1. Introduction
Honey is a distinctive natural product which is composed of simple sugars (glucose and fructose: 60-85%), water (10-20%), and other minor constituents such as enzymes, amino acids, organic acids, vitamins, minerals, carotenoids and phenolic compounds (Mohd Kamal et al., 2021; Samborska, 2019). Malaysian honey has high commercial value due to its distinct flavour and aroma as well as its therapeutic properties (Chan et al., 2017; Yap and Chin, 2021). Malaysian honey is commonly categorised according to bee species (e.g. Apis, Trigona/kelulut) and flower sources (e.g., Acacia, Gelam, Tualang) (Chan et al., 2017; Kek et al., 2014; Md Dan et al., 2018; Mohd Kamal et al., 2021; Yap and Chin, 2021).
In modern diet, an excessive intake of added refined sugar in foods and beverages can lead to several health impacts (Arshad et al., 2022; Sarkar et al., 2023). As a consequence, alternative natural sweeteners have been introduced by food producers to meet the demand and expectation of health-conscious consumers (Arshad et al., 2022; Sarkar et al., 2023). Natural sweeteners such as palm sap, date palm, beet root, honey, coconut, and grapes can be used to replace the refined sugar because they can provide additional nutritional values and potential health benefits (Arshad et al., 2022; Sarkar et al., 2023; Sathivel et al., 2013; Tong et al., 2010). Thus, honey can be considered as a good alternative to sugar in formulation of food, medicinal and pharmaceutical products. Unfortunately, its high viscosity and density hinders its use as an additive for these products (Tong et al., 2010). Honey is similar with other sugar-rich ingredients, its stickiness is a major issue in process handling and spray drying because it has high flow resistance and in the dried products as it promotes hygroscopicity and agglomeration (Md Dan et al., 2018; Sathivel et al., 2013). Therefore, it is desirable to transform liquid honey into solid powders. Moreover, previous studies showed that the stability and quality of bread and cookies were improved when honey powder was used as sugar substitute in the dough formulation (Samborska, 2019; Sathivel et al., 2013; Tong et al., 2010).
Production of honey powder using different techniques has been carried out, namely spray drying, tunnel drying, and vacuum-microwave drying (Cui et al., 2008; Nurhadi et al., 2012; Samborska, 2019; Samborska et al., 2015; Sathivel et al., 2013; Suhag et al., 2021; Tomczyk et al., 2021). Among all, spray drying is the most used technique to produce honey powder because it is a cost effective, well established, and industrial scaleup-able system. Unfortunately, the honey content in the spray dried honey powder is usually less than 50% (Nurhadi et al., 2012; Samborska, 2019; Samborska et al., 2015; Sathivel et al., 2013; Suhag et al., 2021; Tomczyk et al., 2021). In addition, a careful selection and formulation of drying carriers (e.g. maltodextrin, whey protein, Arabic gum) is required to manipulate the glass transition temperature of the honey-drying carriers mixture to reduce surface stickiness of the powders during spray drying process (Samborska et al., 2015).
Encapsulation of natural food in calcium alginate beads using an atomisation system has been widely reported. The natural food is mixed in sodium alginate solution prior to the atomisation process. The mixture is then atomised into fine droplets, and they are allowed to fall into a collection bath made of calcium chloride solution where the droplets are solidified via ionic crosslinking gelation to produce gel beads. Subsequently, the gel beads can be dried to obtain gel powders (Mandal et al., 2010; Vreeker et al., 2008). This method is simple, low cost, and capable to produce gel powders with high loading of encapsulated natural food (Aguirre Calvo and Santagapita, 2019; Mandal et al., 2010). However, the use of atomisation system to produce honey encapsulated Ca-alginate gel powders has not been systematically studied.
The aim of this study was to systematically investigate the effect of solution formulation and process variables on the physical properties of the Malaysian honey encapsulated gel beads produced using a simple atomisation system and then they were dried using a vacuum oven. The physical properties of different formulations of feed solution made of Malaysian honeys and sodium alginate for the atomisation system was investigated. The effect of process variables of the atomisation system on the size, shape and encapsulation efficiency of Malaysian honey encapsulated gel beads was investigated. The size, shape, moisture content, and bulk density of Malaysian honey encapsulated gel powders after vacuum drying were evaluated.
2. Materials and methods
Three types of Malaysia honey were used in this study, namely Acacia, Tualang and Gelam. The honeys were purchased from local suppliers in Malaysia. Sodium alginate powder (KIMICA Corporation, Chiba, Japan) and honey were mixed in distilled water using an agitator motor (IKA, Rawang, Malaysia). The honey-alginate solutions with different concentrations of alginate (i.e., 1.0, 1.5, and 2.0% w/v) and honey (i.e., 20, 40, and 60% w/v) were prepared.
An air atomisation system was developed in-house. It consists of coaxial air spray nozzle (inner and outer diameter of 0.8 mm and 1.2 mm, respectively) connected to a tube that delivers sodium alginate-honey mixture with the aid of a peristaltic pump (Watson Marlow, Falmouth, UK) and a tube that delivers compressed air from a compressor (Airgen, Taoyuan, Taiwan). The flow rate of compressed air was controlled using an air flow meter (Omega Engineering, Bridgeport, US). The honey-alginate solution was atomised into fine gel beads before they were collected and gelled in a calcium chloride solution that was placed underneath the nozzle. After 30 minutes of curing in the bath, the gel beads were separated from the bath and placed in a vacuum oven (local custom made, Malaysia) for drying (70°C, 0.02 atm, 24 hrs). Fig. 1 shows the setup of the air atomisation system coupled with a vacuum oven for honey encapsulated gel powder production.
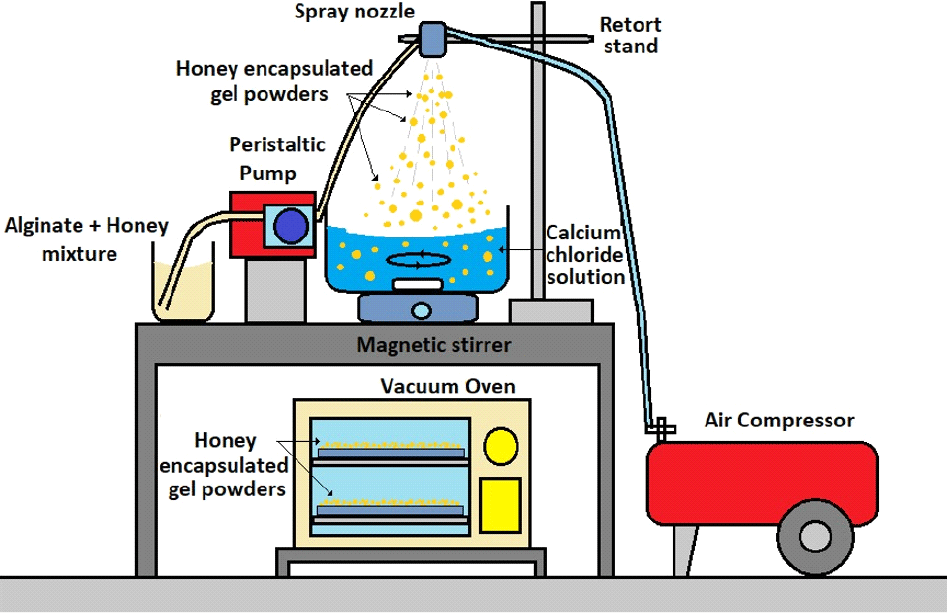
The density and viscosity of honey-alginate solution were measured using a hydrometer (Zeal, London, UK) and a viscometer (Brookfield, Middleborough, US), respectively. The surface tension of the honey-alginate solutions was determined by the modified drop weigh method, known as the LCP coefficient method (Lee et al., 2009).
An image of 30 honey encapsulated gel beads and powders was captured using a digital camera (Iphone 13 Pro, Apple, China). The diameter of the beads and powders was determined using an image analyzer software (Image J, USA). The maximum (dmax) and minimum (dmin) diagonal lengths of the gel beads and powders which were determined using the image analyzer software (Chan et al., 2009). The sphericity factor (SF) was calculated from the following equation.
In this study, the gel beads and powders were considered as perfectly spheres when their SF was less than 0.05, and the gel beads and powders were considered as distorted when their SF was above 0.25.
The total soluble solids of the collection bath were determined using a portable digital refractometer (Pocket Refractormeter, PAL-1, Atago Co., Ltd., Tokyo, Japan). For each measurement, two drops of collection bath solution were dripped into the sample stage of the refractometer. The sample was spread on the optical glass of the refractometer and the readings were recorded in °Brix and indicated as the concentration of encapsulation honey that was leached out from the gel beads in the collection bath (CL). The ‘theoretical’ maximum concentration of the encapsulated honey that was possibly leached out from the beads was calculated (Cmax) (Lopez-Sanchez et al., 2018).
where CI is the concentration of honey encapsulated in the gel beads (%w/v), W is the total mass of encapsulated honey gel beads in the collection bath (g), V is the volume of the collection bath (mL).The encapsulation efficiency (EE) was calculated based on the ‘theoretical’ maximum honey concentration (Cmax) and the experimental measured honey concentration in the collection bath (CL) (Lopez-Sanchez et al., 2018).
The moisture content of the gel beads was measured using the direct gravimetric method. The initial weight of the gel beads was measured by a digital weighing balance (mi) (Sartorius, Goettingen, Germany). A vacuum oven (local custom made, Malaysia) was used to dry the gel beads at 70°C for 24 hrs. After drying, the gel powders were weighted (mf) and their moisture content (mw) was calculated.
A modified mathematical model has been developed to predict the diameter of Ca-alginate particles (dB) produced at intermediate air-liquid mass flow rate ratios using the atomisation system (see Fig. 1.) (Chan et al., 2012; Mansour and Chigier, 1995).
In which, Ohnersorges number (Oh) is used to show the importance of viscous forces to surface tension forces in the honey-alginate solution during gel beads formation process. Weber number (We) is used to show the importance of aerodynamic force of air to surface tension of hone-alginate solution during the atomisation process.
where μ is viscosity of honey-alginate solution (kg/m.s), ρL is density of honey-alginate solution (kg/m3), γ is surface tension of honey-alginate solution (N/m), dN is the nozzle diameter (m), ρa is density of air (kg/m3), and v is air velocity (m/s).The diameter and SF of the gel beads and powders were measured with sample size of 30. The standard deviation of the data was shown using an error bar in the graphs and in parentheses in the tables. One-way analysis of variance (ANOVA) was conducted at 95% confidence interval using Minitab Statistical Software version 15 (Minitab, LLC, State College, PA, USA). Statistical error analysis was conducted to evaluate the accuracy of the gel bead diameter prediction model. The average absolute deviation was used to respectively indicate the average deviation of the predicted data from experimental data.
3. Results and discussion
The physical properties of the mixture of Malaysian honeys and alginate solutions are presented in Table 1. In general, the density and viscosity of honey-alginate solution increases as the concentration of honey increases, regardless of alginate concentration. It is also observed that the density and viscosity of honey-alginate solution was increased when the concentration of alginate was increased for a specified honey concentration. The honey-alginate solutions are more dense than pure water because the honey and alginate in the solution contributes to the mass of the entire solution. However, the density of honey-alginate solutions was lower than that of pure honey (Oroian, 2013). The increase of both honey and alginate concentration in the solution had exponentially increased the viscosity of the solution because both substances are commonly used as solution thickener (Chan et al., 2009; Oroian, 2013). It is interesting to note that the density and viscosity of Tualang-alginate solution is the highest among the three tested Malaysian honey types. On the other hand, the surface tension of honey-alginate solutions was decreased as the concentration of both honey and alginate concentration was increased. This is because when biopolymer is dissolved in water, they tend to accumulate at the liquid surface instead of remaining in the bulk (Hunter, 2001). The surface tension of honey-alginate solutions of same concentration was not significantly influenced by the honey type (p>0.05). The feed solution properties (i.e., density, viscosity, and surface tension) play a significant role in the air atomisation performance (Chan et al., 2012; Mansour and Chigier, 1995). The properties of the Malaysian honey-alginate mixture of different formulations were in the typical range of operating conditions of air atomization system for production of calcium alginate gel beads (Chan et al., 2012; Lee et al., 2016; Mansour and Chigier, 1995).
Fig. 2 depicts the diameter and SF of Acacia honey encapsulated gel beads produced using different concentrations of alginate and flow rates of compressed air. In general, the diameter of the beads decreases as the concentration of alginate increases, for all the tested air flow rates (p<0.05). Since the viscosity of the Acacia honey-alginate solution is increased as the concentration of alginate is increased, the liquid breakup during atomisation of viscous solution is more vigorous and complex, the resultant droplets (or the gel beads) are less uniform (Chan et al., 2012; Mansour and Chigier, 1995). As a result, the SF of the gel beads produced from viscous honey-alginate solution was higher than 0.05 (p<0.05). On the other hand, the diameter of the honey encapsulated gel beads produced using different alginate concentrations was significantly decreased when the air flow rate was increased (p<0.05). The opposite data trend was observed for the SF of the gel beads when air flow rate was increased. The results are as expected because increasing the air flow rate increases the shear force caused by air at the coaxial air spray nozzle, which is capable to force the pendant droplet at the nozzle to detach before it reaches the maximum size supported by the surface tension force at the orifice of the nozzle (Chan et al., 2012; Lee et al., 2016). As a result, small droplets are generated and fell into the collection bath, small gel beads are produced in the end. On the other hand, the increase of shear force during atomisation also has increased the tendency of the droplets to collide each other or to hit the collection bath at high velocity, hence the resultant gel beads are deformed and less spherical (Chan et al., 2012; Lee et al., 2016).
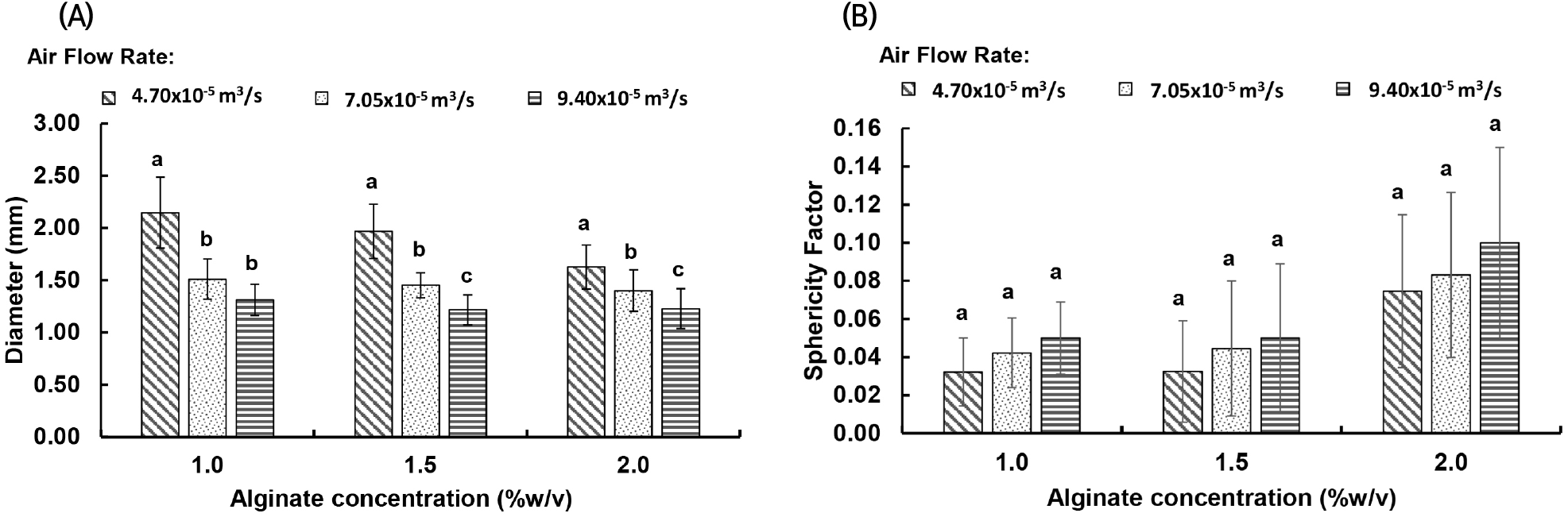
Fig. 3 illustrates the diameter and SF of wet and dried gel beads produced from different concentrations of Acacia honey and different types of Malaysia honey. As expected, the diameter of the wet gel beads was decreased after they were processed through vacuum drying. The diameter of the dried gel powders was approximately 50% of their original wet size, which fell within the range as reported in previous studies (Gal and Nussinovitch, 2007; Smrdel et al., 2008). The wet and dried gel beads produced from 20%w/v Acacia honey were relatively smaller as compared to the rest of the wet and dried samples. The diameter of the wet and dried gel beads was increased by the concentration of honey (p<0.05). Among the three types of Malaysian honey, the wet and dried gel beads produced from Gelam honey were smaller than the rest of the samples (p<0.05). This could be due to low viscosity of Gelam honey which allowed a greater degree of shrinkage to occur during vacuum drying as compared to the other samples (Gal and Nussinovitch, 2007). On the other hand, the gel beads were spheroids after drying, they were round with low SF values with large variations as compared with those of wet gel beads. The results were in agreement with the sphericity of gel beads after drying in previous studies (Gal and Nussinovitch, 2007; Smrdel et al., 2008). The SF of the samples was not apparently influenced by the honey concentration and type (p> 0.05).
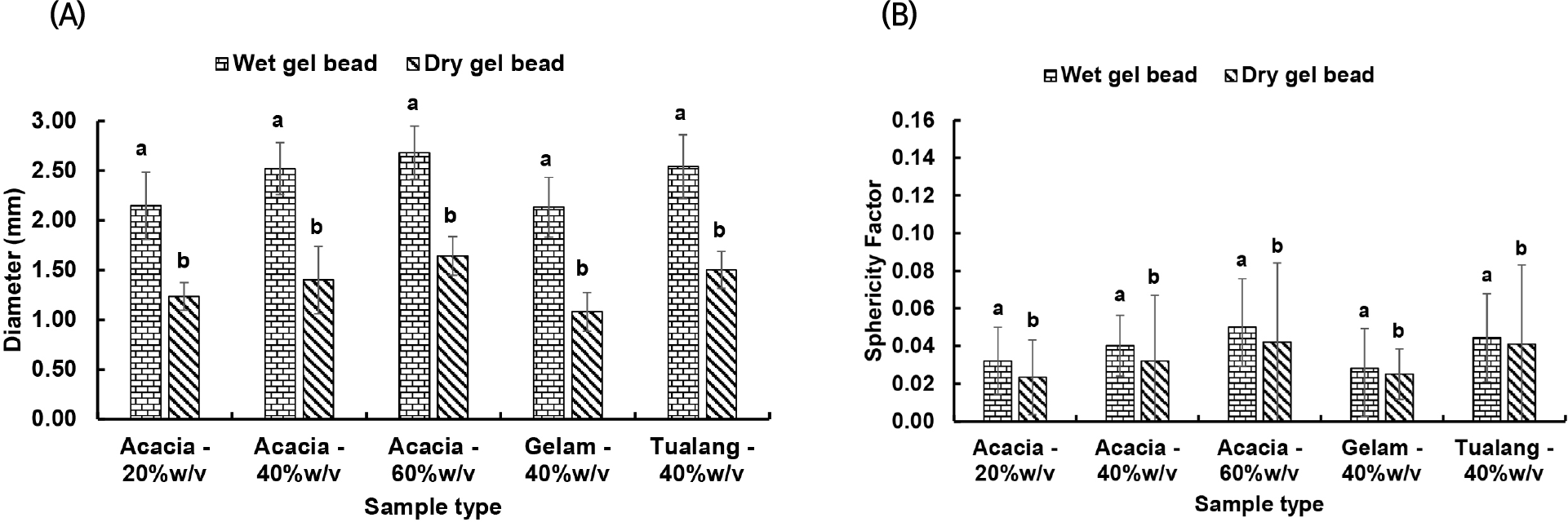
In this study, the honey encapsulated gel beads were produced at intermediate air-liquid mass flow rate ratio which was in the range of 0.22-0.31, Weber number (We) range of 112-545 and Ohnersorges number (Oh) range of 0.35-10.46 (Chan et al., 2012; Mansour and Chigier, 1995). The diameter of honey encapsulated gel beads and powders obtained from the calculation based on the mathematical model and experimental runs was presented in Fig. 4. Based on the results, the diameter of the honey encapsulated gel beads was predicted by the mathematical model with an average absolute deviation of 20%. The mathematical model is capable to provide a good estimation of the diameter of the honey encapsulated gel beads that can be produced using the atomisation system. However, the diameter of the gel powders was over-estimated by the mathematical model. Since the gel beads underwent vacuum drying, the gel beads were dehydrated and shrunk in size (see Fig. 3).
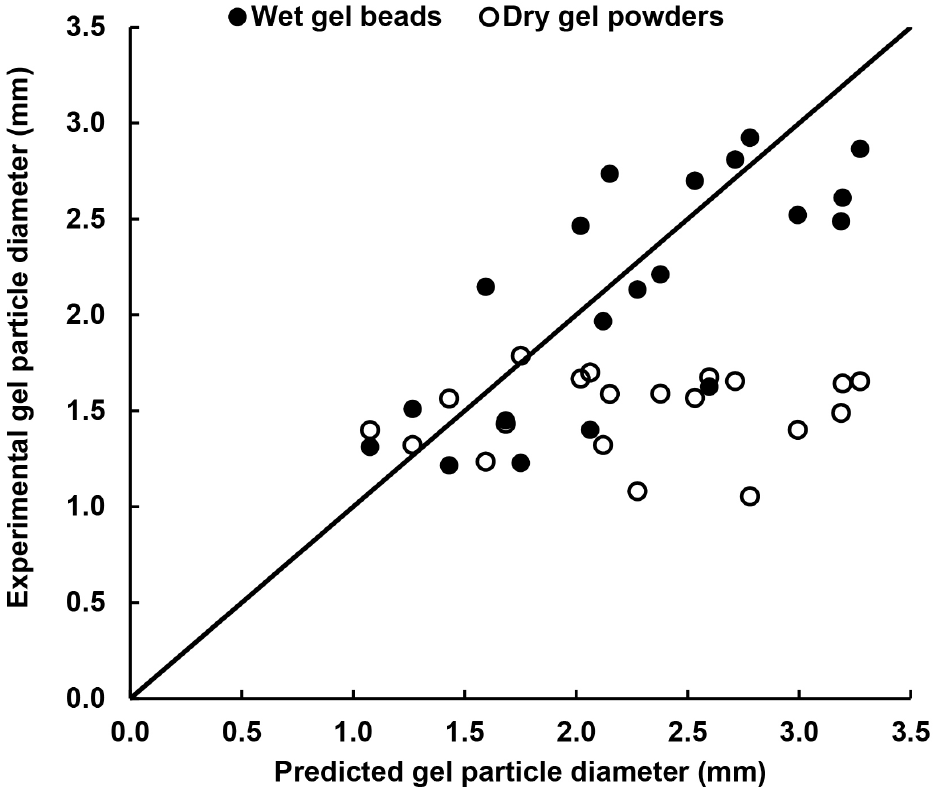
The efficiency of the gel beads produced using different formulations to encapsulate different types and concentrations of Malaysia honey was investigated. Table 2 shows the gel beads are capable of encapsulating Malaysian honeys with efficiency greater than 94%. The encapsulation efficiency of 20%w/v Acacia honey in calcium alginate gel beads was significantly influenced by the alginate concentration (p<0.05). The density of calcium alginate gel matrix formed in the bead is proportional to the concentration of alginate solution (Tanaka et al., 1984). Therefore, the diffusion resistance of the encapsulated compounds through the high-density gel bead is increased as the concentration of alginate is increased (Tanaka et al., 1984). As a result, the encapsulation efficiency is improved. It is noted that the encapsulation efficiency is also improved when the honey concentration is increased in the gel bead produced from 1.0 and 1.5% w/v alginate solution (p<0.05). The diffusion rate of the honey is expected to be reduced when the viscosity of the honey is increased at high concentrations. Hence, high encapsulation efficiency (approximately 97%) can be achieved by the gel beads produced using viscous honey-alginate mixture (p>0.05). On the other hand, the encapsulation efficiency of Acacia honey in the gel beads was significantly lower than that of other types of Malaysian honey (p<0.05). The variations in the physical quality and purity of the tested honeys could be the reason for the obtained results.
Table 3 shows the moisture content of the honey encapsulated gel beads after they underwent vacuum drying. All the samples experienced about 90% loss of their initial weight as the moisture contained in the samples was dried. The moisture content of the dry Malaysian honey encapsulated gel powders was within the common range of honey powders produced using spray drying and oven drying (Samborska et al., 2015). Honey powders with low moisture content has the ability to be easily blended with other ingredients apart from other advantages including convenience, ease of handling, reduced storage space, sanitation and storage for a longer period (Samborska et al., 2015). As a comparison, the moisture content of gel beads made from Gelam and Tualang honey was lower than the rest of the samples (p<0.05). The reason could be the moisture content of Gelam and Tualang honey liquid at initial was lower than that of Acacia honey (Jaafar et al., 2012; Mohd Kamal et al., 2021), hence a lesser portion of moisture was removed from the Gelam and Tualang honey encapsulated gel beads during the drying in the same conditions.
The bulk density of honey encapsulated powders produced from different formulations is presented in Fig. 5. The bulk density of the powders was in decreasing trend as the concentration of honey was increased (p<0.05). This could be due to the increase of powder diameter and powder uniformity when the concentration of honey was increased (as shown in Fig. 3). Moreover, the powders made from Gelam and Tualang honey were relatively smaller and spherical, their bulk density was also higher as compared to those of Acacia honey encapsulated powders (p<0.05). The bulk density of the samples of the current study is higher than that of honey powders produced using spray drying method (commonly in the range of 0.35-0.70 g/cm3) (Samborska, 2019; Samborska et al., 2015; Suhag et al., 2021).
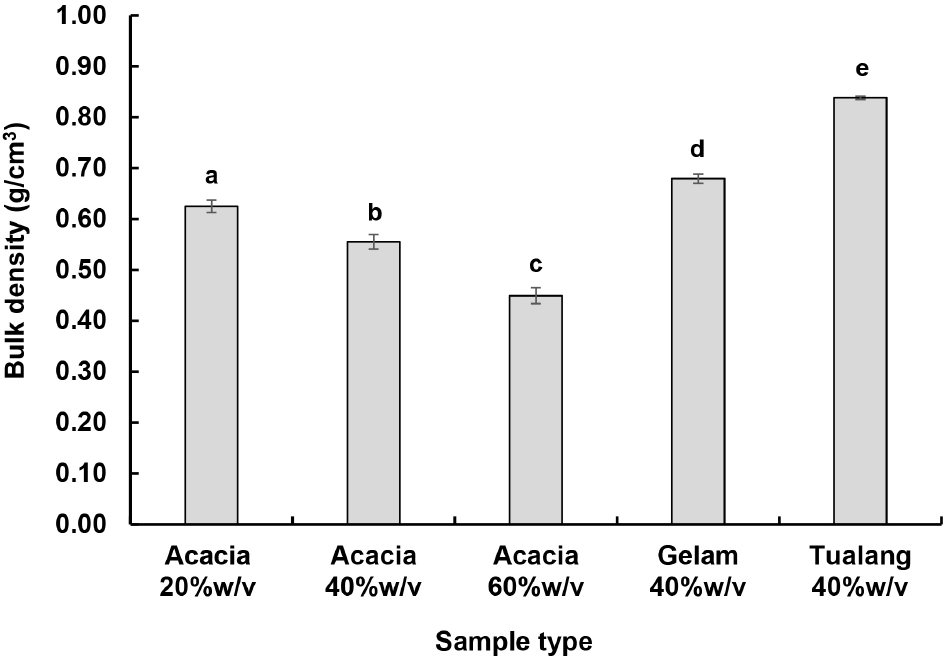
4. Conclusions
The production of Malaysian honey encapsulated gel beads and powders using a sequential method involving air atomisation and vacuum drying was systematically investigated. The physical properties of the feed solutions of the air atomisation system were characterized. The density, viscosity and surface tension of the honey-alginate solutions was determined to be in the range of 1,071-1,483 kg/m3, 102-2,913 mPa.s, and 70.1-85.4 mN/m, respectively. The physical properties of the Malaysian honey-alginate solutions were in the typical range of operating conditions of air atomization system for production of calcium alginate gel beads. Malaysian honey encapsulated gel beads in the size range of 2.16-2.92 mm were produced under the operating conditions of the air atomisation system: the air-liquid mass flow rate ratios of 0.22-0.31, Weber number (We) of 112-545 and Ohnersorges number (Oh) of 0.35-10.46. After vacuum drying, the gel powder in the size range of 1.50-1.79 mm were produced. The diameter of gel beads was decreased when alginate concentration was increased but an opposite effect was observed when the honey concentration was increased. Since the atomisation was conducted at intermediate air-liquid mass flow rate ratios, the gel beads/powders were generally uniform and spherical with low sphericity factor (SF ) values. The diameter of the gel beads can be predicted using the developed mathematical model with an acceptable deviation. Therefore, the developed mathematical model can be used for future applications to produce honey encapsulated gel beads with a desired diameter by manipulating the solution properties and process variables of the air atomisation system. The atomisation system coupled with vacuum drying is simple and capable to produce gel powders with high content of encapsulated honey (up to 60%w/v) and good encapsulation efficiencies (>94%). The diameter prediction mathematical model can be improved by taking consideration of the shrinkage factor due to vacuum drying in further studies, so that the diameter of dry gel powders can be predicted. In order to understand the functionality of the gel powders, the rehydration properties of the gel powders in water and the bioactive compounds retained in the gel powders will be evaluated in future studies.