1. Introduction
Reactive oxygen species (ROS) are the byproducts of metabolic processes in the body. They include H2O2, hydroxyl radicals, singlet oxygen, and superoxide anions. The production of an adequate amount of ROS is critical in cell functions from the activation of white blood cells to intracellular signaling. However, an excessive amount of ROS may cause various adverse conditions, such as cancer, inflammation, and aging, by damaging cells and tissues (Benzi and Moreti, 1995; Fiers et al., 1999; Fukuzawa and Takasoshi, 1990; Halliwell et al., 1992; Rhim and Choi, 2010). To reduce ROS production, synthetic antioxidants, such as butylated hydroxyanisole and butylated hydroxytoluene (BHT), have been used therapeutically. However, legal restrictions have been enacted regarding their methods of use and dosages, as their long-term administration at high dosages may induce side effects, such as cancer (Lee et al., 2012; Shin, 1999; Yen and Hsieh, 1998). Thus, to overcome the instabilities of synthetic antioxidants, an increasing number of studies have been reported recently regarding natural materials with antioxidant activities (Masaki et al., 1995), ranging from various fruits (Cho et al., 2005; Kim et al., 2021) to vegetables (Lee et al., 2009; Nam and Kim, 2017) and herbal medicines (Lee and Ryu, 2019; Lim et al., 2017).
Soybean, which originates from the Manchurian region, has been cultivated in Korea since the period of the Three Kingdoms. It is a food with an outstanding amino acid composition and protein content (Rhim et al., 2003; Shon et al., 2009). Via soybean germination, soybean sprouts are obtained as an excellent nutritional food with an enhanced rate of digestion, as starch is degraded to monosaccharides and the contents of vitamins, proteins, and fibrins increase (Collins and Sand, 1976; Kim et al., 1993). Soybean sprouts also facilitate the production of nicotinamide adenine dinucleotide in the body to promote the synthesis of alcohol dehydrogenase, and thus, they exhibit a remarkable hangover-relieving effect (Park, 1994). Furthermore, soybean sprouts contain isoflavone, which improves menopausal symptoms and prevents osteoporosis, in addition to its anticancer and antioxidant effects, with a role in immunity, along with vitamin C, and a preventive effect on cardiovascular disease, along with fatty acids (Choi et al., 2000; Kim et al., 2003a). Thus, previous studies reported the hypoglycemic effect of a methanol extract of soybean sprouts in rats with streptozotocin-induced diabetes (Kim et al., 2003b) and the effect of a beverage containing an extract of soybean sprouts cultivated in high-concentrated oxygen water on alcohol degradation (Sung et al., 2014). Another study reported the changes in the physicochemical and sensory properties of a soybean sprout extract caused by stir-frying (Hong et al., 2020). Ongoing studies are investigating processed foods containing soybean sprouts as their main ingredients.
Hovenia dulcis Thunb. is a deciduous broad-leaved tree of the Rhamnaceae family that is cultivated in regions of East Asia, including Korea, Japan, and China (Choi et al., 2014; You et al., 2009). The H. dulcis Thunb. fruit is approximately 8 mm in diameter, with a delicate aroma and sweetness. H. dulcis Thunb. fruits contain compounds such as ampelopsin, with an outstanding hangover-relief effect, and thus, they prevent liver damage and improve liver function in contributing to recovery from fatigue (Kim et al., 2000; Kim et al., 2011; Lim et al., 2007; Park et al., 2015). Other effects include antiosteoporotic, antiarthritic, and antistress and antioxidant activities, but few studies evaluate the effects of combinations with soybean sprouts (Cha et al., 2014; Na et al., 2013).
Thus, this study investigated the synergistic effect of the combined use of soybean sprouts and H. dulcis Thunb. fruits by measuring the physicochemical properties and bioactivities to provide basic data regarding the potential use of both materials as ingredients in functional food products.
2. Materials and methods
The soybean sprouts, clam and mussel meat, pen shell scallops, kelp, dried shiitake mushrooms, leeks, daikon, onions, and bay salt used in this study were purchased as domestic raw materials. H. dulcis Thunb. fruit concentrate with 60 °Brix was purchased from Himchanstep (Iksan, Korea) and stored at 4°C prior to use. The mixing ratio of the materials used in preparing the extract of the raw material mixture is shown in Table 1. Kelp and dried shiitake mushrooms were added to purified water and extracted for 1 min at 100°C. After removing the kelp, clam and mussel meat and pen shell scallops were added and extracted for 2 h, and then leeks, daikon, onions, and bay salt were added and extracted for 35 min. Soybean sprouts (33% w/w) were added to the resulting extract of the mixture (67%) and extracted 100°C for 20 min. To obtain the soybean sprout extract (BSE), the solid materials were removed via filtration. The H. dulcis Thunb. fruit concentrate was added to purified water to produce 1.5% w/v H. dulcis Thunb. fruit extract (HDE), and a soybean sprout extract (BHM) containing H. dulcis Thunb. fruit concentrate was prepared by adding the 1.5% w/v H. dulcis Thunb. fruit concentrate to the BSE. All samples used in the subsequent studies were the undiluted original solution.
The methods described by Ko et al. (1998) were used. After 10 min centrifugation of the sample at 890×g, the supernatant was collected and filtered using a 0.45 μm membrane filter (Advantec MFS, Inc., Dublin, CA, USA) through a Sep-Pak C18 cartridge (Waters, Milford, MA, USA). Twenty microliters of the filtrate was analyzed using high-performance liquid chromatography (Prominence Modular HPLC, Shimadzu Co., Kyoto, Japan; Alliance, Waters Co., Milford, MA, USA).
Sulfosalicylic acid (25 mg, Sigma-Aldrich Co., St. Louis, MO, USA) was added to 10 mL of the sample, and the mixture was stored at 4°C for 4 h and then centrifuged. The supernatant was collected and filtered using a 0.2 μm membrane filter (Pall Co., Port Washington, NY, USA), and an amino acid auto-analyzer (Biochrom 20, Pharmacia Biotech, Uppsala, Sweden) was used in the analysis.
The sample (100 mL) was mixed with 25 mL of digestive fluid (HClO4:H2SO4:H2O2 = 9:2:5, v/v/v), and the mixture was heated until it became transparent and filtered using a No. 2 filter paper (Advantec MFS, Inc., Dublin, CA, USA). The filtrate was added to distilled water to yield a final volume of 100 mL, and inductively coupled plasma (Aton scan 25, Thermo Jarrell Ash, Franklin, MA, USA) was used in the analysis.
The TPC was measured using the Folin-Ciocalteu method (Gao et al., 2000). Distilled water (8.4 mL), 0.5 mL of 2 N Folin-Ciocalteu reagent (Sigma-Aldrich Co.), and 1 mL of 20% w/v Na2CO3 (Junsei Chemical Co., Ltd, Tokyo, Japan) were added to 0.1 mL of the 10-fold diluted sample. The mixture was stored for 2 h, and its absorbance was then measured at 725 nm using an ultraviolet-visible (UV/Vis) spectrophotometer (U-1800, Hitachi Ltd., Tokyo, Japan). To quantify the TPC of the extract, a calibration curve was plotted using a diluted solution of gallic acid (Sigma-Aldrich Co.), and the TPC was expressed in μg gallic acid equivalent (GAE)/g.
The TFC was measured using the method described by Abdel-Hameed (2009) with modifications. NaNO2 (5%, 0.15 mL, Sigma-Aldrich Co.) was added to 1 mL of the 10-fold diluted sample and reacted for 6 min at 25°C. AlCl3 (10%, 0.3 mL, Sigma-Aldrich Co.) was then added, and the mixture was stored for 5 min, followed by the addition of 1 mL of 1 N NaOH (Sigma-Aldrich Co.). The absorbance was measured at 510 nm using the UV/Vis spectrophotometer (U-1800, Hitachi). To quantify the TFC of the extract, a calibration curve was plotted using a diluted solution of quercetin (Sigma-Aldrich Co.), and the TFC was expressed in μg quercetin equivalent (QE)/g.
To measure the DPPH radical scavenging activity, the method described by Blois (1958) based on the reducibility of DPPH was used. DPPH (0.4 mM, 3 mL, Sigma-Aldrich Co.) was added to 1 mL of the sample. The mixture was vortexed for 5 sec and then allowed to react in darkness for 30 min. The absorbance was measured at 517 nm using the UV/Vis spectrophotometer (U-1800, Hitachi), and 0.1% BHT and 0.1% α-tocopherol were used as controls.
To measure the reducing power, the method described by Yildirim et al. (2001) was used after modification. Phosphate buffer (pH 6.6, 0.2 M, 2.5 mL) and 2.5 mL of K3[Fe(CN)6] (1% w/v, Junsei Chemical Co., Ltd., Tokyo, Japan) were added to 1 mL of the sample, and the mixture was reacted at 50°C for 30 min. Trichloroacetic acid (2.5 mL, 10% w/v, Sigma-Aldrich Co.) was added to the mixture to stop the reaction, and the solution was then centrifuged at 3,000 rpm for 10 min. One milliliter of the supernatant was collected and mixed with 1 mL of distilled water and 0.2 mL of FeCl3 (0.1% w/v, Sigma-Aldrich Co.). The absorbance was measured at 700 nm using the UV/Vis spectrophotometer (U-1800, Hitachi).
To measure the H2O2 scavenging activity, the method described by Muler (1985) was used. The sample (100 μL) and 20 μL of H2O2 (Junsei Chemical Co., Ltd, Tokyo, Japan) were added to a 96 microwell plate and allowed to react in an incubator for 5 min at 37°C. Subsequently, 30 μL of 1.25 mM 2,2’-azino-bis (3-ethylbenzothiazoline-6-sulfonic acid) (Sigma-Aldrich Co.) and peroxidase (1 unit/mL, Sigma-Aldrich Co.) were added and allowed to react in the incubator for 10 min at 37°C. The absorbance was measured at 405 nm using the UV/Vis spectrophotometer (U-1800, Hitachi).
The experimental animals were 24 male 5-week-old Sprague-Dawley rats purchased from Hyochang Science (Daegu, Korea). The animal study was conducted with the approval of the Institutional Animal Care and Use Committee at Dong-A University, Busan, Korea (DIACUC-20-19). The animal breeding conditions were set at constant levels of temperature (22±2°C), humidity (50%±5%), and 12 h light-dark cycles, and the rats were provided with liberal amounts of food and water. Via randomization, six rats were assigned to each of the following four groups: alcohol-only (Con), BSE, HDE and BHM. Each sample was orally administered at 7 mL/kg, and after 30 min, 5 mL/kg of 40% alcohol was orally administered. Blood was collected from the caudal vein after 30, 60, and 90 min to measure the level of alcohol and after 3, 6, and 9 h to measure the level of acetaldehyde. The collected blood was stored at 25°C for 30 min and then centrifuged at 14,240 ×g for 10 min to isolate the plasma.
The blood alcohol and acetaldehyde concentrations were measured utilizing commercial kits for use in ethanol and acetaldehyde analyses (K-ETOHLQR and K-ACHYD, Megazyme, Bray, Ireland).
The mean and standard deviation were obtained using one-way analysis of variance for significant changes in each sample. The significance was evaluated by Duncan’s multiple range test using the Statistic Analysis System software 9.4, SAS institute Inc., Cary, NC, USA) (p<0.05).
3. Results and discussion
Considerable amounts of organic acids occur in foods, and they markedly affect food flavor (Joo et al., 1996). Table 2 shows the measured organic acid contents in BHM. Malic acid, with the highest content among those of the detected organic acids, enhances food flavor (Woo et al., 2012), and the respective detected amounts in BSE, HDE, and BHM are 559.32, 919.84, and 1,451.03 ppm. Thus, H. dulcis Thunb. fruit concentrate addition to BSE should enhance the flavor via changes in the organic acid composition.
BSE, soybean sprouts extract; HDE, 1.5% Hovenia dulcis Thunb. extract; BHM, soybean sprouts and 1.5% Hovenia dulcis Thunb. mixture.
Table 3 shows the analyzed contents and composition of free sugars in BHM. The respective sucrose contents in BSE, HDE, and BHM are 60.59, 294.60, and 375.09 ppm. The respective glucose contents are 215.03, 368.45, and 645.48 ppm, and the respective fructose contents are 257.18, 432.31, and 738.11 ppm. The results are consistent with the results of Park and Kim (2005) identification of glucose (7.25%) and fructose (5.38%) as the main free sugars in the H. dulcis Thunb. fruit. The results are also consistent with the free sugar contents reported by Kim (2004), where the degradation of sucrose during heating increased the contents of the products, glucose and fructose. Thus, heating during BSE production likely increases the contents of glucose and fructose, in addition to the increases in the sugar contents upon HDE addition.
Free sugars | Contents (ppm) | ||
---|---|---|---|
BSE1) | HDE | BHM | |
Sucrose | 60.59±0.07c2) | 294.60±0.02b | 375.09±0.01a |
Glucose | 215.03±0.05c | 368.45±0.01b | 645.48±0.03a |
Fructose | 257.18±0.02c | 432.31±0.05b | 738.11±0.02a |
Free amino acids react rapidly with other compounds in food materials. Notably, as they encounter sugars, reactions such as browning may occur to enhance the color and flavor or reduce the quality of food (Kim and Ahn, 2014). Table 4 shows the analyzed contents and composition of free amino acids in BHM. The most abundant free amino acids in BSE and BHM are taurine, arginine, and glutamic acid, with proline the most abundant in HDE. However, the content of proline is higher in BHM (2.92 ppm) than BSE (1.78 ppm) and HDE (1.18 ppm). The result is consistent with that reported by Oh et al. (1990): the extracts of the fruits of Lycium barbarum, Angelica gigas, Eleutherococcus senticosus, and Schisandra chinensis displayed variations in the contents and types of eluted free amino acids. The result is also consistent with that reported by Im and Lee (2019): the treatment conditions of an extract of burdock tea led to changes in the contents of the detected free amino acids. In addition, Satoh et al. (2002) reported that proline was crucial in free radical scavenging. Therefore, our result suggests that the free amino acid contents may vary due to differences in sample type, processing method, and mixing ratio. Additionally, our result suggests that further studies should verify the synergistic effect and investigate the antioxidant activities and bioactive materials in detail.
BSE, soybean sprouts extract; HDE, 1.5% Hovenia dulcis Thunb. extract; BHM, soybean sprouts and 1.5% Hovenia dulcis Thunb. mixture.
Table 5 shows the analyzed contents of minerals in BHM. Eight minerals, i.e., Zn, Cu, Fe, Mn, K, Ca, Na, and Mg, are detected. The respective contents of Zn in BSE, HDE, and BHM are the highest at 46.31, 16.74, and 36.28 ppm. The contents of Fe and Mn are higher in BHM than those in BSE or HDE: respective Fe contents of 16.86, 3.77, and 20.15 ppm in BSE, HDE, and BHM, with respective Mn contents of 2.09, 1.56, and 2.84 ppm. Therefore, the antioxidant efficacy of BHM should be high, with high contents of Zn, Fe, and Mn, which are involved in the inhibition of ROS (Lee et al., 2003).
BSE, soybean sprouts extract; HDE, 1.5% Hovenia dulcis Thunb. extract; BHM, soybean sprouts and 1.5% Hovenia dulcis Thunb. mixture.
The TPC and TFC of BHM are shown in Table 6. The TPC in BHM is high at 957.16 ppm, followed by those in BSE and HDE at 531.47 and 500.63 ppm, respectively. A similar trend is observed for the TFC, with a high level of 601.93 ppm in BHM, followed by those of BSE (373.21 ppm) and HDE (367.07 ppm). Natural antioxidants containing vitamins, carotenoids, phenols, and other such compounds serve as effective bioactive materials in preventing oxidative stress, which causes adverse conditions, including allergies, inflammation, and arteriosclerosis (Van, 2016). Additionally, polyphenolic compounds exhibit that may readily bind to proteins and various other compounds as aromatic compounds, and thus, display outstanding anticancer and -oxidant and anti-inflammatory effects (Kang et al., 2002a; Lee et al., 2008). This may be the cause of the excellent antioxidant efficacy of BHM in this study, as it is enriched with polyphenols and flavonoids.
Sample | Contents (ppm) | |
---|---|---|
Total polyphenol (μg GAE/g1)) | Total flavonoid (μg QE/g2)) | |
BSE3) | 531.47± 6.42b4) | 373.21±11.95b |
HDE | 500.63±5.50c | 367.07±14.42b |
BHM | 957.16±10.47a | 601.93±13.80a |
DPPH radical scavenging activity is measured to determine the radical scavenging activity of a given antioxidant material based on the change in absorbance as the antioxidant material donates hydrogen ions or electrons to return radicals to stabilized states (Lee et al., 2007). The electron-donating capacity of BHM is shown in Fig. 1. The respective measured activities of the positive controls 0.1% α-tocopherol and 0.1% BHT are 60.93% and 66.30%, and the respective activities of BSE, HDE, and BHM are 83.94%, 83.26%, and 89.94%. The result is consistent with the DPPH radical scavenging activities of the water extracts of H. dulcis Thunb. leaves and fruit reported by Jeong and Shim (2000). However, the result deviates from the slightly low DPPH radical scavenging activity of the heated extract of a mixture of mussel meat and soybean sprouts reported by Ok et al. (2014). This is likely due to the differences in the contents of antioxidant materials in each added ingredient, and thus, BSE, HDE, and BHM exhibit high DPPH radical scavenging activities in this study.
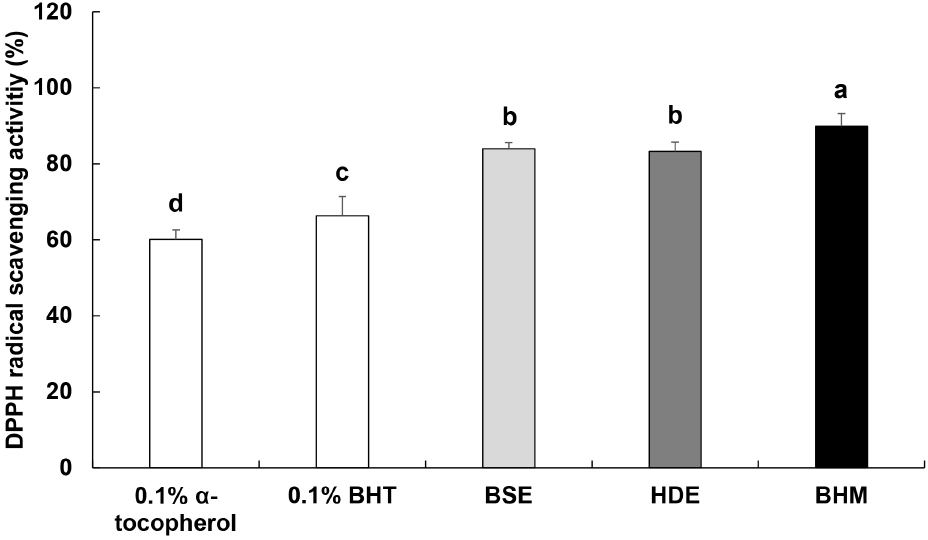
Reducing power is measured to identify the antioxidant activity based on the absorbance upon the conversion of Fe3+ to Fe2+ mediated by an antioxidant material (Kim et al., 2000). The measured reducing power of BHM is shown in Fig. 2. The respective reducing power values of BSE and HDE are 1.87 and 1.61, which do not vary significantly from those of 0.1% α-tocopherol and 0.1% BHT of 1.55 and 1.51, respectively. Conversely, the reducing power of BHM of 2.35 is the highest. Shiddhuraju et al. (2002) reported that the reducing power increases as the level of coloration increases, with a strong correlation between reducing power and antioxidant activity. Hence, BHM, with the highest absorbance, likely displays an excellent antioxidant efficacy.
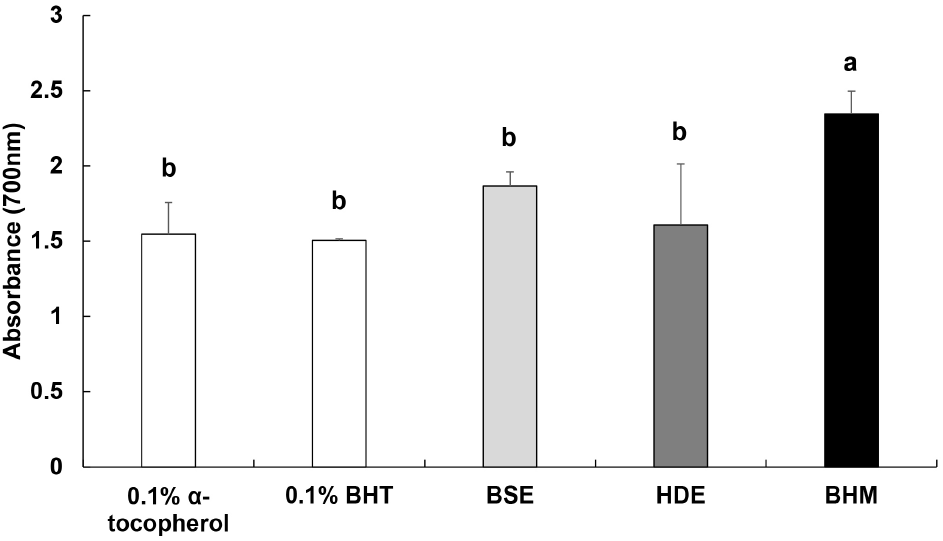
H2O2 exhibits effects such as aging and damages DNA, as it generates a high toxicity in the body via hydroxyl radicals (Kang et al., 2002b). The measured H2O2 scavenging activity of BHM is shown in Fig. 3. The respective scavenging activities of the positive controls 0.1% α-tocopherol and 0.1% BHT are 84.14% and 85.08%, and BHM exhibits the highest activity of 99.33%, followed by those of BSE and HDE at 96.50% and 95.20%, respectively. Lee et al. (2005) reported that the ROS scavenging activity and polyphenolic content correlate positively, and thus, the outstanding H2O2 scavenging activity of BHM is likely proportional to its high TPC, as shown in Table 6.
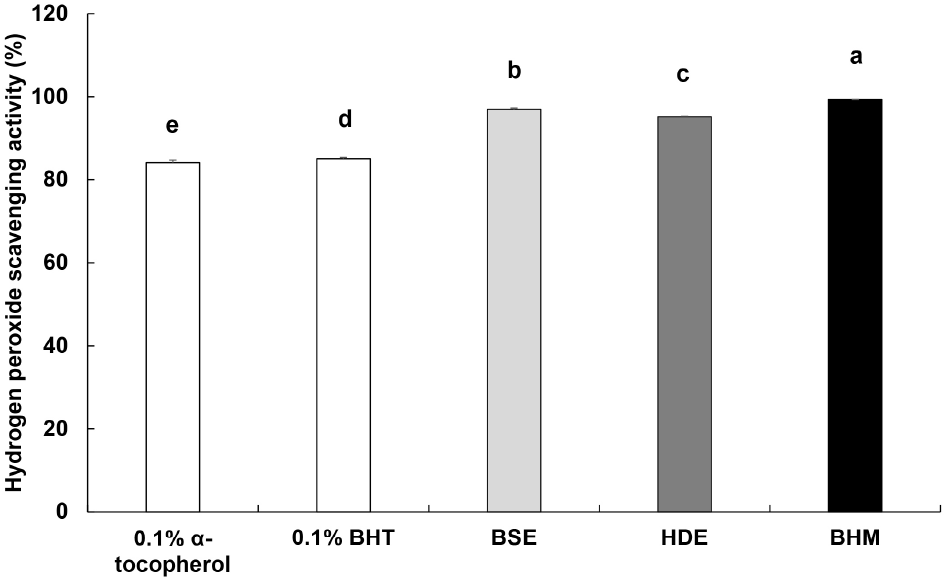
Sixty to ninety percent of alcohol is absorbed within 30 min of ingestion. The accumulation of acetaldehyde produced during alcohol oxidation, rather than the alcohol itself, causes liver damage and the hangover (Choi et al., 2014; Shumate et al., 1967). The concentrations of alcohol and acetaldehyde in blood, as measured to study the alcohol degradation effect of BHM, are shown in Fig. 4. The respective blood alcohol concentrations of Con, BSE, HDE, and BHM are 9.19, 5.30, 3.62, and 2.68 g/L at 30 min after ingestion, reaching the highest levels. In BHM, compared to those of Con, BSE, and HDE, the alcohol concentration is respectively decreased by 69%, 55%, and 38% at 90 min after ingestion and 89%, 83%, and 69% at 180 min after ingestion (Fig. 4(A)). A similar trend is observed for the blood acetaldehyde concentration of BHM: it is respectively decreased by 57%, 27%, and 16% at 3 h after ingestion, 50%, 31%, and 27% at 6 h after ingestion, and 59%, 47%, and 34% at 9 h after ingestion compared to those of Con, BSE, and HDE (Fig. 4(B)). Sung et al. (2014) reported that the blood alcohol and acetaldehyde concentrations may be significantly reduced via the oral administration of a powder form of soybean sprouts extract beverage in rats following the alcohol, and Ji et al. (2001) reported that H. dulcis Thunb. fruit may lower the blood alcohol concentrations in mice with acute alcohol toxicity. These results are consistent with the result in this study, where the blood alcohol and acetaldehyde concentrations are reduced via the administration of BSE and HDE. Compared to the use of only BSE or HDE, the combination may more effectively lower the blood alcohol and acetaldehyde concentrations via a synergistic effect, which may produce an outstanding hangover-relieving effect.
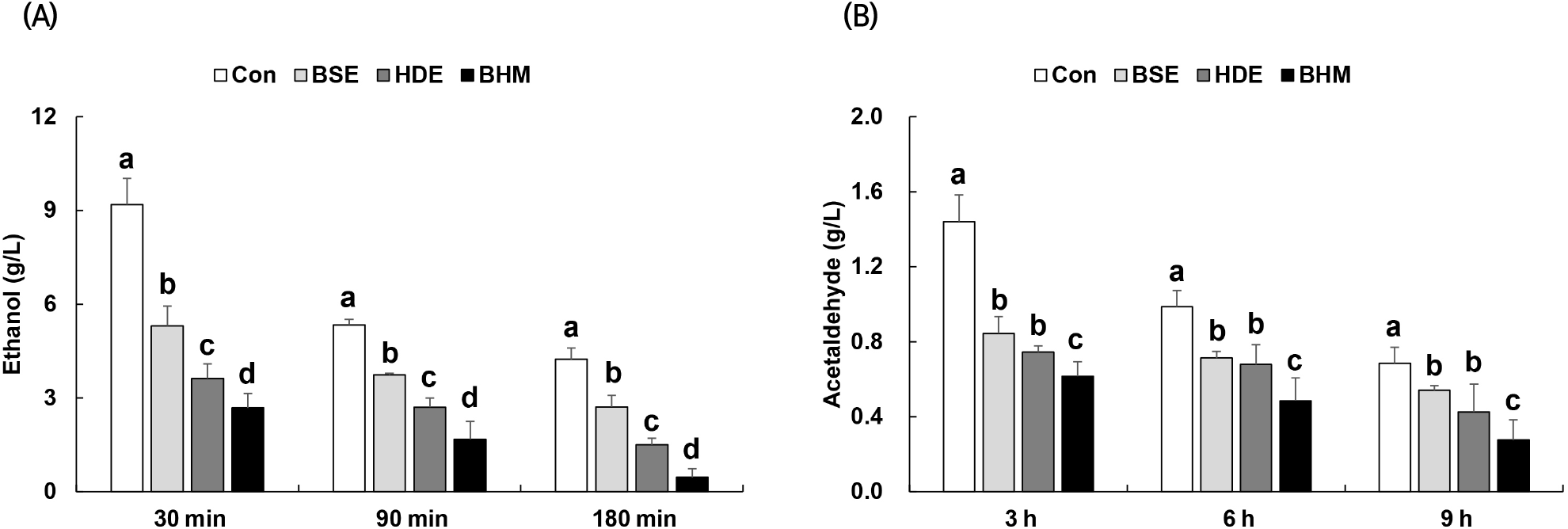
4. Conclusions
To develop a functional food material based on natural products, BHM was produced in this study, and its physicochemical properties and antioxidant activity were examined. The content of malic acid was the highest of those of the organic acids in BHM at 1,451.03 ppm, and the main free sugars in BHM were glucose and fructose, with respective contents of 645.48 and 738.11 ppm. Taurine was the main free amino acid in BHM, with a concentration of 11.95 ppm, followed by those of arginine and glutamic acid at 10.97 and 10.16 ppm, respectively. The most abundant minerals in BHM were Zn and Fe, and the respective TPC and TFC of BHM were 957.16 and 601.93 ppm. The antioxidant activity was measured based on the DPPH radical and H2O2 scavenging activities and reducing power. Compared to those of the positive controls, BHM exhibited an outstanding antioxidant efficacy, and the blood alcohol and acetaldehyde concentrations were significantly reduced. Based on the results, BHM displays potential as a component of functional foods, and the results of this study should provide basic data for use in future research.