1. Introduction
Marine algae, or seaweeds, are integral to Asian diets and traditional medicine, particularly in Korea. Their recognized health benefits have spurred global interest (Peñalver et al., 2020). Seaweeds are classified into brown, red, and green algae, each having distinct components (Lee et al., 2020a). Sargassum fusiforme (SF), a brown alga, is perennial and belongs to the Sargassaceae family (Lee et al., 2020b). Predominantly found along Korea’s southwestern coast, SF has a unique flavor and texture, making it popular in Korea, Japan, and China (Park and Ryu, 2013). SF is a rich source of dietary fiber, minerals, vitamins, and functional compounds such as polyphenols and polysaccharides, including fucoidan, laminaran, and alginic acid (Shin et al., 2014). Its polysaccharide, alginate, consists of α-L-guluronic and β-D-mannuronic acid chains, which form the cell wall. The resistance of alginate to degradation in vivo and its unique structure mean that it is physiologically active (Kwon and Youn, 2017). SF also contains phlorotannins, secondary metabolites formed of phloroglucinol units (Ferreres et al., 2012). Because of its high content of nutrients and bioactive compounds, SF has potential for disease prevention and treatment, for example, anti-inflammatory, anti-obesity, and anti-cancer effects (Sugiura et al., 2016; Wei et al., 2020). Ancient texts, such as the pharmacopoeia titled “Shen Nong’s Canon of Materia Medica,” document the therapeutic uses of SF, such as treating thyroid tumors and alleviating edema and reducing abdominal sounds (Zhang et al., 2020). In addition, the high glutamic and aspartic acid content, key flavor enhancers, of SF has led to the development of seaweed-based processed foods (Dai et al., 2019).
The Jeollanam-do province produces 1.68 million tons of seaweed, accounting for 91% of Korea’s overall seaweed yield, and the Wando region contributes 44% nationally (MOF, 2022; Wandogun, 2022). The ecological conditions of Wando, including a high ecological evaluation index, tidal currents, and species diversity, create an ideal seaweed habitat. This is attributed to the geography of the region, which ensure a steady influx of nutrient-rich coastal and open sea waters, including the cold bottom water of the Yellow Sea (Cho and Choi, 2005; Son, 2011). Notably, the nutrient profile and physicochemical properties of seaweed are strongly affected by the marine environment (temperature, tidal current, and inhabiting marine species), and this results in consumer preference for region-specific seaweeds (Lim and Choi, 2005).
In this study, we analyzed the nutritional composition and taste profiles of Sargassum fusiforme (SF), a seaweed vital to the economy of the Wando region an compared them to those of SF from another area in Jeollanam-do to establish a database for SF component analysis.
2. Materials and methods
SF samples were harvested from Wando (Wando-eup, WD-A; Sinji-myeon, WD-B) and a control region (Jodo-myeon, Jindo: control) in Dec 2022. After thorough washing under running water to remove impurities and salts, the SF was rinsed with distilled water, strained, and freeze-dried (Operon Co., Gimpo, Korea) for experiments.
The proximate composition of SF were determined following the AOAC (2019) method. Moisture was assessed using an air-oven at 105°C, crude fat was extracted by Soxhlet extraction, and crude protein was assessed using the Kjeldahl method with a nitrogen-protein analyzer (Kjeltec 8400 System, Foss, Hoganas, Sweden). The ash content was measured post-ashing in a muffle furnace (F6010, Thermo Fisher Scientific, Waltham, MA, USA) at 600°C. The carbohydrate content was calculated by subtracting the moisture, ash, protein, and fat content from the total weight of the sample, and the caloric value was calculated following Korean Food Code standards.
The samples (0.2-1.0 g) were mixed with nitric acid (7 mL) and hydrogen peroxide (1 mL) and heated for 30 minutes. After heating, the mixture was digested using a microwave digestion system (MARS 6, CEM Corporation, Matthews, NC, USA) and then cooled. Then, a sample was analyzed using inductively coupled plasma optical emission spectrometry (ICP-OES 8300, PerkinElmer Inc., Waltham, MA, USA).
Total dietary fiber was quantified by the AOAC method (2019). In this process, 50 mM MES/Tris buffer (40 mL; pH 8.2), α-amylase (50 μL), and sample (1 g) were mixed for 15 min at 95°C. After cooling to 60°C, the 100 μL of protease solution was added, and allowed to react for 30 minutes before adjusting the pH to 4.5. Subsequently, aminoglucosidase solution was added, and hydrolysis was carried out for 30 min at 60°C. The precipitate was then filtered and dried to measure the fiber content.
For constitutive amino acid analysis, a sample (0.1 g) underwent hydrolysis with 6 N HCl (10 mL) under nitrogen gas for 22 h at 110°C, followed by concentration in under reduced pressure. Free amino acids were extracted from the sample (0.5 g) with 70% ethanol (50 mL) for 30 min, followed by standing for 10 min; the sample was then centrifuged at 1,500 rpm for 15 min three times and concentrated in under reduced pressure. Pretreated samples were dissolved in 0.02 N HCl (20 mL), filtered, and analyzed with an automated amino acid analyzer (Hitachi L-8900, Hitachi, Tokyo, Japan) (Ham et al., 2021).
A sample (5 g) was added to distilled water (100 mL), stirred at 60°C for 1 h, and filtered (Whatman No. 2). The taste profile (sourness, sweetness, bitterness, saltiness, and umami) was assessed using an electronic sensor system (ASTREE II, Alpha MOS, Toulouse, France), and GPS and SPS sensors for calibration. The samples introduced into the device were analyzed using seven sensors for 120 s, and the sensors were washed between sample analysis to prevent contamination.
Independent experiments were conducted in triplicate, and the results are presented as mean±standard deviation. One-way ANOVA (SPSS version 27.0, SPSS Inc., Chicago, IL, USA) was used to determine the statistical significance, and Duncan’s multiple test post-hoc for group differences was set at p<0.05. Discriminant function analysis (DFA) was used to compare the taste pattern obtained from electronic tongue analysis.
3. Results and discussion
Proximate composition anaysis revealed that WD-A and WD-B SF had significantly higher crude protein contents than the control (Fig. 1). The ash content of WD-A was the significantly highest (40.67%), surpassing those of WD-B (37.20%) and the control (36.20%) (Fig. 1). The carbohydrate levels varied: the control region had the highest value (48.73%), followed by those of WD-B and WD-A at 46.07% and 43.13%, respectively. The caloric value of WD-A was the lowest (242.70 kcal/100 g). Further, the dietary fiber and crude lipid were similar for all samples (Fig. 1). Consistent with the findings of Jung (2017) for SF from Wando, Goheung, and Jindo regions, in this study, the SF from Wando showed elevated protein and ash contents compared to the other samples but similar crude lipid levels. The high ash content is attributed to the quartz porphyry of Wando, suggesting the role of the environment on the proximate contents, but there is need for further correlation studies.
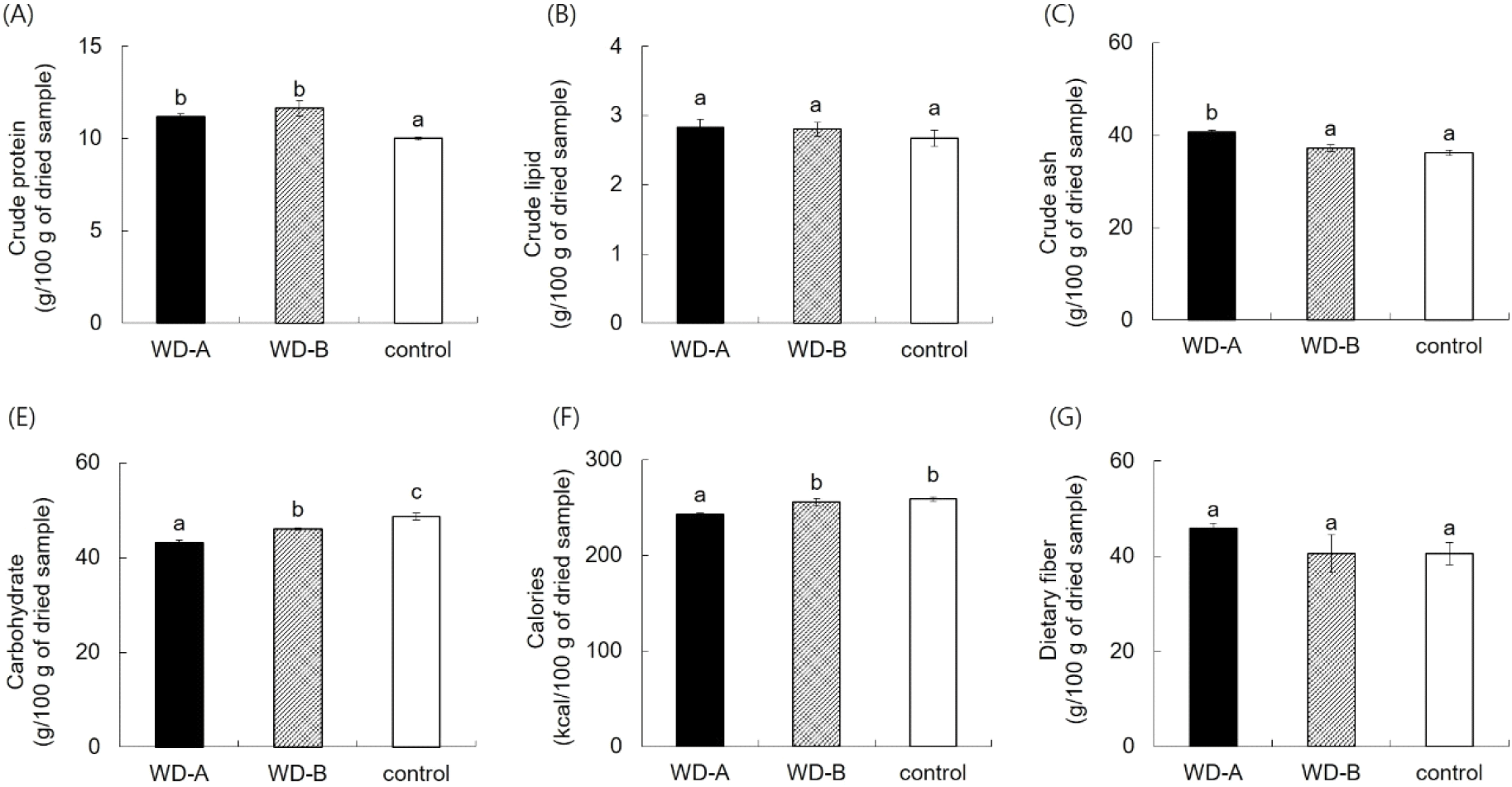
Seaweeds are valued for their mineral content, which can be similar to or exceed those of other foods (Lozano Muñoz and Díaz, 2020). WD-A SF contained significantly more potassium but less sodium, phosphorus, and magnesium than WD-B and the control (Fig. 2). Given the role of potassium in sodium excretion and the increase in sodium-related chronic diseases, potassium-rich foods such as SF are gaining attention. Na et al. (2022) linked a high sodium and low potassium intake to an increased prevalence of obesity and hypertension. As in our study, Yoon et al. (2022) found SF to have the highest potassium content among 11 seaweed types; specifically, a single serving (15 kcal) was found to provide 35% of the recommended potassium intake for adult males. Notably, the mineral profile of WD-A SF, which has high potassium and low sodium levels, stands out among the samples from different regions.
SF from WD-A contained 1.4- and 2.0-times greater iron levels than WD-B and the control, respectively, and this represents a significant difference (Fig. 2). Environmental factors such as ocean salinity, tidal currents, water temperature, and organic matter content influence the nutrient profiles of marine plants and benthic animals. Seaweeds, in particular, has been reported to be rich in minerals essential for growth and bioregulation. Because it selects and accumulates specific elements among the minerals in seawater (Yamamoto et al., 1984). The seawater of the Wando region is known for its high carbon absorption, and the iron concentration of the tidal flats, which is influenced by the quartz porphyry, is higher than other coastal areas, including the control region (data not shown). This environment likely contributes to the elevated iron levels in SF of WD-A. Additionally, SF of WD-A was found to have the highest zinc content (0.68 mg/100 g) of all samples (Fig. 2). Consistent with this finding, high zinc levels have been observed in samples of Lajonkairia lajonkairii from Wando, suggesting that both benthic organisms are affected by the unique marine conditions of the Wando region (Ham et al., 2021).
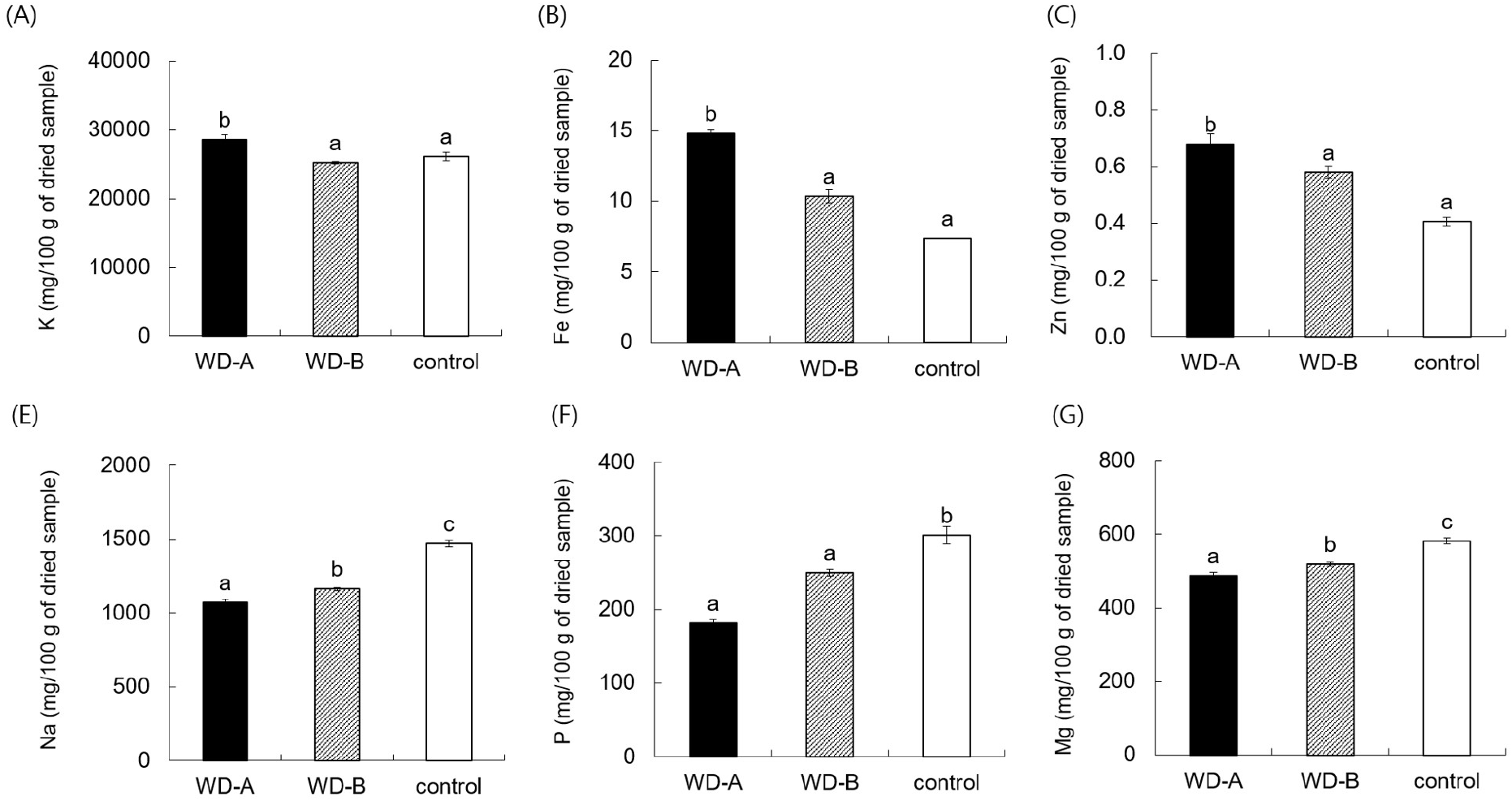
Amino acids are categorized into constitutive amino acids and free amino acids. Free amino acids enhance the taste and flavor of foods, thus affecting their palatability (Mustafa et al., 2007). The free amino acid analysis revealed that SF of WD-A had the highest aspartic acid levels, a key taste-contributing amino acid. In contrast, the seaweed from the control region was rich in glutamic acid and glycine, whereas SF of WD-B contained high levels of other amino acids (Table 1). Notably, taurine and tyrosine, known fatigue-reducing amino acids, were exclusively found in WD-A. Regarding the constitutive amino acids, WD-A had the highest histidine contents, but WD-B had higher levels than the control for all other amino acids (Table 2). Notably, histidine, which is an essential amino acid for infants but not adults, plays a role in hematopoiesis (Kim et al., 2017). Essential amino acids, which must be obtained from the diet because they are not synthesized endogenously (An et al., 2020), were most abundant in WD-B, followed by WD-A, and both samples had significantly higher levels than the control. WD-A was particularly rich in valine, whereas WD-B contained all essential amino acids except lysine. Thus, SF from Wando is an excellent source of essential amino acids.
Electronic tongue systems are capable of analyzing primary tastes such as umami (NMS), sweetness (PKS), bitterness (ANS), saltiness (CTS), and sourness (AHS) and have, thus, become prominent for food characteristic and taste profile analysis (Hong et al., 2020). Crucially, these systems provide objective taste measurements using non-destructive methods without pretreatment (Hong et al., 2021). Therefore, in this study, we compared SF taste patterns from WD-A, WD-B, and the control region using an electronic tongue (Fig. 3). The NMS score of WD-A was 8.8, which is significantly higher than those of WD-B and the control (by 1.9- and 2.0-times, respectively). AHS was also ≥1.8-times higher in WD-A than in the WD-B and control samples. In contrast, the control region scored highest for PKS (7.6) and ANS (7.7); WD-B showed a similar trend but with lower values, whereas WD-A showed significantly lower PKS and ANS values. CTS was similar for all samples. Notably, humans can discern taste differences when primary taste values differ by ≥2.0, and greater differences indicate stronger taste perception (Jo et al., 2012). The high umami and sourness values of WD-A are consistent with its high glutamic and aspartic acid levels, key contributors to umami flavor. In contrast, WD-B had high sweetness and bitterness values. In the DFA to identify patterns based on these results, the discriminant function first score (DF1) was 97.68%, and the discriminant function second score (DF2) was 2.32%. With DF1 values as the reference, WD-A clustered in the negative direction, while WD-B and control clustered in the positive direction, indicating a significant differentiation in taste patterns among the regions (Fig. 4). Overall these differences suggest that the marine origin, environment, and climate influence the taste of SF (Dong et al., 2017).
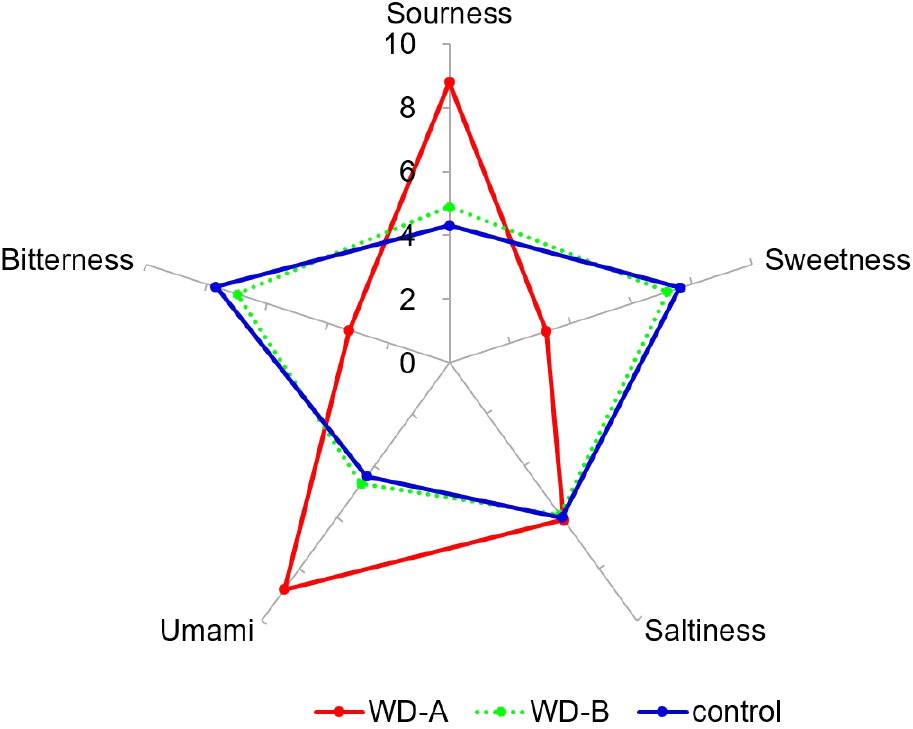
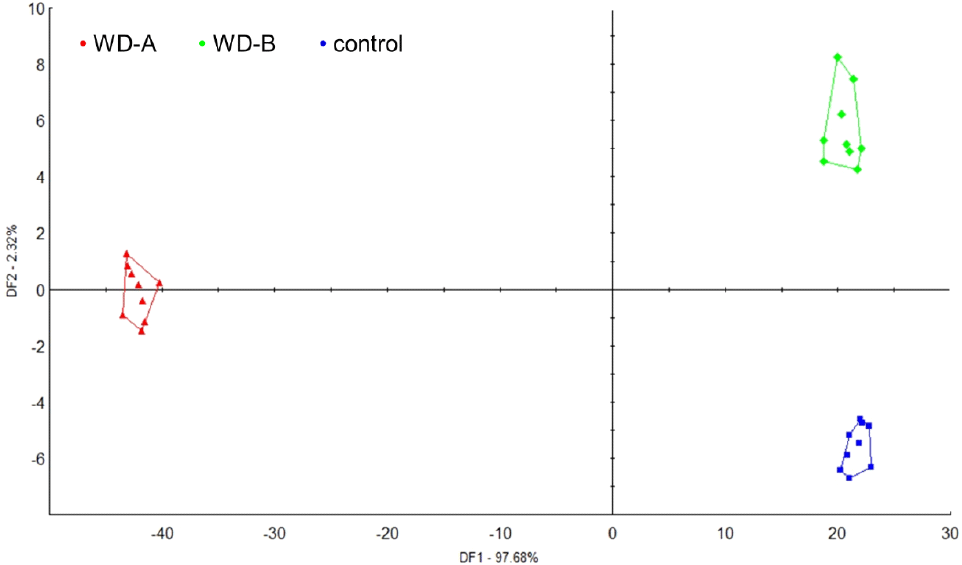
4. Conclusions
In this study, we assessed the nutritional property and taste characteristics of Sargassum fusiforme (SF) from two regions in Wando (Wando-eup, WD-A; Sinji-myeon, WD-B) and a control area (Jodo-myeon, Jindo: control), focusing on macronutrients, amino acids, and minerals. Additionally, taste patterns were evaluated using an electronic tongue system. The crude protein content was significantly higher in the WD-A and WD-B SF compared to the control regions, and ash content was also highest in the WD-A regions. Conversely, WD-A had the lowest carbohydrate contents and caloric values. Mineral analysis indicated that WD-A SF had high potassium, iron, and zinc contents, whereas sodium, phosphorus, and magnesium were more abundant in the control. Amino acid analysis showed that both WD-A and WD-B SF had higher levels of constitutive, free, and essential amino acids, with WD-B leading, followed by WD-A and the control. Notably, taurine and tyrosine, which are associated with fatigue reduction, were exclusive to WD-A. Taste evaluation demonstrated that WD-A SF was characterized by pronounced umami and sourness, whereas WD-B and the control were distinguished by heightened bitterness and sweetness. Therefore, the nutrient composition and taste profile of SF vary with regional marine conditions, and these findings provide valuable insights for future seaweed research in the Wando region.