1. Introduction
Chickpeas (Cicer arietinum L.) are a major legume in human diets, and the global chickpea production in 2020 was estimated to be 15 million tons (FAO, 2022; Gupta et al., 2017). Unlike rice, wheat, and corn, chickpeas are not a staple food; however, they are an excellent source of carbohydrates, proteins, unsaturated fatty acids, dietary fiber, minerals, vitamins, and bioactive components such as isoflavones (Wang et al., 2021). Storage proteins are a mixture protein consisting of salt soluble protein, water soluble protein, and dilute acid/alkali soluble protein (Chang et al., 2022). Soluble proteins such as albumin (16.1%) and globulin (41.7%) are abundantly contained in chickpeas, and have high solubility and digestibility (Ferreria et al., 2019). Clemente et al. (2000) reported that albumins are constituted of many enzymatic and metabolic proteins, indicating they are essential for chickpea growth. Also, albumins play an important role in providing nutrient due to their high lysine and sulfur amino acids contents and their functionalities such as solubility in water, capable of enhancing the foaming properties of chickpea (Grasse et al., 2022). In addition, substantial numbers of phenolic compounds, including flavonols, flavone glycosides, oligomeric and polymeric proanthocyanidins, cinnamic acid, salicylic acid, hydroxycinnamic acid, coumaric acid, gallic acid, caffeic acid, vanillic acid, and ferulic acid, are found in chickpeas (Gupta et al., 2017). The potential health benefits associated with chickpeas include antioxidative, anti-inflammatory, tumor growth inhibitory, hypoglycemic, hypolipidemic, anti-hypertensive, hemagglutination, and gastroprotective effects (Wang et al., 2021). Therefore, chickpeas can be considered a suitable source of functional ingredients for dietary improvement.
Although rich in functional ingredients, chickpea protein is also associated with the presence of anti-nutritional factors, such as trypsin inhibitors, lectins, phytic acid, and sulfur-containing compounds, which may reduce its biological value (El-Adawy, 2002; Wang & Guo, 2021). To remediate these factors, thermal treatments are generally used but can decrease the digestibility of proteins and bioaccessibility of phenolic compounds (El-Adawy, 2002). Germination is a non-thermal treatment that can effectively decrease anti-nutritional factors without damaging the nutritional quality of the seeds (Nitride et al., 2022). During germination, proteins are chemically altered and degraded to provide nitrogen, carbon, and sulfur to the embryo, which consequently reduces the concentration of trypsin inhibitors and lectins while enhancing the bioaccessibility of phenolic components and digestibility of proteins (Nitride et al., 2022). Moreover, Chandrasekaran and Gonzalez de Mejia (2022) reported that germinated chickpeas can produce bioactive peptides with antioxidant, angiotensin-converting, enzyme inhibitory, and anti-inflammatory properties. Hence, if the goal is to improve the nutraceutical components and health-promoting potential of chickpeas, germination may be a promising method.
A few studies have reported the application of germinated chickpeas as a functional supplement in foods, such as bread, noodles, and snacks (Baljeet et al., 2014; Ouazib et al., 2016; Sofi et al., 2020). The germinated chickpeas used in these studies were prepared under various soaking conditions, temperatures, and germination periods. Previous study reported the optimal germination conditions upon process variables: germination temperature (20-35°C) and time (10-240 h) with respect to response variables: total phenolic and flavonoid content, and antioxidant activity (AoxA) (Domínguez-Arispuro et al., 2018). There has still lack to study on the optimization of germination condition including the soaking time of seed and important response parameter such as soluble protein.
Therefore, this study optimized the germination conditions, specifically the soaking time, germination temperature, and germination period, with respect to the total phenolic content (TPC), total flavonoid content (TFC), 2,2-diphenyl-1-picrylhydrazyl (DPPH) radical scavenging activity, and soluble protein content, using the Box-Behnken design (BBD) method. The final objective of this study was to optimize the germination conditions that increased the bioactive components in chickpeas and improved bioavailability to allow their use as a food supplement.
2. Materials and methods
Chickpea seeds (C. arietinum) were purchased from a local market (Changwon-si, Korea). Folin-Ciocalteu reagent, gallic acid, (+)-catechin hydrate, DPPH, and Trolox were purchased from Sigma-Aldrich Co., Ltd. (St. Louis, MO, USA). Sodium nitrite, aluminum chlorite, and sodium carbonate were obtained from Junsei Chemical Company (Tokyo, Japan). Sodium hydroxide, hydrochloric acid, and ethanol were purchased from Duksan Chemical Company (Ansan, Korea).
Chickpea was germinated according to the method described by Xu et al. (2019). Briefly, 250 g of chickpeas were sterilized with 1,000 mL of 0.07% sodium hypochlorite solution for 30 min and subsequently washed with distilled water to reach a neutral pH. After sanitization, the chickpeas were soaked in 1,000 mL of distilled water at 6, 12, and 18 h at room temperature (25°C±2). The hydrated chickpeas were drained using a sieve, covered with a wet cloth and germination was performed under the dark in an incubator (HK-BI025, Korea General Instruments Works Co. Ltd., Hwaseong, Korea). During germination, the seeds were sprayed with 100 mL of distilled water every 12 h. After germination, each sample was freeze-dried using a freeze dryer (Bondiro, Ilshin Biobase Co. Ltd., Yangju, Korea). The freeze-dried samples were ground using a mill (CGS-H1901, EMART Inc., Seoul, Korea) and stored at −70°C until extraction.
The germination conditions were optimized using BBD in response surface methodology (RSM). The three independent variables were soaking time (X1: 6, 12, and 18 h), germination temperature (X2: 20, 25, and 30°C), and germination duration (X3: 2, 4, and 6 d), which were indicated by −1, 0, and 1 (low, middle, and high levels) (Table 1).
Independent variables | Levels | |||
---|---|---|---|---|
−1 | 0 | 1 | ||
X1 | Soaking time (h) | 6 | 12 | 18 |
X2 | Germination temperature (°C) | 20 | 25 | 30 |
X3 | Germination day | 2 | 4 | 6 |
The effects of the three independent variables on dependent variables (Y), namely TPC, TFC, DPPH radical scavenging activity, and soluble protein content, were modeled using BBD in RSM. The 17 experimental runs included 12 factorial and 5 axial points (Table 2).
The quadratic equation for the dependent variables was predicted using the following equation:
Where β0, βi (i =1, 2, 3), and βij (i =1, 2, 3; j =1, 2, 3) are the model coefficients, Xi and Xj are the coded independent variables, and ε is the random error.
Each germinated chickpea (1 g) was extracted using 5 mL of 70% ethanol solution at 65°C for 3 h in a water bath (BS-21, JEIO TECH. Co. Ltd., Daegu, Korea). After extraction, each mixture was centrifuged at 1,628 ×g for 10 min and filtered through a 0.45-μm membrane. Subsequently, the filtrates were stored at −20°C until analysis.
The TPC of the germinated chickpeas was determined using the Folin–Ciocalteu method, as described by Gujral et al. (2013). Each extract (2 μL) was mixed with 50 μL of 10% Folin-Ciocalteu reagent and 150 μL of 20% sodium carbonate solution. The mixtures were allowed to react in the dark at room temperature. After 2 h, the absorbance of each mixture was measured at 765 nm using a spectrophotometer (EMC-11D-V; EMCLAB Instruments, Duisburg, Germany). The results were expressed in milligrams of gallic acid equivalents per gram of dry weight (mg GE/g).
The TFC was determined using the method described by Aung et al. (2022a). Briefly, 0.1 mL of chickpea extract and 1.25 mL of distilled water were mixed. Subsequently, 75 μL of 5% sodium nitrite solution was added and mixed for 6 min, after which 150 μL of 10% aluminum chlorite was added to the mixture. After 5 min, 0.5 mL of 1 N sodium hydroxide was added, and the absorbance was measured at 510 nm using a spectrophotometer (EMC-11D-V, EMCLAB Instruments). The standard used was (+)-catechin hydrate, and the results were expressed in milligrams of catechin equivalents per gram of dry weight (mg CE/g).
The DPPH radical scavenging activity of the germinated chickpeas was measured using the method described by Kim et al. (2020). DPPH solution (0.1 mM) was prepared with 100% methanol to measure DPPH radical scavenging activity. Briefly, 5 μL of extract and 195 μL of DPPH solution were mixed and allowed to stand for 30 min at room temperature in the dark. The absorbance was measured at 515 nm using a spectrophotometer (EMC-11D-V, EMCLAB Instruments). Methanol and Trolox were used as blank and standard, respectively. The results were expressed as micromoles of Trolox equivalents per gram of dry weight (μM TE/g).
Soluble protein content was determined following the Lowry method, as described by Goswami et al. (2021) and Lowry et al. (1951), with some modifications. Chickpea flour (0.5 g) was mixed with 0.2 M phosphate buffer (pH 7.5, 5 mL) and centrifuged at 7,000 rpm for 20 min. The supernatant was collected and used for soluble protein content analysis. For this, 0.1 mL of chickpea extract was added to 0.1 mL of 2 N NaOH, and the mixture was heated at 100°C for 10 min. After 5 min at room temperature, 0.1 mL of 1 N Folin-Ciocalteu reagent and 1 mL of complex reagent, composed of 2% sodium carbonate, 1% copper (Ⅱ) sulfate pentahydrate, and 2% sodium potassium tartrate in a ratio of 100:1:1 (v/v/v), were added. After 45 min of incubation at room temperature, the absorbance was measured at 750 nm using a spectrophotometer (EMC-11D-V, EMCLAB Instruments). Bovine serum albumin was used as a standard, and the results were expressed as milligrams per gram of dry weight (mg/g).
Design-Expert Software version 11 (Stat-Ease Inc., Minneapolis, MN, USA) was used to analyze the experimental data to obtain the best-fit model equations and response plots for TPC, TFC, DPPH radical scavenging activity, and soluble protein content. The most desirable overall combination of soaking time, germination temperature, and germination duration was selected as the optimal germination condition. To validate the predicted optimal conditions, chickpeas were germinated at these conditions as determined by BBD in RSM and analyzed for the selected responses. The absolute residual error (%) was calculated from the predicted and actual data using the following equation:
The data are expressed as the mean±standard deviation of three replicates. Analysis of variance (ANOVA) was used to determine differences among samples, and Duncan’s multiple range test was conducted to determine significant differences at p<0.05 among sample means using the XLSTAT software (Addinsoft, Paris, France).
3. Results and discussion
RSM based on BBD was used to optimize the germination conditions (soaking time, temperature, and duration) with respect to the responses (TPC, TFC, DPPH activity, and soluble protein content) (Table 2). The independent variables were expressed according to the soaking duration (6, 12, and 18 h), germination temperature (20, 25, and 30°C), and germination duration (2, 4, and 6 d), which were displayed with codes −1, 0, and +1, respectively. As shown in Table 2, responses in the ranges of 0.91-1.53 mg GE/g for TPC, 0.26-0.54 mg CE/g for TFC, 1.71-3.65 M TE/g for DPPH radical scavenging activity, and 124-144 mg/g for soluble protein content were observed at the proposed optimal germination conditions. To achieve the goodness of the model, the removal of insignificant terms (p>0.05) were eliminated and different models were used in calculation for realistic model (Aung et al., 2022b). The obtaining final equations of responses were as follows:
To fit the model, the important model response parameters, including regression coefficient (R2) value, adjusted R2 value, p-value, lack of fit (p-value), and adequate precision, were evaluated and are shown in Table 3. Model significance was indicated by p<0.05 for TPC, TFC, DPPH activity, and soluble protein content. Furthermore, an insignificant lack of fit (p-value) (p>0.05) indicated that the model was good. The R2 values for TPC, TFC, DPPH radical scavenging activity, and soluble protein content were 0.862, 0.844, 0.715, and 0.829, respectively. The high R2 values and similar adjusted R2 values revealed that the examined variables were reliable and high values closer to 1 represents a better fit model (Aung et al., 2022b). All value showing higher than 0.7 were assumed as acceptable level for calculation (Umar et al., 2018). Adequate precision existed for TPC (12.37), TFC (7.32), DPPH activity (9.75), and soluble protein content (6.94) (Table 3). According to Aung et al. (2022b), adequate precision describes the signal-to-noise ratio, and high precision indicates that the fitted model has an adequate signal to navigate the design space. Therefore, the model was assumed to be suitable for optimizing the germination conditions for chickpeas.
The TPC values of the chickpea extracts obtained from various germination conditions determined by BBD are presented in Table 2, and three-dimensional surface plots are shown in Fig. 1. The highest TPC value (2.10 mg GE/g) among samples was observed in run number 9, which used a soaking time of 12 h, temperature of 30°C, and duration of 6 d (Table 2). In contrast, the lowest TPC value (0.91 mg GE/g) was obtained from run number 13, which used a soaking time of 6 h, temperature of 25°C, and duration of 2 d. As shown in Table 3 and Fig. 1(A)-(C), the TPC values of the germinated chickpeas were affected more by germination duration and temperature than by soaking time. A similar trend was also reported by López-Martínez et al. (2017), who showed that temperature is an important factor for TPC values during the germination of legumes or pulses. Additionally, an increase in TPC in legumes or pulses during germination might be due to the release of bound phenolic compounds to free phenolics from cell walls by endogenous enzymes (Bubelova et al., 2018). Mamilla and Mishra (2017) reported that the hydrolase and polyphenol oxidase activities during legume germination drive the release of free phenolics from seeds.
TPC, total phenolic content (mg GAE/g); TFC, total flavonoid content (mg CE/g); DPPH RSA, DPPH radical scavenging activity (μM TE/g); GE, gallic acid equivalent; CE, catechin equivalent; TE, trolox equivalent; R2, coefficient determination; Adeq precision, adequate precision.
The TFC values of germinated chickpeas in the 17 experimental runs were determined using an AlCl3 colorimetric assay, and the results are shown in Table 2 and Fig. 1(D)-(F). The TFC values ranged from 0.54±0.04 to 0.23±0.01 mg CE/g, indicating a significant difference at p<0.05. The increase in TFC values was affected more by germination duration than by temperature or soaking time, as shown in Fig. 1(E) and (F). Similarly, Mesfin et al. (2021) reported that flavonoid content increased in germinated chickpeas as the germination time increased. These results were attributed to enzymatic biosynthesis in the seed coats and cotyledons with increased germination time. Moreover, these biochemical metabolic processes might produce other secondary plant metabolites due to enzymatic activation (Lin and Lai, 2006). Flavonoids are a group of health-promoting compounds with antioxidant and biological activities; thus, germination is a useful method for enhancing flavonoid content in legumes and pulses (Guo et al., 2012).
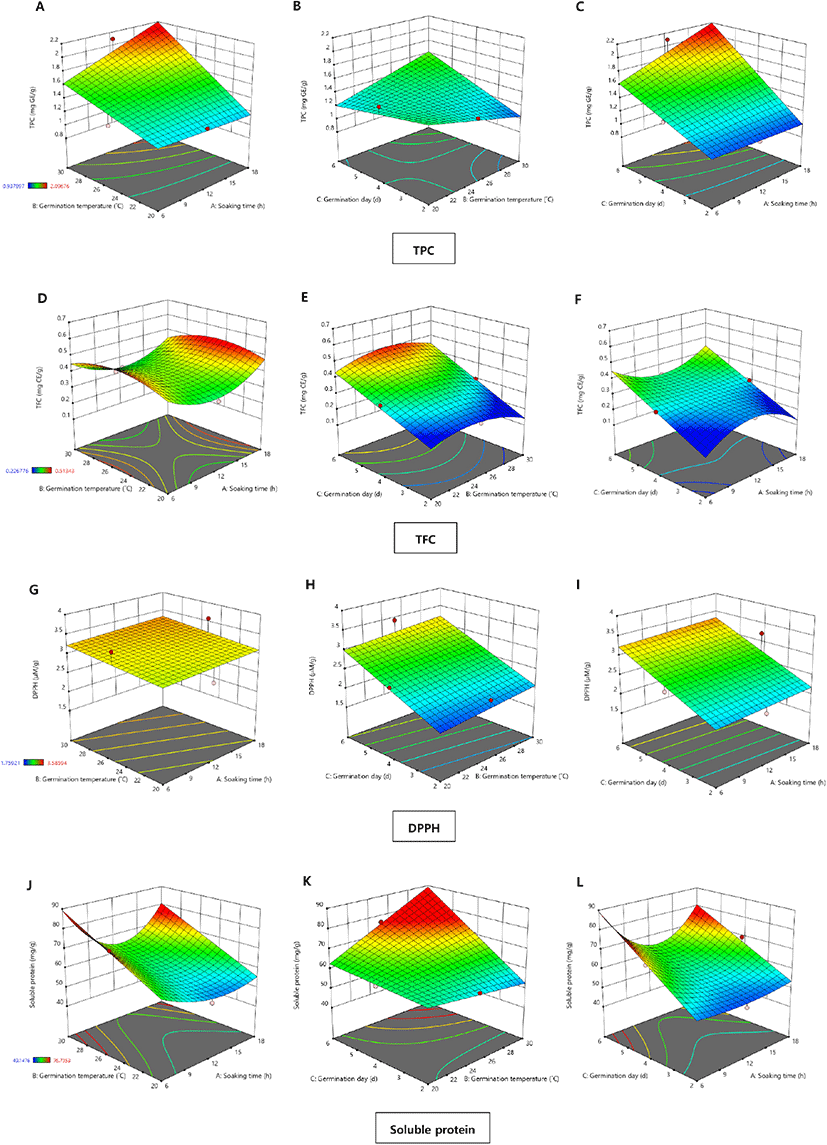
The DPPH radical scavenging activity of the germinated chickpea samples is presented in Table 2 and Fig. 1(G)-(I). Significant differences were observed in DPPH radical scavenging activity (p<0.05) among the 17 experimental runs, which ranged from 1.71 to 3.65 μM TE/g. The ANOVA results (Table 3) showed that only the germination duration positively affected the DPPH radical scavenging activity. As shown in Fig. 1(G)-(I), the three-dimensional response surface plots also showed a similar trend owing to increased concentrations of health-promoting compounds, such as TPC and TFC, in the germinated chickpeas after longer germination durations. According to Xu et al. (2018b), a longer germination time (6 d) had a strong effect on the antioxidative activity of germinated chickpea samples. Xu et al. (2018a) explained that this might be due to higher levels of soluble free phenolic compounds.
The soluble protein content of germinated chickpea samples is listed in Table 2. There were significant differences among the soluble protein content of different samples (p<0.05). The highest soluble protein content (76.7±2.25 mg/g) was from a sample germinated at 25°C for 6 d after soaking for 6 h. In contrast, the sample that was soaked for 12 h and germinated at 30°C for 2 d contained the lowest soluble protein (49.1±1.24 mg/g). In the study of sorghum germination by Singh et al. (2017), protein solubility of soaked and raw were insignificant. However, the increased protein solubility was observed for longer germination time at same germination temperature (30°C). Also, it increased at higher germination temperature (35°C) than lower temperature (25°C) for same time of germination (48 h). It can be assumed that the higher germination temperature and longer period of germination could increase the level of soluble protein. As shown in Fig. 1(J)-(L), soluble protein contents showed no significant changes when the soaking time increased at the same level of germination temperature and day. Thus, soluble protein content in germinated chickpeas was affected more by germination duration than by soaking duration or germination temperature. Moreover, these trends are shown in the three-dimensional response surface plots in Fig. 1(J)-(L), which illustrate the impact of germination time on the soluble protein content. Protein solubility and digestibility are important criteria for evaluating protein quality (Khattak et al., 2008). Ferreira et al. (2019) reported that large amounts of proteases synthesized during chickpea germination might play a role in increasing the soluble protein content.
The germination conditions were optimized by setting a goal of the maximum level for all responses. The model was validated by comparing the predicted and actual values of the responses under optimal conditions (Table 4). As shown in the overlay plot (Fig. 2), the optimal germination conditions were a soaking time of 6 h, temperature of 30°C, and duration of 6 d. The predicted values of the responses at this optimal condition were 1.63 mg GE/g for TPC, 0.40 mg CE/g for TFC, 3.22 μM TE/g for DPPH activity, and 89.23 mg/g for soluble protein content (Fig. 2, Table 4). The experimental values of these responses differed slightly from the predicted values and were 1.64 mg GE/g for TPC, 0.43 mg CE/g for TFC, 3.05 μM TE/g for DPPH activity, and 82.86 mg/g for soluble protein content. The absolute errors of all responses were less than 8%, which indicated the accuracy of the model design and a reasonable agreement between the predicted and actual values.
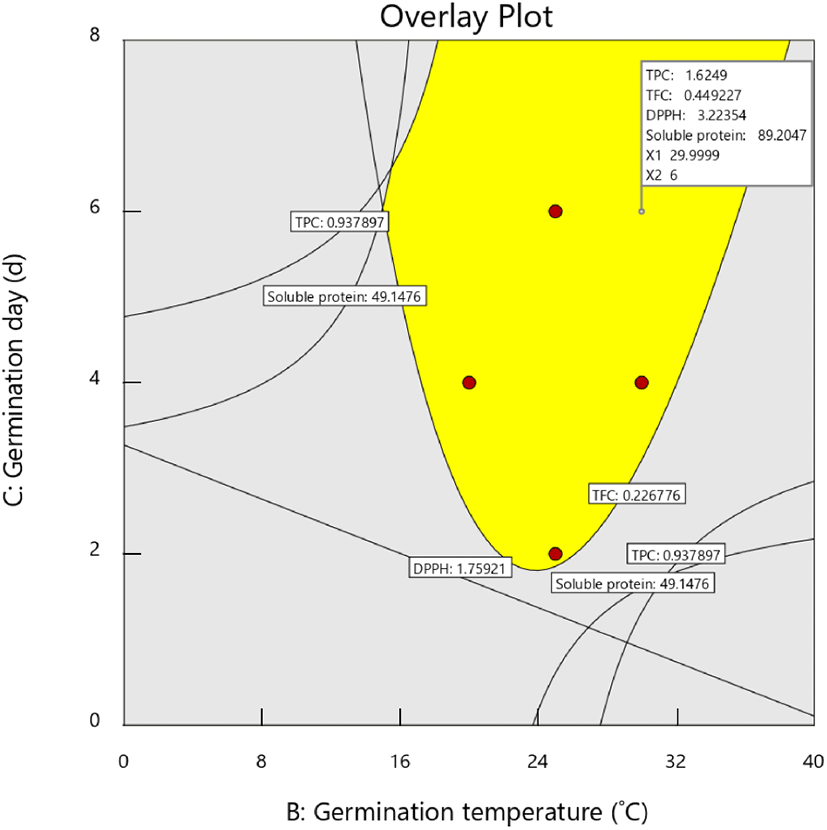
4. Conclusions
In this study, germination conditions were successfully optimized by considering various soaking times, germination temperatures, and durations to generate chickpeas with strong antioxidant potential, high total phenolic and flavonoid content, and high soluble protein content. Statistical evaluations demonstrated the reliability of the design used to optimize the parameters, and process verification confirmed the prediction accuracy. Experimental analysis indicated that, of the options examined herein, the shortest soaking time (6 h), highest germination temperature (30°C), and longest germination period (6 d) were the best conditions to optimize the selected responses. Furthermore, germinated chickpeas under these optimal conditions showed high levels of bioactive components, antioxidant activity, and soluble protein content. In terms of coded conditions, more studies required to analyze the trend of soaking time (6 h) or less, germination temperature (30°C) or more, and germination day (6 d) of later as boundary conditions. These studies will be helpful if you proceed with the central synthesis programming method. Further studies should be conducted to gain a clearer understanding of the metabolite profile of germinated chickpeas prepared under these optimal conditions.