Introduction
Cumin (Cuminum cyminum L.) is an aromatic herbaceous plant member of the family Apiaceae (Umbelliferae) and is an indigenous plant originated from the East Mediterranean, Egypt, and Turkistan regions. Currently, cumin is widely cultivated in India, Pakistan, Uzbekistan, Iran, Turkey, Morocco, Syria, Chile, Mexico, and China (Tuncturk and Tuncturk, 2006). Cumin seeds are generally known as “Zeera,” or “Zira” among the Central Asian countries (Nadeem and Riaz, 2012). In Uzbekistan, cumin is one of the main food ingredients in Uzbekistani cuisines. Cumin seeds are oblong in shape and yellow-gray in color. Since the ancient times, cumin seeds have been widely used in various cuisines, both in whole-seed or in ground forms. Cumin seeds have been used as a traditional food ingredient in countless types of foods, including soups, kormas, and as an ingredient in several types of spice blends for thousands of years. In addition, health benefits such as digestive stimulating, antidiabetic, and anti-inflammatory activities and other health-enhancing effects of cumin have been documented (Srinivasan, 2018). Some studies have suggested that cumin is rich in antioxidants (Bukhari et al., 2009). Cumin seeds extracted using alcohol or water solvents were found to possess many nutraceutical benefits namely, anti-allergic, antioxidant, anti-aggregative, and hypoglycemic effect (Sowbhagya, 2013). Hence, cumin and its products, such as cumin extracts, essential oil, and powder, can be a beneficial resource of nutraceuticals rich in bioactive compounds.
A higher content of bioactive compounds extracted from cumin seed is of great importance in the development of improved processing and extraction methods. Various studies have been conducted on identifying the phenolic compounds as well as the antioxidant activity and antimicrobial effects of cumin seed extract and essential oil. Agrawal et al. (2016) evaluated different seed and leaf extracts of cumin and found a high total phenolic and flavonoid content that is associated with their antioxidant properties. In addition, the antioxidant activity of Cuminum cyminum L. was found to be higher than that of some common spices such as Z. officinale, C. sativum, A. sativum, C. tamala, C. verum, and E. cardamomum (Sultana et al., 2010).
Various thermal extraction methods, such as reflux and Soxhlet extraction, have been applied in the extraction of biologically active compounds and polyphenols from plant materials (Al Juhaimi and Ghafoor, 2013). However, the effectiveness of these methods is compromised by the loss of polyphenols due to oxidation hydrolysis and ionization during extraction, as well as the persistent issue of longer extraction time. Recently, extraction techniques such as ultrasonic-assisted extraction, microwave-assisted extraction, accelerated solvent extraction, and supercritical fluid extraction have been developed for the extraction of polyphenolic compounds from plants (Ong, 2004). Among these techniques, ultrasonic-assisted extraction (UAE) is a simple, economical, and effective alternative to conventional extraction techniques (Ilghami et al., 2015). Additionally, UAE is a quick and effective extraction method that uses ultrasonic waves to create the cavitation effect in the solvent by which the movement of small vacuum bubbles in the liquid increases penetration of the solvent into the raw plant materials (Teng et al., 2014).
Response surface methodology (RSM) was used to optimize the extraction conditions, including extraction temperature, ethanol concentration, and extraction time. RSM is a powerful mathematical and statistical-based technique that is widely used by researchers for the purpose of optimizing the parameters of an extraction procedure (Bimakr et al., 2012; Ilghami et al., 2015). To the best of our knowledge, many studies have employed RSM to optimize the UAE conditions for the extraction of phenolic compounds (Al Juhaimi and Ghafoor, 2013; Domingos et al., 2008; Haji and Qavamnia, 2015), but there is none on the application of RSM in optimizing UAE for the extraction of bioactive components from cumin seeds (C. cyminum L.). Thus, our study aimed to determine the optimal UAE conditions for the extraction of cumin seeds with high yield of total phenol content and antioxidant activity, as well as the ability to absorb free radicals that could be measured using DPPH and ABTS analyses and verified using RSM.
Materials and methods
Dried cumin (Cuminum Cyminum L.) seed samples were obtained from Hwami Co., Ltd., Incheon, Korea. Cumin seeds were milled into powder form using a laboratory mill (RT-04, Yung Kang, Taiwan). Cumin powder was passed through a 425 μm mesh sieve and retained on a 180 μm mesh sieve (between the sieves), kept in a polyethylene zip bag, and stored at −20°C until further use. Cumin seed extract was referred to as the cumin extract in this study.
All chemicals and solvents used for the extraction procedure, such as organic solvent ethanol and sodium carbonate, were purchased from Duksan Pure Chemical Company (Ansan, Korea).
Gallic acid, Folin-Ciocalteu’s phenol reagent, 1,1- diphenyl-2-picrylhydrazyl (DPPH) and 2,2'-azino-bis-(3- ethylbenzthiazoline-6-sulfonic acid) (ABTS) were obtained from Sigma Chemicals Co. (St. Louis, MO, USA).
In this study for ultrasonic-assisted extraction (UAE) was consumed the sonication cleaning bath (JAC-3010, KODO, Hwaseong, Korea). The apparatus has the following characteristics: a frequency of 40 kHz, an ultrasonic input power of 250 W, and volume of 10 L (overall size 300× 240×150 mm). One gram of cumin powder was added to the 20 mL of solvent (0-80% of ethanol) in the 50 mL conical tubes (30×115 mm), then the samples were put in an ultrasonic bath for the extraction. Then the extract was filtered (Whatman No. 1) via a Buchner funnel under vacuum conditions, after then the filtered extract was placid in the 15 mL conical tubes (17×120 mm).
The extraction yield of cumin extract was defined as total soluble solid content after the evaporation of extraction solvent and moisture. The extraction yield content of cumin extract was determined by gravimetric method (AOAC, 2003). Cumin seeds extract (2 mL) was placed in a disposable aluminum dish with a diameter of 70 mm and dried 24 hours in the oven at 105°C, and then the dish was cooled for 10 minutes before weighing. The weight difference was used to calculate the percentage of the extraction yield content of cumin seeds extract.
Where W1 represents the weight of cumin powder, W2- weight of dried cumin extract, W3 initial weight of cumin extract before drying.
TPC was measured using Folin-Ciocalteu’s method as previously described (Singleton et al., 1999). Before reaction, cumin seeds extract was diluted well with distilled water and 100 μL of diluted samples was a mixed with 50 μL of the Folin-Ciocalteu’s reagent and 300 μL of 2% Na2CO3. After keeping for reaction of the sample at room temperature during 15 min, 1 mL of distilled water was added, and mixture was vortexed quickly before measuring the absorbance at 725 nm. The milligrams of gallic acid equivalents per gram of dry weight (mg GAE/g) was used to express the results.
The cumin extracts antioxidant activity was analyzed as well as the ability to absorb free radicals measured by DPPH assay (Blois, 1958). 100 μL Cumin extracts were added to the 90 μL of DPPH solution (1 μmol/L in ethanol). Then the sample was shaken vigorously and kept for the reaction for 30 min at dark place. Then the decrease in absorbance was measured at 517 nm. The ethanol was used as a control instead of the sample. DPPH was expressed by the following equation:
ABTS was performed accordingly by the method described by (Shalaby and Shanab, 2013) with some modification. This was on the assumption of the capability of various substances to scavenge ABTS radical cation. The radical cation was prepared by mixing 7 mM 0.0406 g ABTS stock solution with 0.007 g potassium persulfate and leaving the mixture for 12-16 h in the dark place. Cumin extracts 50 μL were reacted with 950 μL of ABTS solution. The measurements were taken immediately at 724 nm after 30 min reaction at dark place. Control was ethanol used instead of the sample. ABTS was calculated by the following equation:
Response surface methodology (RSM) was used to optimize the extraction conditions using a statistical analysis system (SAS) software. There were three independent variables: ethanol concentration (X1), extraction time (X2), and extraction temperature (X3). By using single-factor experiments and central composite design (CCD), the parameters of ethanol concentration (0, 20, 40, 60, and 80%), extraction time (15, 30, 45, 60, and 75 min), and extraction temperature (15, 30, 45, 60, and 75°C) were selected as suitable conditions and were encoded at five levels (-2, −1, 0, +1, and +2) (Table 1). The CCD matrices consisted of six center points, eight factorial points, and six axis points at a distance of ±2, which led to a total of 20 sets of experimental runs. The experimental data were combined into a second-order empirical polynomial model using regression analysis as presented in the following equation:
Xn | Response variables | Levels | ||||
---|---|---|---|---|---|---|
-2 | -1 | 0 | +1 | +2 | ||
X1 | Extraction temperature (°C) | 15 | 30 | 45 | 60 | 75 |
X2 | Ethanol concentration (%) | 0 | 20 | 40 | 60 | 80 |
X3 | Extraction time (min) | 15 | 30 | 45 | 60 | 75 |
where Y symbolizes the independent responses; β0, βi, βii, and βij are the regression coefficients of the process variables for the intercept, linear, quadratic, and cross-product terms; respectively, and symbolizes the error. The statistical significance of the coefficients in the regression equation was verified using the SAS software. The suitability of the polynomial model equation for the predicted response was evaluated using coefficient R2.
The cumin extracts obtained under optimal conditions were analyzed for phenolic compounds using the method described by Seal (2016). The HPLC system (Jasco International Co. Ltd., Tokyo, Japan) consisted of a UV detector (UV-2075 plus, Jasco International Co. Ltd.) and a C18 reversed-phase column (250×4.6 mm, 5 μm). The column was eluted using 1% acetic acid aqueous solution (A) and acetonitrile (B) at a flow rate of 0.7 mL/min. The elution gradient was as follows: 0–10% B, 28–40% B, 39–60% B, and 50–90% B. The UV detector was set at 272 nm, and the injection volume was 20 μL. Catechin, caffeic acid, syringic acid, p-coumaric acid, rutin, ferulic acid, myricetin, quercetin, and kaempferol were obtained from Sigma Aldrich Co. (St. Louis, MO, USA) and were used as standard materials.
All experiments were performed in triplicate, and results were expressed as mean±standard deviation. Four-dimensional response surface plots were obtained using the Mathematica program (Version 12.0, Wolfram, Champaign, IL, USA) and SAS software (Version 9.2, SAS Institute Inc., Cary, NC, USA).
Results and discussion
In optimization studies, a second-order polynomial model is an official empirical model that is generally used by process engineers (Myers and Montgomery, 2009). Table 1 shows the CCD for optimized UAE conditions for cumin seeds, which was illustrated in the model using the least-squares method. SAS software was used to define the significance and adequacy of the selected model. The CCD matrix indicating extraction yield, TPC, DPPH, and ABTS radical scavenging activities of cumin seed extracts using UAE is summarized in Table 2. Results of the three target responses from the CCD matrix were also subjected to analysis using SAS software. The second polynomial equations for the quality prediction of cumin extract are shown in Table 3. The reliability of the model was confirmed by the values of coefficient of determination, R2, which are represented by the regression equations shown in Table 3. The fitted model showed the following R2 values for yield (0.9077), TPC (0.9131), DPPH (0.9008), and ABTS (0.8882). To predict the correlation between independent process variables and dependent responses, four-dimensional surface plots were created. The type of solvent plays an important role in the extraction of biologically active compounds from plant sources (Wang et al., 2008). Therefore in this study, ethanol was used as the extraction solvent due to its low toxicity and easy recovery using reduced-pressure distillation (Wang et al., 2008).
The experimental results for extraction yield are presented in Table 2. The coefficient of determination for the polynomial equation was 0.9077 and significance was less than 0.001% (Table 3). The peak point of the extraction yield was 22.1% in the test run 4 under following UAE conditions: the extraction temperature of 30°C, the ethanol concentration of 60% and an extraction time of 60 minutes. While the lowest yield was 11.9% in run 12, which used conditions of extraction temperature of 45°C, ethanol concentration of 80%, and extraction time of 45 minutes. Based on the second polynomial equation (Table 3), four- dimensional response surface plots were created and shown in Fig. 1A-D. In the Fig. 1A showed four-dimensional response surface plots presenting constant levels for yield of 18, 19, 20 and 22%. This figure reflected the effects of variables in UAE process, including extraction temperature, ethanol concentration, and sonication time on yield. The yield of more than 20% could be obtained at a low temperature range of 15-35°C for 45-75 min or at a high temperature range for 35-55°C for 25-45 min. Our result suggested that higher temperature benefit yield extraction and reduce extraction time, science higher extraction temperature could increase the movement of solvent molecules and accelerate mass transfer ratios, thus, reducing the extraction time. Extraction of solids and liquids is a mass transfer phenomenon in which solids contained in a matrix are transferred to a solvent that comes into contact with the matrix (Ghafoor et al., 2009). This mass transfer phenomenon can be improved by varying the diffusion coefficients caused by ultrasound and extraction temperature. Ultrasonic waves destroy plant matrix cells and contribute to the release of cell contents into the extraction medium (Vinatoru et al., 1997). These were also explained by Corrales (2008) that the phenomenon of mass transfer at elevated temperature increases the internal liquid phase, which increases the pressure, causing centrifugal circulation of dissolved substances through the installation elements. In addition, heat treatment can also destroy the bonds of the phenolic matrix and affect the membrane structure of plant cells, making them less selective due to coagulation of lipoproteins (Corrales et al., 2008).
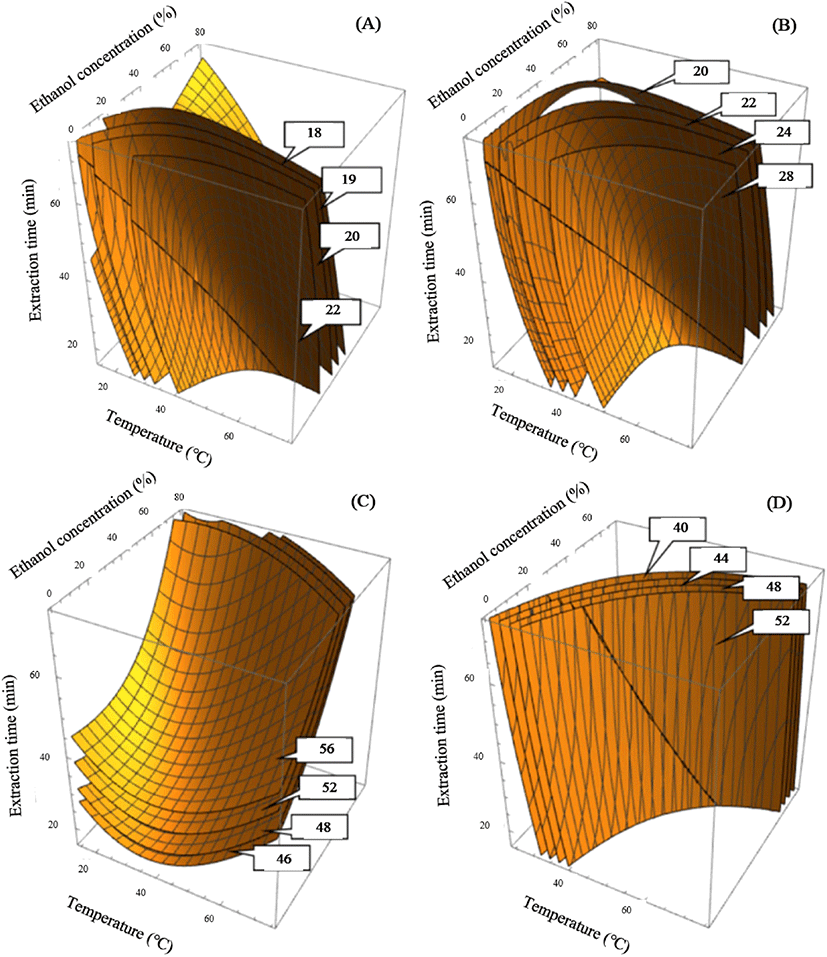
In Fig. 1A, the extraction yield increased substantially with the increase in ethanol concentration, and a satisfactory yield of more than 20% could be reached at an ethanol concentration range of 20-60%. The effect of ethanol concentration on the yield can be explained by the fact that adding water to ethanol improves the extraction rate (Tomšik et al., 2016).
The model predicted a highest extraction yield of 20.31% under the following extraction conditions: X1=39°C, X2= 40% and X3=51 min. The experimental extraction yield value of 21.0% was almost same with the model-predicted value (20.31%). The highly substantial effect was found in ethanol concentration and extraction temperature, as can be seen from the graph of the reaction surface 4D, shown in Fig. 1A. Thus, the extraction temperature (30-45°C), ethanol concentration (20-60%) and extraction time (45-60 min) showed favorable results for yield of cumin seeds extract.
Our experimental data for TPC are presented in Table 2. The R2 for a polynomial equation was 0.9131 at a significance level of p<0.005 (Table 3). The peak point of TPC at 27.49 mg GAE/g was observed at an ethanol concentration of 60%, extraction temperature of 30°C, and extraction time of 60 min (Run 4, Table 2). The lowest TPC of 16.91 mg GAE/g was obtained in test run 13, in which distilled water was used as a solvent for the extraction at 45°C for 15 min. The predicted maximum TPC was 27.1 mg GAE/g (Table 4). The response surface plots indicated the highest predicted peak point of TPC at constant values of 20, 22, 24, and 28 mg GAE/g (Fig. 1B). Accordingly, for the four-dimensional response surface plot results, optimum extraction conditions for satisfactory TPC of more than 25 mg GAE/g comprised the extraction temperature (30-45°C), ethanol concentration (20-40%), and sonication time (30-45 min).
According to Murakami et al. (2004), heat treatment can destroy the cell wall and subcellular compartments of plant materials to release more components. TPC increased significantly from 20 to 27 mg GAE/g following the increase in extraction temperature from 30 to 45°C, after which TPC decreased (Fig. 1B). High temperature weakens the cell walls and increases the solubility and diffusion coefficients of the extracted compounds, thereby facilitating mass passage through the solid substrate. (Yue et al., 2012). However, excessively high heat treatment can destroy the polyphenolic compounds due to the accelerated hydrolysis, internal redox reactions, and polymerization (Alonso-Salces et al., 2001).
The significance of temperature, ethanol concentration, and extraction time of UAE for different plant materials has been extensively studied (Al Juhaimi and Ghafoor, 2013; Knorr et al., 2004; Yue et al., 2012). Collectively, these results showed that a 60% ethanol concentration gives the highest TPC yield of 27.49 mg GAE/g, indicating a maximum appropriate polarity of ethanol concentration at 60% (Al Juhaimi and Ghafoor, 2013; Yue et al., 2012).
An optimized extraction method with parameters of ethanol concentration (53.6%), extraction temperature (49°C), and extraction time (2.8 h) that could obtain the highest TPC yield (24.66 mg GAE/g) from cumin seeds using a shaking water bath has been reported (Al Juhaimi and Ghafoor, 2013). In our present study, similar conditions for temperature and ethanol concentration were observed, except the extraction time. Considering all results together, we demonstrated that UAE is a highly effective method that saves time and cost compared to the traditional extraction techniques. Knorr (2004) also reported that UAE increases the rate of mass transfer due to cavitation forces, by which the breaking of small vacuum bubbles in the liquid/solid can create localized pressure, accelerating the rupture of plant tissues and release of intracellular substances into the solvent.
The experimental data for DPPH assay are shown in Table 2. The correlation coefficients of the polynomial equation results were 0.9008 at a significance level of p<0.001 (Table 3). The highest DPPH of 54.92% was detected in test run 4 under UAE conditions of ethanol concentration of 60%, extraction temperature of 30°C, and extraction time of 60 min. Minimal DPPH (36.88%) was obtained in test run 13 under UAE conditions of an extraction temperature of 45°C, ethanol concentration of 0%, and extraction time of 15 min. The response surface plot for DPPH radical scavenging activity demonstrated the highest prediction point at values of the constant of 46, 48, 52, and 56% (Fig. 1C). The extraction temperature of 15-45°C, ethanol concentration of 40-60%, and extraction time of 45-60 min showed good results for the DPPH radical scavenging activity of cumin seed extract. After optimization, the model predicted maximum DPPH radical scavenging activity of 52.47% under the following extraction conditions: extraction temperature of 39°C, ethanol concentration of 40%, and extraction time of 51 min. The experimentally obtained amount of DPPH (55.71%) was almost the same as the value predicted by the model (52.47%, Table 4).
The experimental data for ABTS scavenging activity are presented in Table 2. The determination coefficient of the polynomial equation was 0.8882 at a significance level of p<0.001 (Table 3). The highest ABTS of 44.59% was observed at X1= 60°C, X2= 60%, and X3= 30 min (Run 7, Table 2). The lowest ABTS of 26.08% was observed in run 9 under the following UAE conditions: extraction temperature of 15°C, ethanol concentration of 40%, and extraction time of 45 min. The predicted maximum ABTS was 41.04% (Table 4). The response surface plot for ABTS radical scavenging activity indicated the highest predicted level at a constant quantity of 40, 44, 48, and 52% (Fig. 1D). Extraction temperature of 45-60°C, ethanol concentration of 20-60%, and extraction time of 45-60 min showed suitable results for ABTS radical scavenging activity of cumin seed extract. After optimization, the model predicted a maximum ABTS radical scavenging activity of 41.04% under the following extraction conditions: extraction temperature of 39°C, ethanol concentration of 40%, and extraction time of 51 min. The experimental ABTS value of 44.12% was similar to the model-predicted value (41.04%, Table 4).
All the extraction conditions (extraction temperature, solvent concentration, and sonication time) had a considerable influence on the DPPH radical scavenging activity. However, the duration of extraction was observed to be of little importance to ABTS scavenging activity. Our results showed that the DPPH radical scavenging activity increased for a short time with increasing temperature, but at low temperature the activity was maintained over a longer period of extraction time. It has been suggested that the bioactive compounds in plant materials may not dissolve completely in the solution without heat treatment (Khatun et al., 2006), which can destroy the cell wall and the subcellular compartments of plant materials. Evidently, heat treatment or thermochemical reactions can be applied to increase the release and formation of radical-absorbing compounds (Maeda et al., 1992). Furthermore, Kishk and El-Sheshstawy (2010) proposed that increasing the temperature of ginger extraction led to a decrease in the radical scavenging activity due to the increased damage of phenolic compounds at higher temperature. Therefore, determining the optimal extraction temperature for the UAE is crucial.
The samples extracted with a 60% ethanol concentration had a better scavenging capacity for DPPH and ABTS radicals. The extraction time affects antioxidant activity due to the increased exposure duration of the solvent with solids, and thereby increases the diffusion of components (Ghafoor et al., 2009). The optimal extraction time for maximum absorption capacity of DPPH radicals was 60 min. Cumin extracts obtained at 30 min showed greater scavenging capacity for ABTS radicals. This may be due to the fact that the antioxidant compounds in extracts obtained at various timepoints were examined in separate assays (DPPH and ABTS purification assays), as explained by Chang-Laing (2015).
Suitable models for all responses were very significant and reliable in the experimental field, and canonical analysis confirmed that the critical points for all answers were maximum in the experimental field. The four-dimensional response surfaces were superimposed to predict the optimum UAE conditions, indicated by the maximum predicted peak at constant values for yield at 18-20%, TPC at 24-28 mg GAE/g, DPPH at 54-56%, and ABTS at 46-52% (Fig. 2). Optimal UAE conditions were as follows: temperature 39°C, ethanol concentration 40%; and extraction time 51 min, for predicted values of yield at 20.31%, total polyphenol content at 27.11 mg GAE/g, DPPH at 52.47%, and ABTS at 41.04% (Table 4). To confirm the accuracy and reliability of the predicted models, as well as to evaluate the deviation between the estimated and experimental values under the predicted optimal conditions, verification experiments was performed. Experimental values obtained were yield 21.0%, total polyphenol content 26.8 mg GAE/g, DPPH 55.7%, and ABTS 44.1% (Table 4). No significant differences were observed between the experimental and estimated values, thus verifying that the models fitted well with the experimental data and were valid and reliable. When compared to the predicted value substituted in the conformity model type, the experimental value appears to be 98.86-107.5% of the predicted value was recognized for the effectiveness of the extraction conditions (Table 4). Our results revealed that the predicted model is suitable for the high yield extraction of bioactive components from cumin under optimal UAE conditions, and the designed model is an ideal tool for predicting optimal extraction conditions.
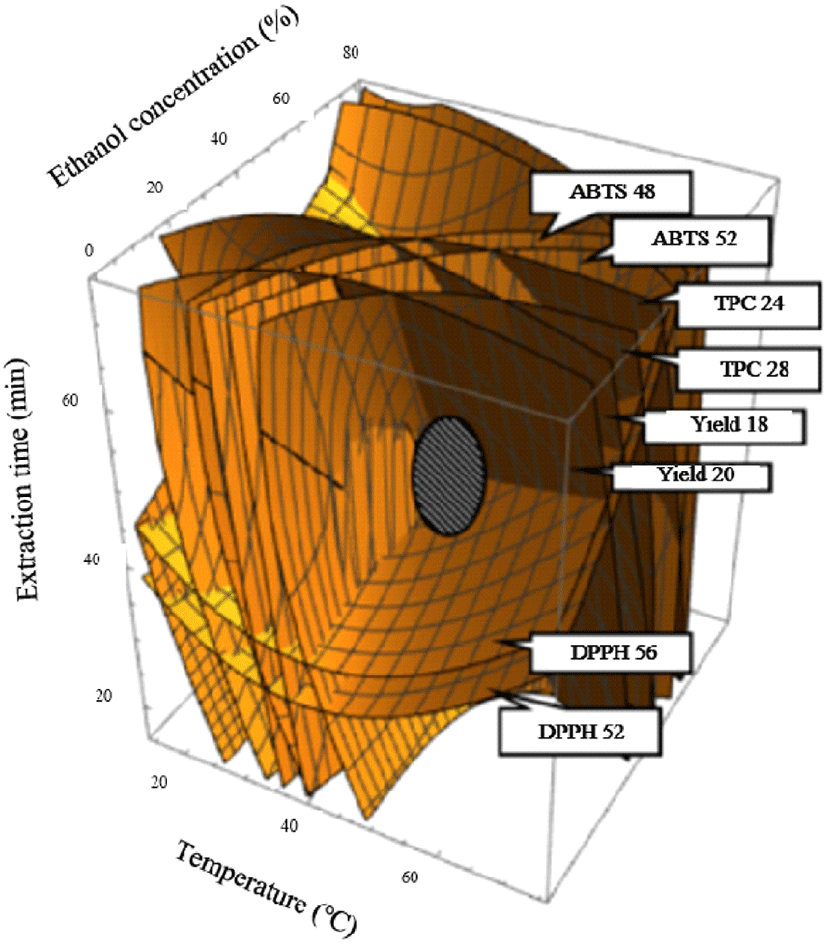
The phenolic compounds in cumin extract is shown in Table 5. The 9 phenolic compounds (catechin, caffeic acid, syringic acid, p-coumaric acid, rutin, ferulic acid, myricetin, quercetin, and kaempferol) were identified from extract. Among them, myricetin and rutin were predominant compounds. The composition of phenolic compound was different with previous studies (Rebey et al., 2012a; Rebey et al., 2012b). This difference may be attributed by various factors, such as environmental factor and extraction condition (Rebey et al., 2012a; Rebey et al., 2012b).
Conclusion
This study was focused on to find optimal extraction conditions to extract of bioactive compounds from cumin seeds operating the ultrasonic-assisted extraction method. This method of extraction is an ecologically sustainable technique with very effective, lower power-efficient, and without applying of high pressure or temperature. Thus, phytochemical compounds can be extracted from natural plants at considerably low temperatures without undergoing thermal decomposition, maintaining their original form for biological activity analysis. In the present study, bioactive compounds such as polyphenols and antioxidants were rapidly extracted from cumin seeds using UAE. Based on the results the optimal UAE conditions were an temperature of 39°C, concentration of ethanol 40% (v/v) and 51 min of extraction time supposed using superimposed surface plots, that provides the expected highest amounts of yield (20.31%), TPC (27.11 mg GAE/g), antioxidant activity DPPH (52.47%) and ABTS (41.04%). Optimal conditions for maximal UAE extraction of TPC, DPPH, ABTS radical-scavenging activity and extract yield of Cumin (Cuminum cyminum) seeds have been effectively established by RSM. The HPLC analysis was performed to identify phenolic compounds in the extract, which obtained from optimal extraction condition. The results showed that extract include the catechin, caffeic acid, syringic acid, p-coumaric acid, rutin, ferulic acid, myricetin, quercetin, and kaempferol.