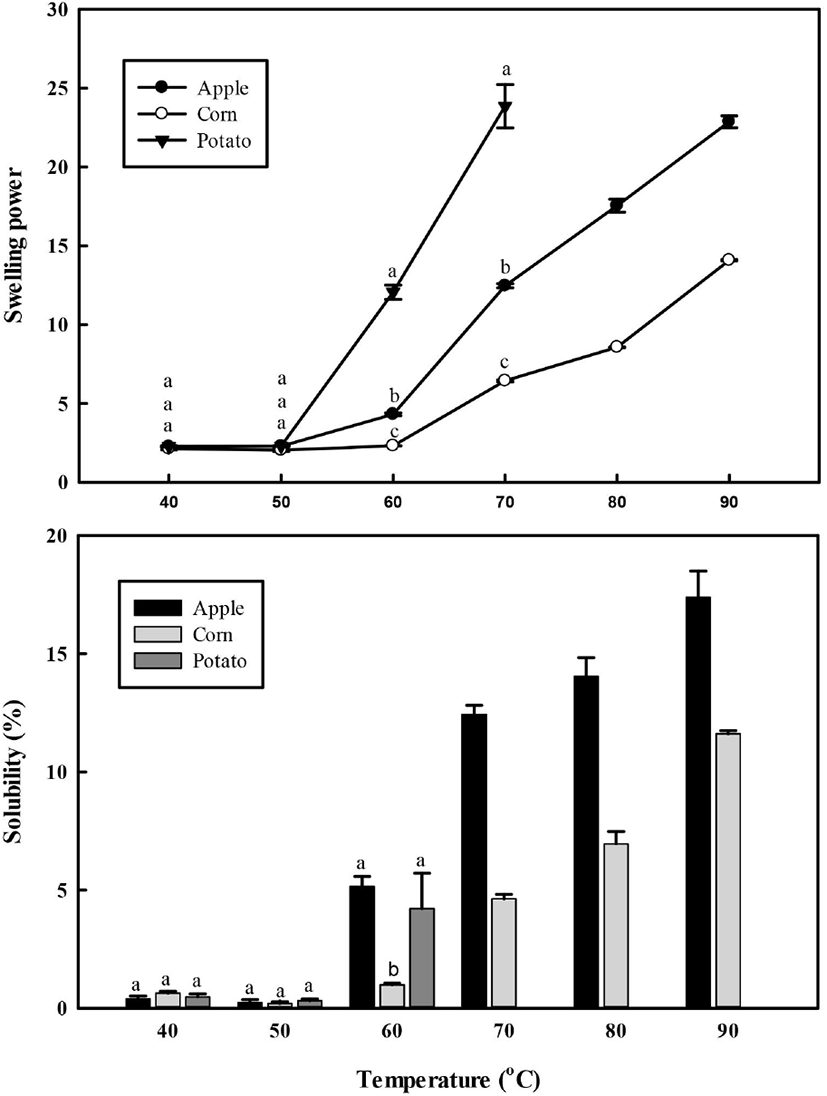
The solubilities of the apple, corn, and potato starches are presented in Fig. 3. Potato starch solubility was unstable at 60°C and could not be measured at more than 70°C because the sediment and supernatant were not separated for measurement. The starch solubility remained constant until 50°C but increased at 60°C, similar to the result obtained for the swelling power (Fig. 3). Increases in solubility with increasing temperature were observed to be faster in apple starch than in corn starch. This may be due to differences in the amylose content of these starches (Table 1). Higher amylose content in starch granules has been reported to increase the solubilization capacity in an aqueous solution due to its leaching at high temperatures (de Barros Mesquita et al., 2016).
The pasting properties of starch play an essential role in selecting a starch source for industrial use. These were investigated and are listed in Table 2. The peak viscosity and breakdown of the ‘Fuji’ apple starch were 450.5 RVU and 133.8 RVU, respectively which was higher than those of corn starch (255.1 RVU and 90.1 RVU) and lower than those of potato starch (815.8 RVU and 735.2 RVU). This high level of peak viscosity and breakdown in potato starch is consistent with the findings previously reported by Noda et al. (2004). The difference in peak viscosity and breakdown of apple starch and corn starch might be due to the differences in the phosphorus content (Table 1). Among the three starch types, apple starch had the highest hold and final viscosity values. Additionally, the apple starch was similar to corn starch in terms of breakdown viscosity and setback. Because the setback of apple starch was lower than that of potato starch, the retrogradation of apple starch was expected to be slower than that of potato starch. The peak time was the shortest in potato starch, the longest in corn starch. The pasting temperature of apple starch was 70.2°C, which was lower than that of corn starch (77.1°C), but higher than that of potato starch (65.8°C). The reported pasting temperatures of ‘Gala’ and five other apple cultivar starches ranged from 69.9 to 71.3°C (Stevenson et al., 2006). The above results suggest that the high viscosity of potato starch pastes was maintained for a sort period and that of the apple starch pastes was maintained for extended period. This property is available for the utilization of apple starches. Apart from the pasting temperature, further comparisons between the pasting properties of ‘Fuji’ apple starch and other apple varieties (Stevenson et al., 2006) could not be performed because of the notable differences in the experimental conditions used of these studies.
The In vitro digestibilities of the apple, corn, and potato starches are listed in Table 3. Apple starch had the highest In vitro digestibility among the three starches at 180 min. The RDS content of apple starch was 34.3%, which was significantly higher than those of corn (26.2%) or potato (13.4%) starch (p<0.05). Comparatively, the SDS content of apple starch was the highest among the three samples, resulting in the lowest RS content. The In vitro digestibility of starch derived from other apple varieties has not yet been reported. In general, starch digestibility decreases with an increasing amylose content (Singh et al., 2010). However, the particle size of starch exerts a greater digestibility effect than the amylose content, with smaller particles resulting in increased digestibility. This is likely due to the enhanced binding of the enzyme to smaller particles with an increased surface area (Langworthy and Deuel, 1922; Tester et al., 2006). Therefore, the high digestibility of ‘Fuji’ apple starch may be explained by its small particle size (Table 1). This characteristic can be applied to fields that require a rapid digestion.
The syneresis of the starch gels after freeze-thaw treatment is presented in Fig. 4. The syneresis of all three starches increased with an increasing number of freeze-thaw cycles. The syneresis curves showed a gradual increase as the freeze-thaw cycles were repeated, probably because the starch syneresis was close to its maximum or entered a stage of stagnation due to repeated freezing and thawing (Baker and Rayas-Duarte, 1998). The syneresis of apple starch was the lowest, indicating the highest freeze-thaw stability (Wang et al., 2013). Physical changes due to freeze-thawing of the starch gel are related to moisture retention and retrogradation of starch (Majzoobi et al., 2016). Therefore, it is presumed that retrogradation of apple starch with the lowest syneresis is slow. This result is consistent with the prediction that the apple retrogradation of apple starch is slower than that of potato starch because the setback of apple starch was lower than that of potato starch (Table 2).
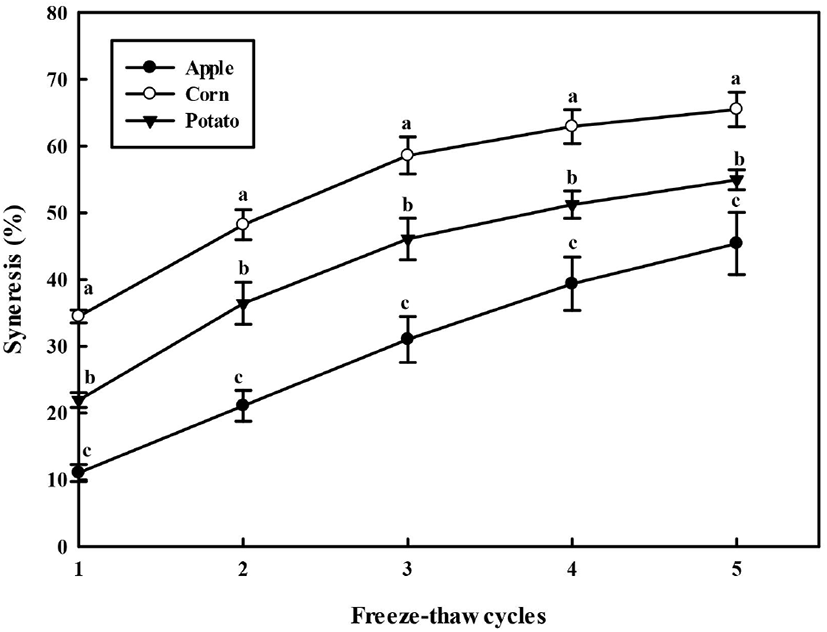
In conclusion, the chemical, morphological, pasting, digestive, and syneresis properties of the starches isolated from thinned young ‘Fuji’ apples, corn, and potatoes were analyzed and compared. The granules of apple starch were spherical, hemispherical, and broken spherical and revealed C-type X-ray diffraction patterns, unlike those of corn (A-type) and potato (B-type) starches. The mean particle size of the apple starch granules was the smallest among all the starches studied. Apple starch had higher hold and final viscosities, a lower syneresis, and a higher In vitro digestibility, than the other two starches. The phosphorus content, swelling power, and pasting temperature values of the apple starch were between those of the potato and corn starches. These results indicate that apple starch forms a paste or gel with long-term high viscosity and digestibility, which leads to a retrogradation delay. These unique properties of young ‘Fuji’ apple starch could support a wide range of uses, although further research is required before its industrial application.