1. Introduction
Coconut oil is produced from the kernel of mature coconuts which can be used for toothpaste (Shaheena et al., 2019), soap (Aerts et al., 2016), food products and supplements (Famurewa et al., 2017; Khor et al., 2014), and cosmetics (Pengon et a., 2018; Sungpud et al., 2020), as well as other industrial products by high or low acidic treatment, and/or strong heating (Syaputri et al., 2021a). There are three ways to make virgin coconut oil (VCO) in West Sumatra, with heating being the most common method, involving incubating the coconut milk for 12 h at room temperature and then heating for ±6 h to get the oil, with the others being, enzymatic processes, and fermentation. During the process of heating, the coconut milk is continuously stirred until the oil and dreg is separated, forming two layers. This is performed at a fixed temperature or lightly heating to remove the moisture and prevent the rancidity of the oil. However, the chemical compounds are broken and oxidative stress is increased during the heating process (Alves et al., 2015). Meanwhile, long-term oxidative stress conditions potentially lead to serious damage to the human body (Vatner et al., 2020). The other method is by enzymatic processes using bromelain enzyme which breaks down the protein layers of coconut emulsion to separate them from the water and purify the oil by activated carbon adsorbent (Palilingan, 2015), but this method will increase the additional production cost to produce coconut oil.
The best method, which involves a microbial starter which plays a role in the breaking of milk emulsion (Handayani et al., 2008), is the fermentation process by lactic acid bacteria (LAB), which does not involve high temperatures and chemical solvents, hence, a distinctive coconut taste and smell are produced. In addition, the fermentation process can improve the safety and quality of coconut oil, because LAB produces antimicrobial agents against foodborne pathogens, thereby extending their shelf-life (Costa et al., 2020). Suryani et al. (2014) reported that Lactiplantibacillus plantarum (Lpb. plantarum) formerly known as Lactobacillus plantarum (Zheng et al., 2020) was identified in coconut milk. Lactobacillus bulgaricus which is used as a starter to produce coconut oil was also reported to effectively increase lauric acid (42.95%), moisture content, free fatty acids, acid, and peroxide values than Aspergillus oryzae, Candida rugosa, and Saccharomyces cerevisiae in coconut oil (Handayani et al., 2008).
While coconut oil can be converted into various products because it is subjected to processes that involve changes in acidity and salinity (Syaputri et al., 2021a), during fermentation, LAB produces lactic and acetic acids, ethanol, hydrogen peroxide, as well as bacteriocins, each with antimicrobial activity (Syaputri and Iwahashi, 2020). With these environmental stresses; such as pH, temperature and salinity, causing changes to the components involved in the growth process of LAB, this research was conducted to confirm whether LAB present in VCO can be used as a bio-preservative in the product, even under conditions of stress. It also aims to explore the antibacterial activity of LAB under selective environmental stresses and the expected usefulness to improve coconut oil quality.
2. Material and methods
Fermented VCO was obtained from Padang, West Sumatra, Indonesia. One mL of VCO was diluted into 9 mL of Man, Rogosa, and Sharpe (MRS) broth (Becton, Dickinson and Company, Franklin Lakes NJ, USA) until 10−7 and plated on MRS agar (Becton, Dickinson and Company, Franklin Lakes NJ, USA) at 37°C for 24 h under anaerobic conditions. The colonies presented as milky-white in color, circular, and convex were identified as LAB, isolated, and plated on MRS agar. The colonies indicated as LAB were selected and identified as Lpb. plantarum which has been previously studied (Syaputri et al., 2021a) and Latilactobacillus sakei. The bacteria were then kept at −80°C in MRS broth with glycerol 20% (Wako Pure Chemical Industries, Osaka, Japan). The bacterial growth analysis was measured at λ=600 nm using spectrophotometry (Molecular Device SpectraMax M5, San Jose, US).
L. sakei was grown in MRS broth at 37°C for 18 hours, the biomass of the cell was calculated as 8 log CFU/mL, and L. sakei genomic DNA was extracted using the Extrap Soil DNA Kit Plus Ver.2. (Nippon Steel Eco-Tech Corporation, Tokyo, Japan), according to the manufacturer’s protocol. The quality of the extracted DNA was assessed by gel electrophoresis using a 1% agarose gel in 1× TAE buffer. The gel was stained by ethidium bromide and photographed under ultraviolet light.
The 16S rRNA gene was used to identify L. sakei and amplified using a pair of universal primers 27 F: (5'-GAGTTTGATCCTGGCTAG-3') and 1525 R: (5'-AGAAAGGAGGTGATCCAGCC-3') (Syaputri et al., 2021b). The reaction of polymerase chain reaction (PCR) was carried on in a fast reaction tube (Applied Biosystems, Waltham, US) containing 12.5 μL 2× Green Master Mix PCR (Promega, Madison, USA), 1.25 μL of 0.05 pmol/μL of each primer, 9 μL nuclease-free deionized water (Promega, Madison, USA), and 1 μL template DNA. The PCR program was run following the temperature program, namely initial denaturation of DNA for 5 min at 95°C, 25 cycles of 1 min at 94°C, 1 min at 56°C, 1.5 min at 72°C, and final extension for 7 min at 72°C. The product was analyzed by electrophoresis (Mupid-exU, Shiga, Japan) using 1% (w/v) agarose gel in TAE 1× buffer at 100 V for 30 min. The size of the DNA fragments was estimated using a FastGene 100 bp DNA Ladder (Nippon Genetics, Düren, Germany) to detect the presence of 1,500 bp band. The Fast GeneTM Gel/PCR Extraction kit (Nippon Genetics, Düren, Germany) was used for purification before sending the extracted DNA for sequencing. The purification of PCR result was sequenced by DNA Sequencing Analytical service by the Division of Genomic Research, Gifu University using the Multicapillary DNA Sequencer ABI Prism 3100/3130 Genetic Analyzer (Thermo Fisher Scientific, Waltham, USA). The sequences were edited and assembled into contiguous sequences (contigs) using BioEdit and were aligned using the Bioedit-ClustalW Multiple Alignment. The sequence was read and compared against the NCBI database using BLAST (http://blast.ncbi.nlm.nih.gov/Blast.cgi). Isolates with 97% or higher similarity in sequences were identified as the same species (Johnson et al., 2019).
The antibacterial activities were estimated using the wells diffusion method (Syaputri et al., 2021a) and the results were evaluated based on the presence of a clear halo surrounding the wells. L. sakei was grown under selective environmental stresses, with pH 3.0, 4.5, 7.0, and 9.0 and at 37°C in MRS broth. With adjustments carried out using 5 mol/L NaOH and 5 mol/L of HCl (Wako Pure Chemical Industries, Osaka, Japan) to increase and reduce the pH, respectively, all the cells are harvested at the beginning of the stationary phase. The samples were filtered through a 0.20 μm pore size filter (Advantec Toyo Roshi Kaisha Ltd., Tokyo, Japan) and incubated at temperatures of 60, 70, 80, 90, and 100°C for 30 min. The indicator bacteria (Escherichia coli K12 JM109, Bacillus subtilis, and Staphylococcus aureus JCM 20624) were incubated at 30°C for 12 hours in Luria Bertani broth (Becton, Dickinson and Company, Franklin Lakes, NJ, USA). The wells were cut on Mueller-Hinton agar (Becton, Dickinson and Company, Franklin Lakes, NJ, USA) and impregnated with 100 μL cell-free supernatant (CFS) of L. sakei. Sodium ampicillin (Avantor, US) 100 μg/mL was used as a positive control. Isolates that produced clear zones, indicating growth inhibition were considered positive, with the plates being incubated at 30°C for 24 hours to assess antibacterial activity as halo formation.
All the experiments were repeated three times to make sure the data was suitable for statistical analysis. All the data expressed as the mean±standard error of the mean and the significant differences were analyzed by one-way of variance (ANOVA) followed Tukey’s post hoc test using RStudio version 4.1.3 (Northern Ave, Boston, USA) suitable for windows 10. p⟨0.05 was considered as significantly different.
3. Results and discussion
LAB was isolated from fermented VCO with a total bacterial load of 3.4×108 CFU/mL. The morphology of the MRS agar plate was presented as milky-white in color, circular, and convex (Astuti, 2016). Two species were identified as Lpb. plantarum and L. sakei, while the 16S rRNA specific for LAB was amplified using universal primers to confirm the species and the result showed a length of 1,500 bp as demonstrated in Fig. 1. The PCR result indicated that the band is clear enough without smear.
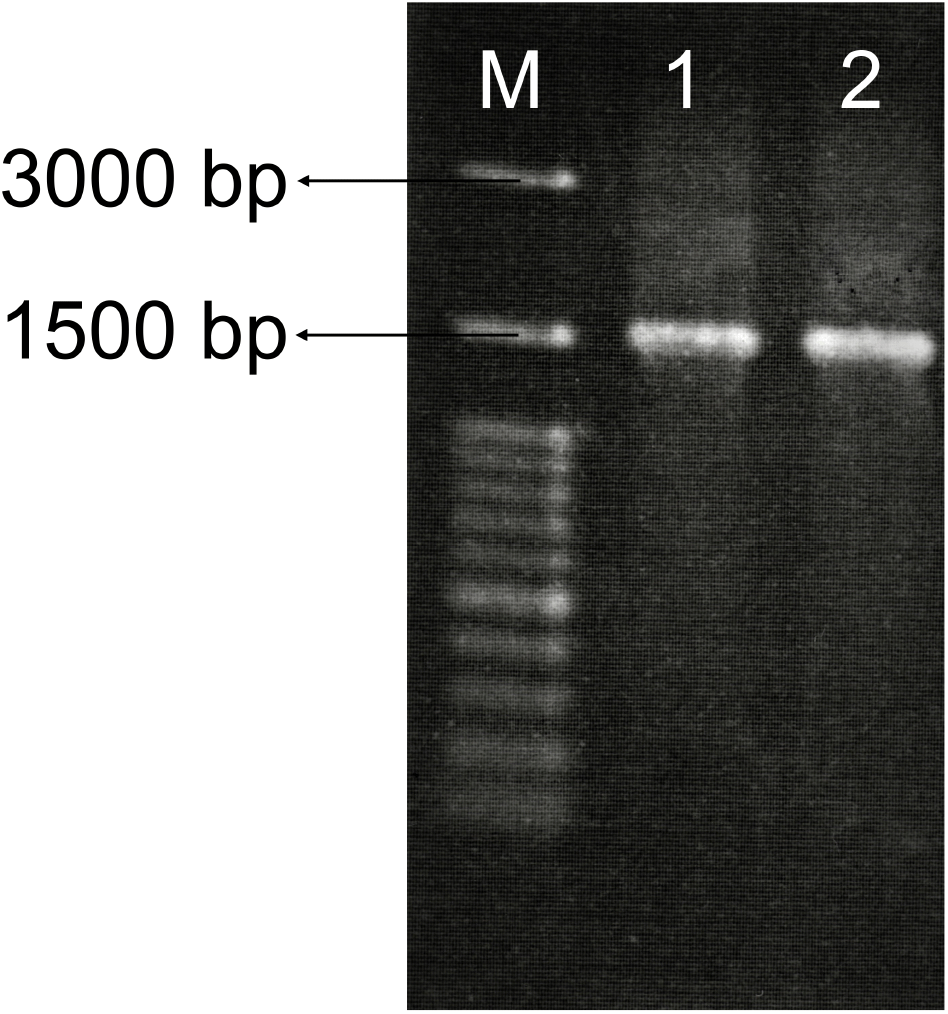
A total of 10 closely related species were selected to make the phylogenetic tree based on their genetic similarities and differences (Fig. 2), where the PCR result was purified and sequenced to discover the species, then the sequence was read and compared against the NCBI database using BLAST. A phylogenetic tree of L. sakei based on 16S rRNA sequence analyses was constructed using MegaX Construct/Test Neighbor-Joining Tree which showed the placement of representative strains. The candidate sequenced shared 99% similarity with Latilactobacillus sakei.
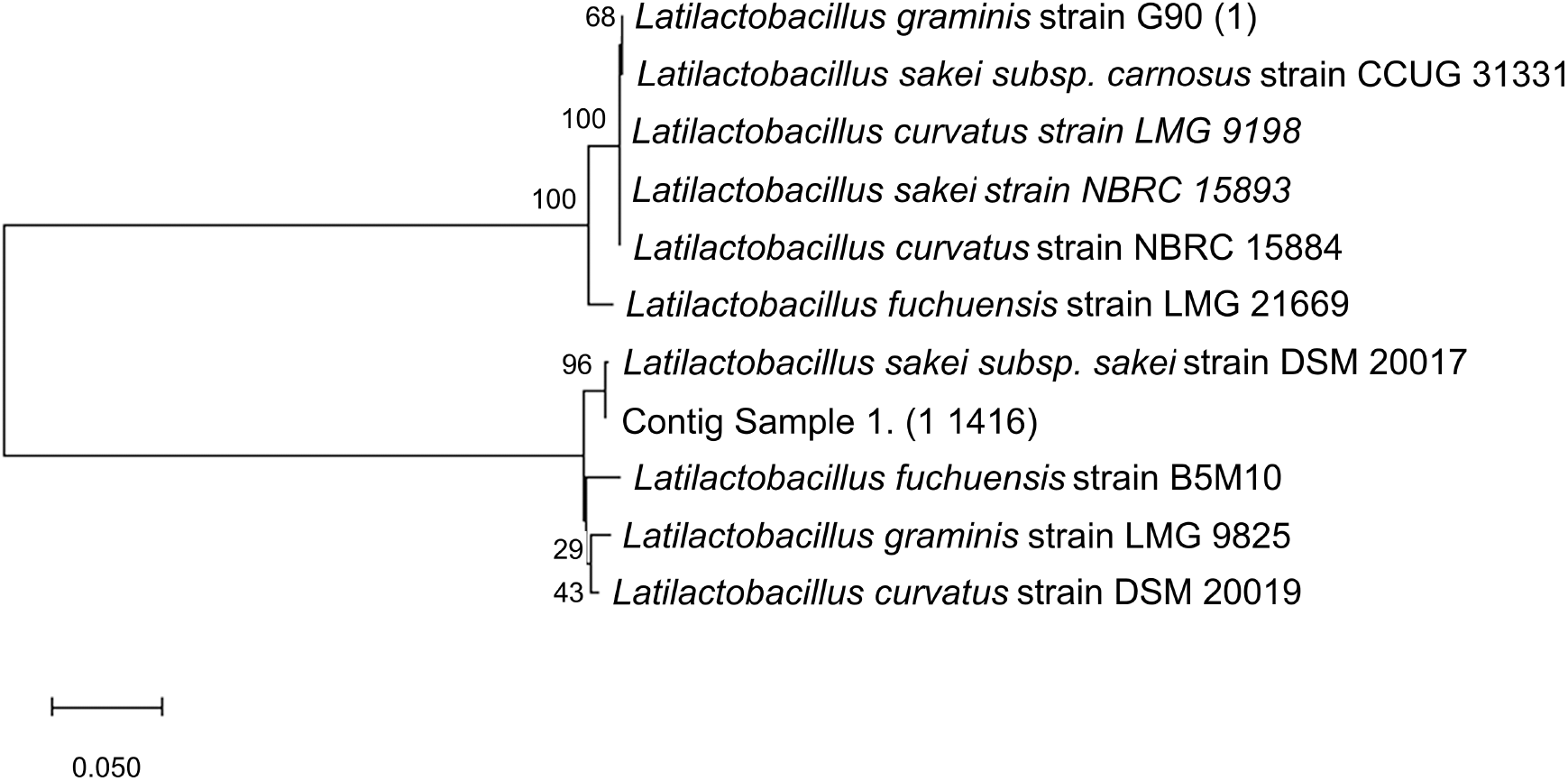
The bootstrap value on the phylogenetic tree of L. sakei isolated from VCO and L. sakei subsp. sakei strain DSM 20017 with accession number NR_042443.1 was 96%. With bootstrap confidence intervals usually being estimated, specifically for complex data structures, as they are a fundamentally more ambitious measure of accuracy than a simple standard error (Efron et al., 1996), there is a computer-based technique for assessing the accuracy of almost any statistical estimate. The phylogenetic tree was built using a cut-off value of <95%, and it is considered that the resampling is well supported.
L. sakei is commonly isolated from fermented foods (Janßen et al., 2020), animal faces (Li et al., 2020), fresh meat products (Zagorec and Champomier-Vergès, 2017), and sake breweries (Takahashi et al., 2021). It is reportedly related to L. curvatus, L. fuchuensis, and L. graminis (Zagorec and Champomier-Vergès, 2017). Furthermore, Zagorec and Champomier-Vergès (2017) stated that other methods to identify LAB include using rpoB genes. L. sakei subsp. carnosus and L. sakei subsp. sakei have been clarified as two subspecies based on Random Amplified Polymorphic DNA (RAPD) and phenotypic characterization. Another identification method, revealing the several clusters within L. sakei (Li et al., 2020), is using strain-specific genomic markers or MultiLocus Sequence Typing (MLST). Based on the sequenced data from 16S rRNA specific primer for LAB, the isolated sample from coconut oil is L. sakei subsp. sakei.
L. sakei is a psychotropic lactic acid bacterium, previously classified in the genus Lactobacillus, and has potential for bio-preservation (Ohta-Shimizu et al., 2021). It resists harsh conditions during preservation, such as high salt concentration, low water activity, low temperature, and pH (McLeod et al., 2010). The growth analysis was measured to determine the stationary phase of the cell. Based on the results, the optimum growth under environmental stresses was at pH 9, followed by the sample without treatment, as well as pH 7 and 4.5 but no growth was observed at 3.0. L. sakei under environmental stresses were harvested in the stationary phase due to the production of maximum secondary metabolites (Barbieri et al., 2020). LAB is reported to have antimicrobial activity, which is produced at the beginning of the stationary phase (Saranraj et al., 2013). The cells of the samples without treatment and under environmental stresses of pH 3, 4.5, 7.0, and 9.0 were harvested at 12, 32, 28, 12, and 8 hours, respectively, to determine the antimicrobial activity (shown in Fig. 3). Antimicrobial activity is required by L. sakei as a bio-preservative of VCO.
The result indicated that the bacterial growth was influenced by the pH value, and this is consistent with Takahashi et al. (2021) which reported that L. sakei isolated from sake breweries was unable to grow at pH below 3.9, and the growth analysis was influenced by the strain, pH, specific nutrients, and temperature. While this bacterium, L. sakei, has a contribution to the taste of sake, such as the umami taste (Takahashi et al., 2021), previous research also found that L. sakei can grow optimally at pH 4.1-6.5 at temperatures of 4°C-20°C (Costa et al., 2020; Takahashi et al., 2021), hence, an analysis was carried out on whether L. sakei has a contribution to the taste of coconut oil.
The antimicrobial activity of L. sakei was measured under environmental stresses conditions, specifically temperature and pH, considering the product manufacturing process as a bio-preservative. The growth procedure was performed under selective environmental stresses, at pH 3.0, 4.5, 7.0, and 9.0 at 37°C on MRS broth, while sodium ampicillin 100 μg/mL was used as a positive control. The results showed that the antimicrobial activity under environmental stresses (pH) had a significant on the inhibition zone with p⟨0.05. The environmental stresses conditions (pH) and indicator bacteria were significantly different on antimicrobial activities. The antimicrobial activity of L. sakei against E. coli K12 JM109 was more effective in the sample without treatment, followed by pH at 4.5 and 7.0, 9.0, and 3.0. Moreover, whereas L. sakei did not show any antimicrobial activity against S. aureus JCM 20624 at pH 3.0, and better inhibition was found against S. aureus JCM 20624 than sodium ampicillin 100 μg/mL, the antimicrobial activity against B. subtilis was more effective at pH 4.5, followed by 7.0, the sample without treatment, 9.0, and 3.0, while the activity against S. aureus JCM 20624 was significantly effective in the without treatment sample, followed by pH 7.0, 4.5, and 9.0 as shown in Fig. 4.
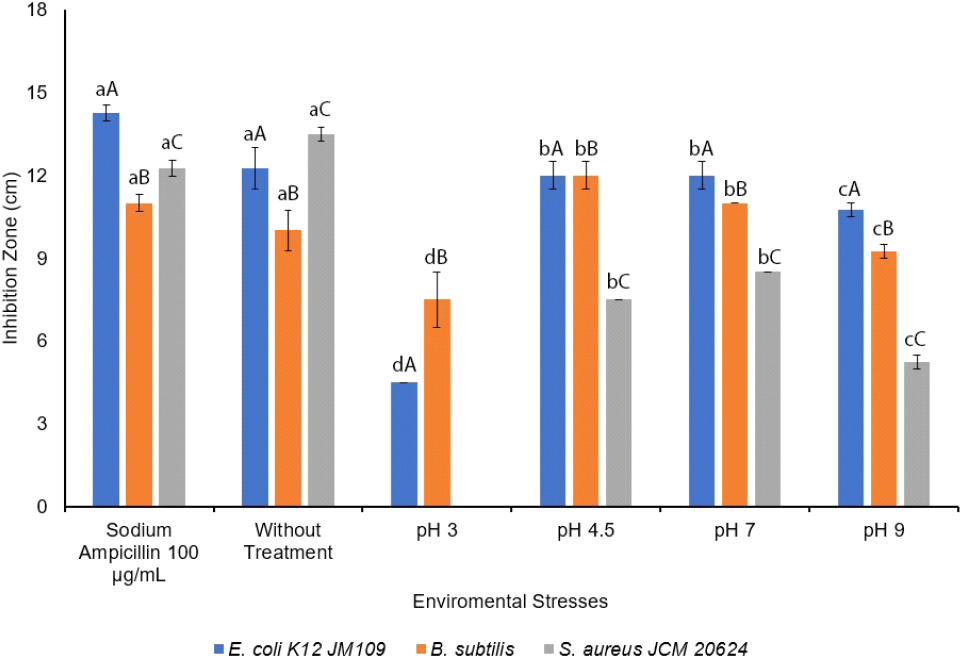
Although the growth analysis of L. sakei was optimum under pH 9.0, the antibacterial effect was optimum at pH 4.5-7.0 against E. coli K12 JM109, B. subtilis, and S. aureus JCM 20624. With L. sakei being reported to inhibit Listeria monocytogenes (Abitayeva et al., 2021; Costa et al., 2020), Vibrio sp. (Joffraud et al., 2006), S. aureus, Serratia marcescens, E. coli (Abitayeva et al., 2021), and Salmonella typhimurium SL1344 (Kim et al., 2021), Huh and Hwang (2016) reported that L. sakei subsp. ALI033 has antifungal activity and is unstable at high pH levels while antifungal activities were not detected at pH 5.0-8.0 (Huh and Hwang, 2016).
The antimicrobial activity was measured under high temperatures namely 60, 70, 80, 90, 100°C, and the results showed no significant difference with p⟨0.05 (Fig. 5). The results showed that the antimicrobial activities of L. sakei against E. coli K12 JM109 at 60, 70, 80, 90, 100°C are 8.5±0 mm with n=3. Moreover, the antimicrobial activity against B. subtilis and S. aureus JCM 20624 were more effective at without temperature treatment, followed at 60°C and 90°C, at 100°C, and 70°C and 80°C. Slavica et al. (2010) reported that L. sakei I 154 possess thermal resistance. Furthermore, L. sakei produced antilisteria effect at 65, 80, 90, and 100°C, but the activity decreased at 100°C after 10, 30, and 60 minutes of treatment (Slavica et al., 2010). As L. sakei isolated from VCO can be used as a natural antimicrobial agent to extend coconut oil’s shelf-life, this bacterium has also been reported to produce organic acids such as lactic (Takahashi et al., 2021), citric, acetic, malic, and tartaric acids (Huh and Hwang, 2016), as well as hydrogen peroxide (Huh and Hwang, 2016), CO2, diacetyl, acetaldehyde, amino acids (Zagorec and Champomier-Vergès, 2017), and bacteriocins (sakacin) (Musatti et al., 2020).
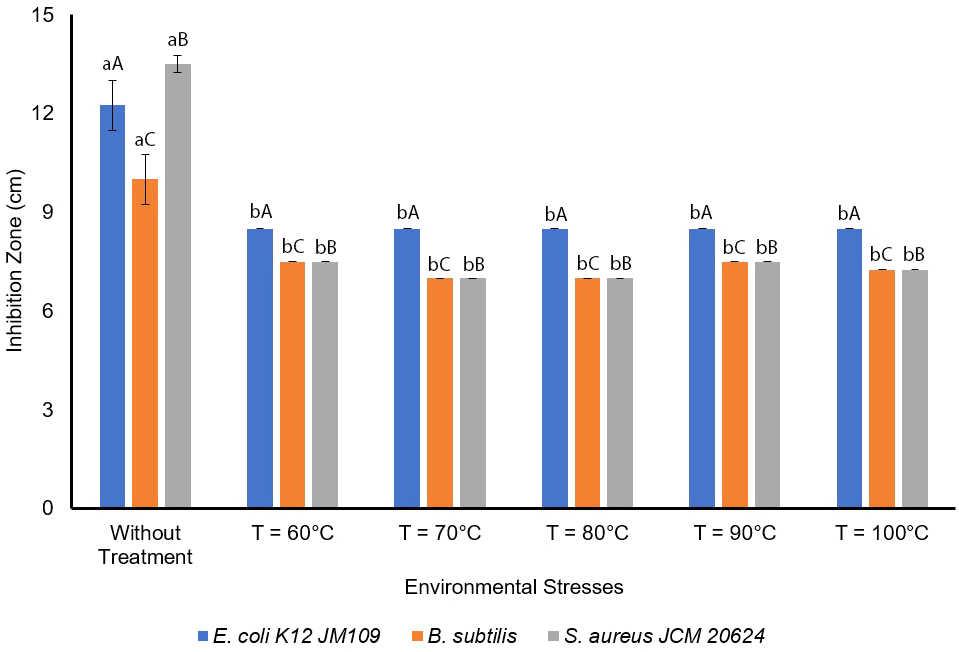
4. Conclusion
We concluded that LAB isolated from VCO was 99% similar to L. sakei. The growth analysis was influenced by pH and the optimum rate was pH 9.0. However, the antibacterial activities were optimum at pH 4.5-7.0, and even under high temperatures of 60, 70, 80, 90, and 100°C, the CFS of L. sakei still gave antimicrobial activity.