1. Introduction
Cannabis sativa is an annual plant belonging to the Cannabinaceae family. It was first cultivated in approximately 1st century AD. Although its origin is known to be Central Asia, it was mainly cultivated in Canada and China. C. sativa is distinguished into hemp and marijuana depending on the content of δ-9-tetrahydrocannabinol (THC), a psychotropic substance. As opposed to marijuana, the THC content in hemp is 0.3% or less, whereas the content of cannabidiol (CBD), which inhibits THC’s hallucinogenic effects, is high. Moreover, research has suggested that hemp has neuroprotective, anti-inflammatory, and antirheumatic effects (Jeong et al., 2014; Maroon et al., 2018; Sohn et al., 2021; Yoon et al., 2018).
Hemp seeds contain approximately 25% of proteins with a good balance of essential amino acids and are abundant in unsaturated fatty acids, mineral nutrients, and fiber (Bartkiene et al., 2016; Noelia et al., 2020). Research on hemp seeds has focused mainly on CBD or the nutritional components of the seed itself. In the Gyeongbuk hemp regulation free zone in Gyeongsangbuk-do, the industrialization of products containing CBD for medical purposes associated with epilepsy, dementia, and nerve disorders is studied (Sohn et al., 2021).
Mitochondria generate reactive oxygen species (ROS), which act as signal transmission substances within a cell. However, high levels of ROS cause various chronic and degenerative diseases and promote aging because it non-selectively and irreversibly destroys cell components such as protein, lipid, DNA, etc. Therefore, antioxidant materials that eliminate ROS are utilized as health functional food (Yoo et al., 2005). Biologically active peptides (Cho et al., 2019) that act as natural antioxidants can be obtained by hydrolyzing various high-protein vegetable raw materials and animal food such as milk, egg, fish, insects, etc. (Jung et al., 2009). Biologically active peptides are oligopeptides mainly composed of 2-20 amino acids and, thus, are easily absorbed into the body owing to their low molecular weight (Cho et al., 2019; Yu et al., 2017). Moreover, biologically active peptides have been reported to exhibit anti-diabetic, antihypertensive, anticarcinogenic, antithrombotic, and antioxidant activities, as well as relieve pain and improve immune function (Cho et al., 2019; Park et al., 2018; Seol et al., 2012; Yoo et al., 2013). However, there is a lack of research on the preparation of hydrolyzed protein using hemp seeds and their biological activities.
Therefore, this study aimed to prepare protein hydrolysates containing biologically active peptides from hemp seeds via enzymatic hydrolysis of defatted hemp seeds, a by-product of hemp seed oil production. The antioxidant activity of defatted hemp seed protein hydrolysates (HPHs) was also analyzed and compared to provide preliminary data for natural antioxidant material development.
2. Materials and methods
The defatted hemp seed powder used in this experiment was purchased from Nutiva (Richmond, CA, USA), and the defatted soybean powder was purchased from Jungan-ri Herbal Village Cooperative (Yangpyeong-gun, Gyeonggi-do, Korea) and stored in a −20°C freezer. Proteases alcalase, bromelain, flavourzyme, neutrase, and papain were purchased from Daejong Co. Ltd. (Seoul, Korea) among Novo (Seoul, Korea) products. The 2,4,6-trinitrobenzene sulfonic acid (TNBS) used to measure the degree of hydrolysis of protein was purchased from G-Bio-Sciences (St. Louis, MO, USA), and sodium dodecyl sulfate (SDS) was purchased from Wako (Osaka, Japan). Tetramethylethlyenediamine (TEMED) and 30% acrylamide/bis solution (29:1) were purchased from Bio-Rad (Hercules, CA, USA). Other reagents and solvents were purchased from Sigma-Aldrich Co. (St. Louis, MO, USA).
Defatted hemp seed powder was suspended in distilled water and prepared into a 4% (w/v) substrate solution. The solution was heated for 20 min at 90°C to inactivate the enzyme and then allowed to cool down. Then, each protease (alcalase, bromelain, flavourzyme, neutrase, and papain) at a concentration of 1% (w/w) with respect to the substrate was added to the solution. Next, hydrolysis was performed for 12 h at 100 rpm and 55°C, followed by heating for 20 min at 90°C to inactivate the enzymes. After cooling, the hydrolysates underwent centrifugation for 20 min at 13,000 ×g, following which the supernatants were filtered with a cell strainer and then subjected to decompression filtration using Whatman filter No. 3. Finally, the samples were centrifuged for 1–2 h at 5,000 ×g with a membrane filter (Amicon Ultra-15, EMD Millipore Corporation, Billerica, MA, USA) to obtain protein hydrolysates of 3 kDa or below. The protein hydrolysates were lyophilized for 72 h and stored at 20°C until further use. The yield of hydrolysates was represented as the weight of the lyophilized protein hydrolysates as % (w/w) with respect to the defatted hemp seed powder weight used to prepare the hydrolysates. Defatted soybean protein hydrolysate was prepared with alcalase using the same method as described above.
Using the TNBS method, the degree of hydrolysis of HPHs was measured according to the processing time of each enzyme preparation (Choi et al., 2013). Each hydrolysate was centrifuged for 10 min at 4°C and 3,000 ×g, and the supernatant was used to measure the degree of hydrolysis. By mixing (v/v) 10 μL of 1% TNBS and 990 μL of 100 mM sodium bicarbonate (pH 8.5), 0.01% TNBS was prepared. Next, 50 μL of TNBS and 100 μL of the reaction mixture of each enzyme preparation were mixed and allowed to react with each other for 2 h in a 37°C incubator, following which 1 N HCl and 10% SDS were added to stop the reaction. Finally, a microplate spectrophotometer (Bio Tek Instruments Inc., Winooski, VT, USA) was used to measure the absorbance at 335 nm wavelength. In this study, the available amino group concentration (μg/mL) was obtained from the standard curve drawn using tyrosine.
To examine the protein hydrolysis characteristics of defatted hemp seed powder, SDS-PAGE was used to characterize the proteins within the hydrolysates. SDS-PAGE was performed using 15% polyacrylamide gel, and 24 μL of the supernatant was obtained after subjecting each hydrolysate to centrifugation for 10 min at 4°C, and 3,000 ×g was loaded onto the gel. The samples underwent electrophoresis for 2 h at 80 V. Proteins were stained using 0.1% coomassie brilliant blue, and bleaching was performed using 30% methanol containing 10% acetic acid. Finally, Gel Logic 2200 PRO Imaging System (Carestream Health Inc., Rochester, NY, USA) was used to visualize the polyacrylamide gel. A Bio-Rad Laboratories (Carlsbad, CA, USA) product was used as the molecular weight marker.
The Folins-Denis method was used to measure the total polyphenol contents. In a 96-well plate, 60 μL of the hydrolysate sample and 60 μL of Folin-Ciocalteu phenol reagent that was diluted two times were added and left unattended for 3 min. Next, 60 μL of 10% sodium carbonate (Na2CO3) was added to the sample and allowed to react for 1 h. Finally, the absorbance at 700 nm wavelength was measured using a microplate spectrophotometer. The total polyphenol compound content was obtained by acquiring standard curve drawn using gallic acid and tannic acid, and were presented as gallic acid equivalent (GAE; μg GAE/mg) and tannic acid equivalent (TAE; μg TAE/mg), respectively.
The ABTS radical scavenging activity was measured according to the method described by Yu et al. (2017). Potassium persulfate with a final concentration of 2.45 mM and 7 mM ABTS were mixed and left unattended for 12-16 h at room temperature in a dark room to form radicals. The solution was diluted with phosphate buffered saline (PBS, pH 7.4) to ensure that the absorbance of the mixed solution at 734 nm wavelength is 0.70±0.02 before use. In a 96-well late, 20 μL of the hydrolysate sample and 180 μL of diluted ABTS solution were added and left unattended for 1 min. Next, absorbance was measured at 734 nm wavelength using a microplate spectrophotometer. The measurement was presented as the RC50 value, that is, the concentration of the hydrolysate sample required for disrupting the radical by 50%. Trolox was used as the positive control and the antioxidant activity of the hydrolysate sample was calculated and presented as trolox equivalent (TE; μg TE/mg).
The H2O2 scavenging activity was measured according to the method described by Yu et al. (2017). In a 96-well plate, 20 μL of hydrolysate sample diluted by each concentration, 100 μL of PBS, and 20 μL of 1 mM H2O2 were added and allowed to react with each other for 5 min in a 37°C incubator. Next, 30 μL of 1.25 mM ABTS and 30 μL of 1 U/mL peroxidase were added and allowed to react with each other in a 37°C incubator. Finally, the absorbance at 405 nm wavelength was measured using a microplate spectrophotometer. The measurements were presented as RC50 values, that is, concentrations required for reducing 50% of the absorbance. Trolox was used as the positive control to present the standard curve, and the antioxidant activity of the hydrolysate sample was calculated and presented in μg TE/mg.
Inhibitory effect on 2-Deoxy-D-ribose oxidation was measured by modifying the method described by Chung (1997). To the solution containing 100 μL of 10 mM 2-Deoxy-D-ribose, 10 mM FeSO4 · 7H2O, and 10 mM ethylene-diamine-tetraacetic acid (EDTA) each, 100 μL of hydrolysate samples that were diluted by each concentration, 500 μL of 0.1 M potassium phosphate buffer (pH 7.4), and 100 μL of 10 mM H2O2 were added until the final reaction solution reached a volume of 1 mL. Next, the solution was incubated at 37°C for 4 h. Following incubation, 50 μL of 10% trichloroacetic acid (TCA, w/v) was added to 100 μL of the reaction solution and allowed to react for 10 min at 95°C. Finally, the solution was cooled, and 100 μL of the supernatant was used to measure the absorbance at 532 nm wavelength using a microplate spectrophotometer. Butylated hydroxyanisole (BHA) was used as the positive control.
To measure the inhibitory effect on linoleic acid oxidation, 1 mL absolute alcohol, 1 mL 5% linoleic acid, 2 mL 50 mM sodium phosphate buffer (pH 7.0), and 1 mL of the hydrolysate sample were added, mixed, and heated at 40°C and 100 rpm. At one-day intervals, thiobarbituric acid (TBA) was measured to identify the linoleic acid oxidation. Next, 50 μL of linoleic acid reaction substrate solution, 100 μL of 0.8% TBA (w/v), and 100 μL of 20% TCA (w/v) were added, mixed, and allowed to react for 20 min at 95°C or above. The reacted solution was centrifuged for 15 min at 1,850 ×g and 20°C. Finally, 180 μL of the supernatant was extracted, and its absorbance was measured at 532 nm. Trolox was used as the positive control.
All experiments were repeated at least three times, and the results were presented as the mean and standard deviation (mean±SD). SPSS Statistics 26 software (Statistical Package for the Social Science, Version 26.0, SPSS Inc., Chicago, IL, USA) was used for statistical analysis. In addition, one-way ANOVA was used to determine statistically significant differences between groups, and Duncan’s multiple range test was performed as a post hoc test. Statistically significance was set at p<0.05.
3. Results and discussion
Protein hydrolysates were prepared from defatted hemp seed powder using five different proteases, namely bromelain, papain, alcalase, flavourzyme, and neutrase.
When proteins are hydrolyzed, the available amino group concentration increases in proportion to the degree of hydrolysis, and this phenomenon can be used as an index to represent the degree of hydrolysis (Choi et al., 2013). Thus, the degree of hydrolysis of each enzyme according to the processing time was measured using a TNBS assay with tyrosine as an indicator substance. The concentration of the reacted available amino group for 24 h was 9.40 mg/mL in the flavourzyme group, followed by 4.56 mg/mL in the alcalase group, 3.78 mg/mL in the neutrase group, 3.21 mg/mL in the bromelain group, 1.50 mg/mL in the papain group, and 0.58 mg/mL in the non-enzyme group (Fig. 1). In the case of the flavourzyme group, the degree of hydrolysis was the highest, and while their available amino group concentration increased steadily up to 24 h, the remaining enzyme groups showed a relatively lower increase. At 8 and 12 h time elapse, no significant differences were found in the degree of hydrolysis among all enzyme groups at 24 h. Thus, it was inferred that 8-12 h was the appropriate duration for enzyme treatment for the preparation of defatted hemp seed protein hydrolysates.
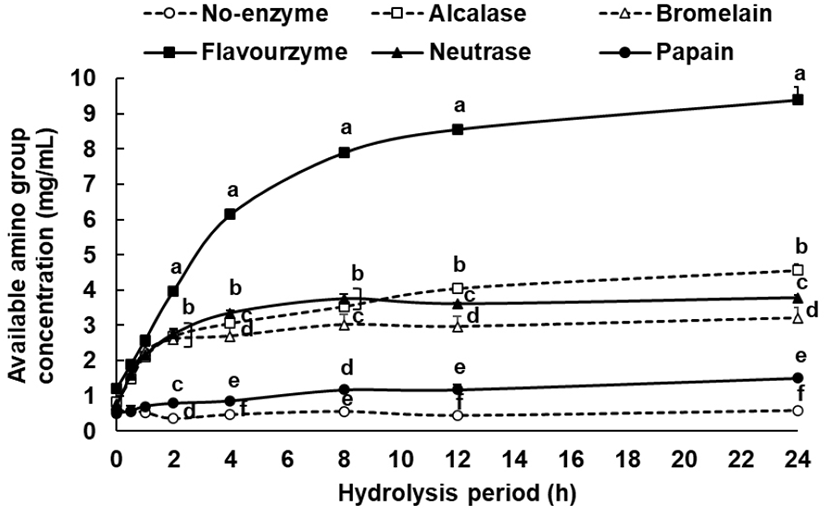
In addition, SDS-PAGE was performed to identify the hydrolysis characteristics of HPHs, and the results are shown in Fig. 2. When comparing with the non-enzyme group, proteins of 20 kDa or above did not show overall in enzyme groups, and proteins were decomposed to 20 kDa or below. In particular, protein bands of 10 kDa or above were nearly not observed with protease flavourzyme, and peptides of approximately 10 kDa identified in other enzyme groups were not observed. Thus, it was assumed that proteins were decomposed to peptides with smaller molecular weights. Based on these results, it was concluded that protein hydrolysis was successful after 12 h, similar to the results of TNBS, and the hydrolysis efficiency of the flavourzyme group was greater than that of other enzyme groups.
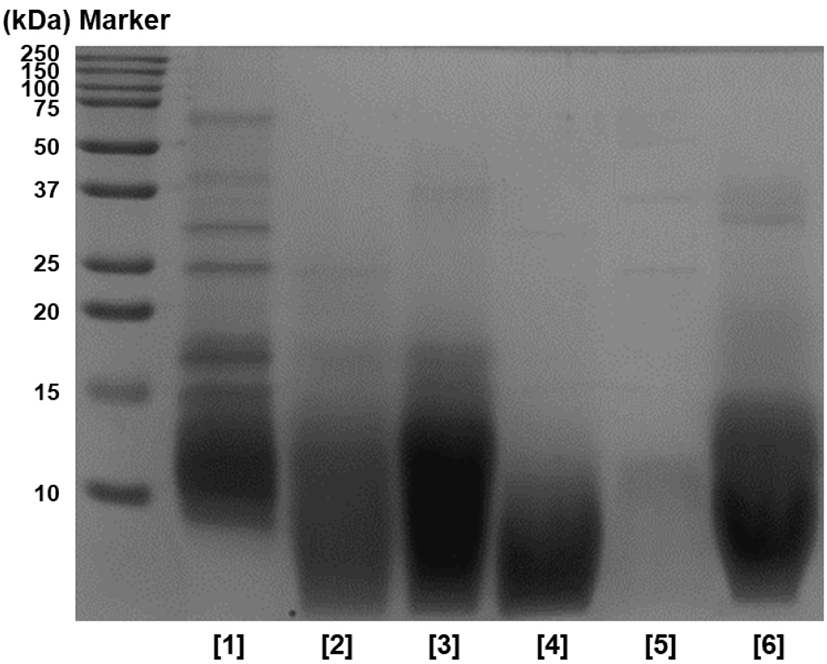
Table 1 presents the yield of the protein hydrolysates prepared using each enzyme. The yield of the protein hydrolysate prepared using flavourzyme was the greatest at 24.30%, followed by that prepared using neutrase at 22.75%, bromelain at 20.65%, and alcalase at 17.35%. Thus, the enzyme groups showed a greater yield than the non-enzyme group. However, the yield of papain group was 11.75%, which suggested a low protein hydrolysate preparation yield that was not significantly different from the yield of the non-enzyme group at 7.64%. The flavourzyme group was found to contain smaller peptide molecules, and this result was highly correlated to the results of the degree of hydrolysis (Figs. 1 and 2).
Hydrolysates | Yields of protein hydrolysates (%)1) |
---|---|
No enzyme | 7.64±0.03b2) |
Alcalase | 17.83±0.07ab |
Bromelain | 20.65±0.05a |
Flavourzyme | 24.30±0.05a |
Neutrase | 22.75±0.12a |
Papain | 11.57±0.05ab |
Tang et al. (2000) found that among alcalase, flavourzyme, and neutrase groups, alcalase showed the highest degree of hydrolysis at 4 h when only the defatted hemp seed protein was separated for hydrolysis. However, the radical scavenging activity was outstanding in the neutrase group, and a higher radical scavenging activity was shown in the 4 h hydrolysate than in the 2 h hydrolysate. When comparing with these results, the flavourzyme group at 4 h showed the highest degree of hydrolysis when defatted hemp seed that was not subjected to protein separation was hydrolyzed, and rapid hydrolysis occurred even after 4 h (Fig. 1). Therefore, the optimal hydrolysis duration was set to 12 h in this study to prepare each hydrolysate and perform the antioxidation experiment. The results showed that the radical scavenging activity was highest in the alcalase group, which differed from the results of Tang et al. (2009). This was attributed to the preprocessing condition of the defatted hemp seed as well as the difference in peptide and amino acid generated according to the duration of hydrolysis, which resulted in the difference in antioxidizing effect.
Before examining the antioxidation activation of the defatted hemp seed protein hydrolysates of each enzyme, the total polyphenol contents was measured based on gallic acid and tannic acid as base substances, and the results are shown in Table 2. The total polyphenol contents was converted to the GAE value. The non-enzyme group showed 10.09 GAE/mg, whereas the enzyme groups showed 13.09-17.40 GAE/mg. Thus, the total polyphenol content of all enzyme groups was measured to be significantly higher than the non-enzyme group. In all the enzyme groups, alcalase, bromelain, and neutrase treatment groups showed relatively high polyphenol content, and the total polyphenol contents converted to the TAE value was also similar to the GAE value.
According to Kim et al. (2019), the increase in total polyphenol contents of defatted rice bran according to the degree of protein hydrolysis was reported to be attributed to the bound polyphenol existing in combination with macromolecules such as protein converted to lower-weight-molecules by proteases. Thus, bound polyphenols are transformed into free polyphenols, and the measurement value for total polyphenol content increases. Therefore, it was speculated that the total polyphenol content would be the greatest in flavourenzyme according to the measurement results of the degree of hydrolysis of the defatted hemp seed protein hydrolysates, but the total polyphenol contents of flavourenzyme protein hydrolysates was measured to be lower than that of alcalase, bromelain, and neutrase protein hydrolysates, indicating that the free polyphenol content can be relatively high despite a low degree of hydrolysis and that the correlation between the change in free polyphenol content and the degree of protein hydrolysis is not high.
When ABTS and potassium persulfate are mixed to react in a dark room, a blue-green radical is generated, and the antioxidant activity of the hydrolysate sample can be measured based on the bleaching reaction that occurs as this ABTS radical is scavenged by antioxidizing substances (Park et al., 2017; Suh et al., 2019).
The experimental results were presented as RC50, that is, the protein hydrolysate concentration necessary for scavenging 50% ABTS radical, and as TEAC, that is, the converted value of the antioxidant activity of the hydrolysate sample with trolox as the base substance. The RC50 value for the ABTS radical was lowest at 56.95 μg/mL in the alcalase group, followed by 60.83 μg/mL in the papain group, 61.53 μg/mL in the neutrase group, 63.39 μg/mL in the bromelain group, 65.96 μg/mL in the flavourzyme group, and 147.00 μg/mL in the non-enzyme group, and the alcalase group showed the greatest significant scavenging activity (Table 3). In addition, all enzyme groups showed a significantly greater ability to scavenge ABTS radicals than the non-enzyme group. In particular, the alcalase group showed an RC50 value 0.5 times or lower than that of the non-enzyme group. The TEAC value was also highest in the alcalase group, and the flavourzyme group showed relatively low scavenging activity among the enzyme groups.
Meanwhile, the total polyphenol content of neutrase and papain groups that did not show a significant difference in RC50 value was significantly higher in neutrase group than papain group. The total polyphenol contents in flavourzyme and papain groups did not show a difference, but the ABTS radical scavenging activity was found to show a significant difference between the groups (Table 2, 3). These results indicate that the correlation between the ABTS radical scavenging activity of the protein hydrolysates and the total polyphenol contents is low. In addition, the peptide and amino acid generated through hydrolysis partially contributed to the antioxidant activity of the defatted hemp seed protein hydrolysates. Thus, the antioxidant activity of the protein hydrolysates was attributed to various substances including peptides and polyphenol compounds contained in hydrolysates.
The antioxidant activity of HPHs was compared with that of defatted soybean protein hydrolysates, which were reported to have great antioxidant activity among plant-derived protein hydrolysates (Park et al., 2010). The RC50 of the alcalase group of defatted soybean was 84.14 μg/mL; thus, the protein hydrolysates of all defatted hemp seed were found to have relatively higher ABTS radical scavenging activity than the defatted soybean alcalase group excluding the non-enzyme group.
The RC50 value for H2O2 was 90.73 μg/mL for the alcalase group, followed by 98.46 μg/mL for the neutrase group, 107.05 μg/mL for the papain group, 113.34 μg/mL for the bromelain group, 135.39 μg/mL for the flavourzyme group, and 436.41 μg/mL for the non-enzyme group, which suggested that the alcalase group had the greatest H2O2 scavenging activity (Table 4). When the protein hydrolysates of each enzyme and the non-enzyme group were compared, all enzyme groups showed significantly greater H2O2 scavenging activity than the non-enzyme group. When the defatted soybean alcalase group was compared, all enzyme groups showed higher H2O2 scavenging activity than the non-enzyme group. Moreover, the alcalase group had significantly greater H2O2 scavenging activity than the other enzyme groups similar to the ABTS radical scavenging activity. The TEAC value was also most significant in the alcalase group, and the TEAC values of the enzyme groups were found to be about 3.0-4.3 times greater than the TEAC value of the non-enzyme group, so it was found that the antioxidant activity of the defatted hemp seed had significantly increased through the preparation of protein hydrolysates.
This experiment involved measurements that applied the principle of generating malondialdehyde by the oxidation of 2-deoxy-D-ribose with hydroxyl radical which was generated from the reaction of Fe2+ and H2O2 (Duan et al., 2014; Lee et al., 2005).
With BHA as base substance, the 2-deoxy-D-ribose oxidation inhibition rate of the hydrolysate sample was shown in Fig. 3. The results of identifying the oxidation inhibition rate after treating each protein hydrolysates at 0.5-2 mg/mL showed that all enzyme groups showed an inhibition rate between 33.71%-66.62%, which were significantly higher than those exhibited by the non-enzyme group. Meanwhile, some enzyme groups showed relatively higher inhibition rates; however, the inhibition rates of all enzyme groups did not show statistically significant differences.
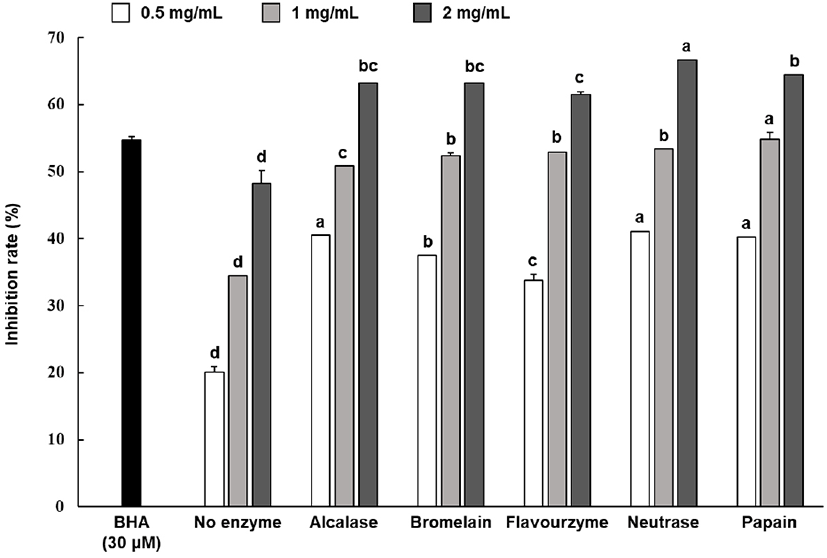
The peroxides that are generated at the onset of lipid oxidation involve the oxidation of Fe2+ to Fe3+ to create a reddish brown pigment. As lipid oxidation progresses, low molecular weight compounds such as malondialdehyde combines with TBA to form reddish compounds (Yu et al., 2017), and the color intensifies as the generated amount of malondialdehyde increases (Halliwell et al., 1990).
The alcalase protein hydrolysate, which was confirmed to have relatively high antioxidant activity from the various antioxidant tests, was used to determine the inhibitory effect on linoleic acid peroxidation, and trolox was also used as the positive control group at 2 μg/mL. From day 2 through day 4, lipid peroxide generation increased in both the protein hydrolysates and control group, and after day 3, linoleic acid oxidation was significantly inhibited following treatment with alcalase protein hydrolysate compared to that in the control group (Fig. 4(A)). In addition, the comparison results of the oxidation inhibition activity by concentration (Fig. 4(B)) suggested that the activity significantly depends on the concentration of alcalase protein hydrolysate.
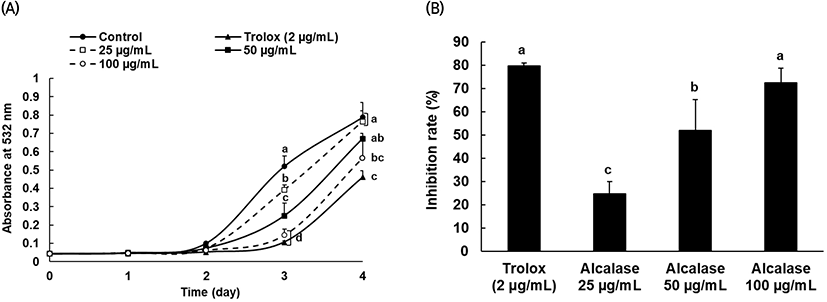
4. Conclusions
This study established the conditions for preparing hydrolysates using various proteases with defatted hemp seed powder as raw material. The antioxidant activities of the protein hydrolysates prepared using each enzyme were also compared. The degree of hydrolysis of each enzyme showed that all enzymes (alcalase, bromelain, flavourzyme, and neutrase) excluding papain showed a high degree of hydrolysis, and flavourzyme particularly showed a relatively high degree of hydrolysis. Compared to that in the non-enzyme group, the total polyphenol compound content was significantly higher in all the enzyme groups, among which alcalase, bromelain, and neutrase protein hydrolysates showed relatively higher total polyphenol contents. Regarding the ABTS radical scavenging activity, H2O2 scavenging activity, and 2-deoxy-D-ribose oxidation inhibition activity, all enzyme groups showed higher antioxidant activity than the non-enzyme group, and the antioxidant activity of alcalase protein hydrolysates was, in particular, relatively higher. In addition, alcalase protein hydrolysates with outstanding overall antioxidant activity and total polyphenol content were used to identify the inhibitory effect on linoleic acid peroxidation. The results showed that the inhibitory effect was significantly dependent on the concentration. The antioxidant activity of alcalase protein hydrolysates and soybean protein hydrolysates, reported to have outstanding antioxidant activity, were compared. The results suggested that the defatted hemp seed protein hydrolysates prepared using alcalase had relatively higher antioxidant activity than the defatted soybean protein hydrolysates. Through this study, preliminary data for establishing the optimal enzyme preparation conditions necessary for the preparation of defatted hemp seed protein hydrolysates were obtained. Further, the utilization potential of hemp seed for developing natural antioxidation material was confirmed.