1. Introduction
Commonly used browning inhibitors, such as sulfites, ascorbic acid, and its derivatives, are utilized during packaging and storage to delay the degradation period of fresh or minimally processed fruits and vegetables (Sridhar et al., 2021). In addition, costly yet effective technologies, like low-temperature utilization, modified atmosphere storage, and heat treatment, extend the shelf life of fruits and agricultural products. As a result, there is a pressing need for a safe, cost-effective, and eco-friendly method to preserve the nutritional value of perishable food items, including fruits.
Effectively managing agricultural and industrial waste biomass is crucial for sustainable production and consumption. To minimize environmental impacts and support a circular economy, it is essential to implement waste reduction strategies and utilize waste materials to produce bio-composites. With global egg production expected to exceed 90 million metric tons by 2030, it is critical to find alternative uses for eggshell waste, which contains primarily calcium carbonate along with proteins and minerals (Nys et al., 2001). One potential solution is to utilize eggshells in various applications, such as manufacturing biological catalysts and antimicrobials, enhancing culinary dishes, improving soil quality, and developing biocomposites (Balaz et al., 2021; Kulshreshthan et al., 2022; Vandeginste, 2021). To assess the effectiveness of biocomposites derived from eggshells, modifying the physical and surface characteristics of eggshells is crucial. Eggshell powder, which is cost-effective and renewable, is commonly used as a bio-filler in bio-composites (Bootklad and Kaewtatip, 2013; Ji et al., 2009). Studies have shown that incorporating eggshell powder in composites can enhance toughness, heat resistance, and degradation resistance compared to other fillers like calcium carbonate.
Gum Arabic, obtained from Acacia trees, is a natural gum with several functional qualities used in the food and pharmaceutical industries as an emulsifier, stabilizer, and thickening agent (Barak et al., 2020; Fallourd and Viscione, 2009). Despite its solubility in water and versatility, pure gum Arabic has limitations in food packaging applications due to its insufficient mechanical strength and barrier properties (Aphibanthammakit et al., 2018). Combining gum Arabic with bio-fillers like eggshell powder has the potential to improve its functionality and expand its uses in food packaging.
The transformation of agricultural waste biomass such as eggshells and gum Arabic into bio-composites establishes the groundwork for a future industry that will rely less on finite resources and promotes a more sustainable and resilient ecosystem. Therefore, the present study aimed at fabricating a gum Arabic-eggshell hybrid composite film using the solution casting method and characterized its morphological, mechanical, and thermal properties, as well as its degradability. In addition, the bio-composite was evaluated for application in postharvest storage of tomato fruits.
2. Materials and methods
Bromothymol blue, dinitro salicylic acid, 2,6-dichlorophenol indophenol and D-galacturonic acid were purchased from SRL Pvt. Ltd (Maharashtra, India). Metaphosphoric acid, pectin, polygalacturonic acid, sodium hydroxide, sodium phosphate, sodium trichloroacetic acid and phosphoric acid were obtained from Sigma-Aldrich (Gillingham, UK).
Eggshell waste from the Landmark University, (Omu-Aran, Kwara State, Nigeria) cafeteria was properly cleaned in distilled water to remove any related pollutants before being oven-dried at 50°C to constant weight for 6 h. The dried eggshell debris was ground into a fine powder and sieved through a 180 μm mesh sieve before being stored in an airtight bag inside a desiccator.
Using a magnetic stirrer, half a gram (0.5 g) of eggshell powder (EC) was dissolved in 5 mL of distilled water, and the solution was sieved through a 100 μm mesh size. In a separate beaker, a 15% (w/v) gum Arabic (GA) solution was prepared by heating 15 g of GA powder in 100 mL of solution at 50°C for 45 min on a magnetic stirrer. To remove particle matter connected to the gum Arabic crystals, the solution was filtered through a muslin cloth. The EC-reinforced GA composite was fabricated by adding the EC powder solution into the GA solution and stirring constantly for 30 min at 70°C. Glycerol (3 mL) was added to the solution (as a plasticizer), which was vigorously agitated to achieve a homogeneous mixture. The GA-EC composite mixture was cooled to room temperature before being degassed for 20 min under a vacuum (0.09 MPa), transferred into a petri dish, and placed inside a forced air oven at 50°C for 72 h.
We employed a digital micrometer (Mitutoyo, Japan), to ascertain the thickness. The measurements were conducted at 10 randomly selected points on each sample, and the mean value was utilized to describe the thickness. The transparency of the sample at a wavelength of 560 nm was measured using spectrophotometry. The extent of the sample’s dissolution in water was assessed using the method described by Pavin et al. (2010). The film’s moisture content was determined by calculating the difference in mass before and after drying it to a constant mass in a hot air oven at 100°C, using Equation (1).
Where W1 and W2 refer to the initial and final weights of the film before and after drying, respectively.
The water vapor permeability (WVP) of each film was determined using a modified version of the ASTM procedure E96-90, as documented by Wu et al. (1995). A 68 mm diameter film sample was shaped into a sphere and positioned on top of the opening of a cylindrical cup. The cup contained a specific quantity of anhydrous CaCl2, which had 0% relative humidity. This was done to ensure a consistent 75% disparity in relative humidity between the samples. The airtight cylindrical container was then placed in a desiccator and exposed to a saturated sodium chloride solution at a temperature of 25°C and a relative humidity of 75%. Weight measurements were taken at eight-hour intervals following a two-hour period of enabling the equipment to stabilize. The rate of change in cup weight, measured to the closest 0.0001 g, was determined as a function of time using linear regression analysis with a r2 value greater than 0.99. The water vapor transmission rate (WVTR) was calculated by calculating the ratio of the slope to the exposed area of the sample. Conversely, the WVP was determined using Equation (2).
S represents the saturated vapor pressure of water at 25°C, R1 represents the relative humidity in the desiccator, R2 represents the relative humidity in the permeation cell, and D refers to the thickness of the sample. The analysis was replicated thrice.
The tensile strength of the sample was measured by applying a force of 100 N at a rate of 10 cm/min at room temperature, using a Universal Testing Machine (Hounsfield Series S, Redhill, UK) according to the ASTM standard D882, as described by Rahman and Jamalulail (2012). The data from the tensile strength analysis was utilized to calculate the elongation at the break of the sample. Tensile stress was calculated using Equation (3).
The samples were affixed to stubs using double-sided sticky tape and then coated with a 20-nm-thick layer of gold using a sputtering process. Examination and observation were conducted using a scanning electron microscope (Tescan Clara, Cambrige, UK).
FTIR spectra of the samples were recorded using the attenuated total reflection (ATR) mode, with 32 scans. The spectral scanning was conducted with an FTIR spectrometer (JASCO, Easton, MD, USA) operating at a frequency range of 400-4,000 cm−1 and a resolution of 2 cm−1.
X-ray diffraction was measured using a Rigaku X-ray diffractor unit (Freiberg, Germany). The unit was equipped with a Cu kα radiation source with a voltage of 40 kV and a current of 30 mA. The wavelength of the radiation used was 1.54 A°. The XRD scan was conducted in the angular range of 2.0 to 80.0° (2θ). The scanning speed was set at 2 degree/min. The integral intensity of the chosen velocity was determined using Rigaku profile-fitting analysis (Rigaku XRD-6000 software version 4.1).
Thermogravimetric analysis (TGA) was conducted using a Perkin Elmer Pyris 1 TGA analyzer (Waltham, MA, USA). Briefly, 5 mg of sample was subjected to heating in a temperature range of 30-800°C, with a heating rate of 10°C/min, under nitrogen atmospheric.
Approximately 5 mg of each sample was placed into sealed aluminum pans. The DSC analysis was conducted using a 2920 Modulated DSC [TA Instrument (New Castle, DE, USA) under a nitrogen environment]. The temperature range for the analysis was from 30°C to 250°C, with a heating rate of 10°C/min.
The degradability of the GA-EC composite films was evaluated by soil burial according to the method of Pavin et al. (2010) described by Oluba et al. (2021).
For this experiment, a sample of twenty-four (24) fully grown, unripe green tomato fruits with identical sizes and shapes and no visible lesions or post-harvest illnesses were selected. The fruits underwent a thorough cleansing process using distilled water and were thereafter left to dry in the air for a duration of 6 h. The fruits were subsequently divided into three groups, each consisting of eight. These groups were labeled as control, GA-EC0.5, and GA-EC1.0, depending on the fruits’ weights and sizes. The tomato fruits in the GA-EC0.5 and GA-EC1.0 groups were carefully wrapped with their respective composite films, ensuring full covering of the whole surface. The fruits devoid of any film packing were utilized as a control. The fruits were stored at an optimal temperature of 25±2°C for preservation. In addition, the fruits were regularly assessed on days 0, 5, 10, 15, and 20 for changes in weight and any alterations in physiological and biochemical quality indices.
After the treatment period, tomato fruits from each group were individually blended and strained using Whatman™ No. 1. The resulting liquid was then stored in clean, sterile bottles at a temperature of 4°C until it was needed for further analysis.
The fruits in each treatment group were individually weighed using an electronic weighing scale (Sartorius BSA224S-CW, Germany, precision of 0.01) on day 0 (W0) and days 4, 8, and 12 (Wf). The percentage weight reduction was determined using Equation 4.
The pH of the solution obtained after diluting 10 mL of the filtrate with 50 mL of distilled water was ascertained utilizing an electronic pH meter (H12210, Woonsocjet, RI, USA).
The lycopene content of the tomato fruit extract was quantified following the method described by Oluba et al. (2022).
The activity of polyphenol oxidase (PPO) was determined by observing the formation of a colored benzoquinone solution from catechol, as previously described by Oluba et al. (2022).
The assay for pectin methyl esterase activity was conducted using a method initially developed by Hagerman and Austin (1986), and subsequently modified by Ali et al. (2022). The mango fruit sample was mixed with 1 g of NaCl (8.8%) solution and homogenized before being centrifuged at 1,000 ×g for 20 minutes at a temperature of 4°C, and the resulting supernatant was labeled as the enzyme mixture. Subsequently, the enzyme mixture was combined with 100 L of a 1% solution of bromothymol blue and 1 mL of a 0.5% pectin solution. The resulting mixture was left undisturbed for a duration of 10 min, after which the absorbance was measured at a wavelength of 620 nm. The enzyme activity was quantified in units per gram of protein.
The analyses were conducted three times, with the results presented as mean±SD. Tukey’s test with a 95% confidence interval was used to compare means in GraphPad Prism 8.4.3 (Boston, MA, USA), which also included analysis of variance. We used OriginPro 2016 for our charting requirements.
3. Results and discussion
The data on the physico-mechanical characteristics of the GA-EC bio-composite film is presented in Table 1. From the results, moisture, transparency, water solubility, and WVP of the film were significantly (p<0.05) lower in the GA-EC1.0 composite compared with GA-EC0.5. There was no significant (p>0.05) difference in film thickness and elongation at the break between GA composite films containing 0.5 g and 1.0 g EC. The tensile strength was much higher in the GA-EC1.0 composite than in the GA-EC0.5 composite (Table 1). Data from this study revealed that including EC to GA at 1 g concentration to form a GA-EC1.0 composite film resulted in improved mechanical properties of the composite film, particularly tensile strength and elongation at break, which correlates with the level of incorporated EC. This observation could be linked to the EC potential improvement in the interfacial adhesion between the EC particles and the GA film matrix, thus, restricting movement within the GA matrix chain while increasing the macroscopic rigidity of the GA-EC composite. According to Bootklad and Kaewtatip (2013), the organic components on the EC surface may also act as coupling agents, facilitating the dispersion of the EC inside the GA matrix to build a continuous network matrix.
Results are means±SD of triplicate determinations.
Different superscripts (a,b) in the same row are significantly different at p<0.05 by Student t-test.
In this study, the evaluation of film barrier qualities evaluated by WVP is critical for determining the performance of films designed for food packaging purposes. The potential ability of films to prevent moisture or vapor absorption could considerably contribute to the film’s ability to preserve the freshness and safety of food, thus extending its shelf life. The WVP of the GA-EC composite films fabricated in this investigation reduced as the amount of EC powder added increased. This is consistent with the findings of Feng et al. (2014). The use of EC in GA film impeded moisture diffusion through the film due to the insolubility of EC. In addition, the organic molecules on the EC surface aid adhesion between the EC particles and the GA film matrix, hence, improving the degree of dispersion of EC into the film matrix and making the resulting gum GA-EC composite film more rigid and stiff. Furthermore, as demonstrated by the SEM results in this work, the existence of voids in the surface microstructure of EC powder contributes to its ability to adsorb moisture onto its surface.
The SEM image of GA powder at 250× magnification revealed unevenly formed rough surface particles (Fig. 1A). The rough surface morphology of GA particles is beneficial for generating a highly viscous aqueous solution. Similarly, the microstructural appearance of the EC particles is non-homogeneous and uneven in size. The particle shape is random, and the SEM micrograph shows that the EC comprises crystalline microporous particles that are randomly ordered in a porous microstructure (Fig. 1B). The sample surface was found to be rough, which implies it may easily adhere to other materials and, as a result, can be utilized as a biodegradable filler. Due to the non-complete dispersibility of the EC powder into the aqueous viscous milieu of the GA solution, the surface microstructure of the GA-EC composite films revealed the presence of certain knobs, which appeared to be more noticeable in the GA-EC1.0 composite (Fig. 1D) compared to GA-EC0.5 (Fig. 1C). This finding could be attributed to decreased interface adhesion forces between GA and EC powder. Consequently, a higher EC powder concentration contributes to the deformation of the structural integrity of the GA-EC composite film. This finding is similar to the previous report by Jiang et al. (2018) and Sun et al. (2014), which showed that when corn starch is combined with EC powder, the surface smoothness diminishes with increasing EC concentration.
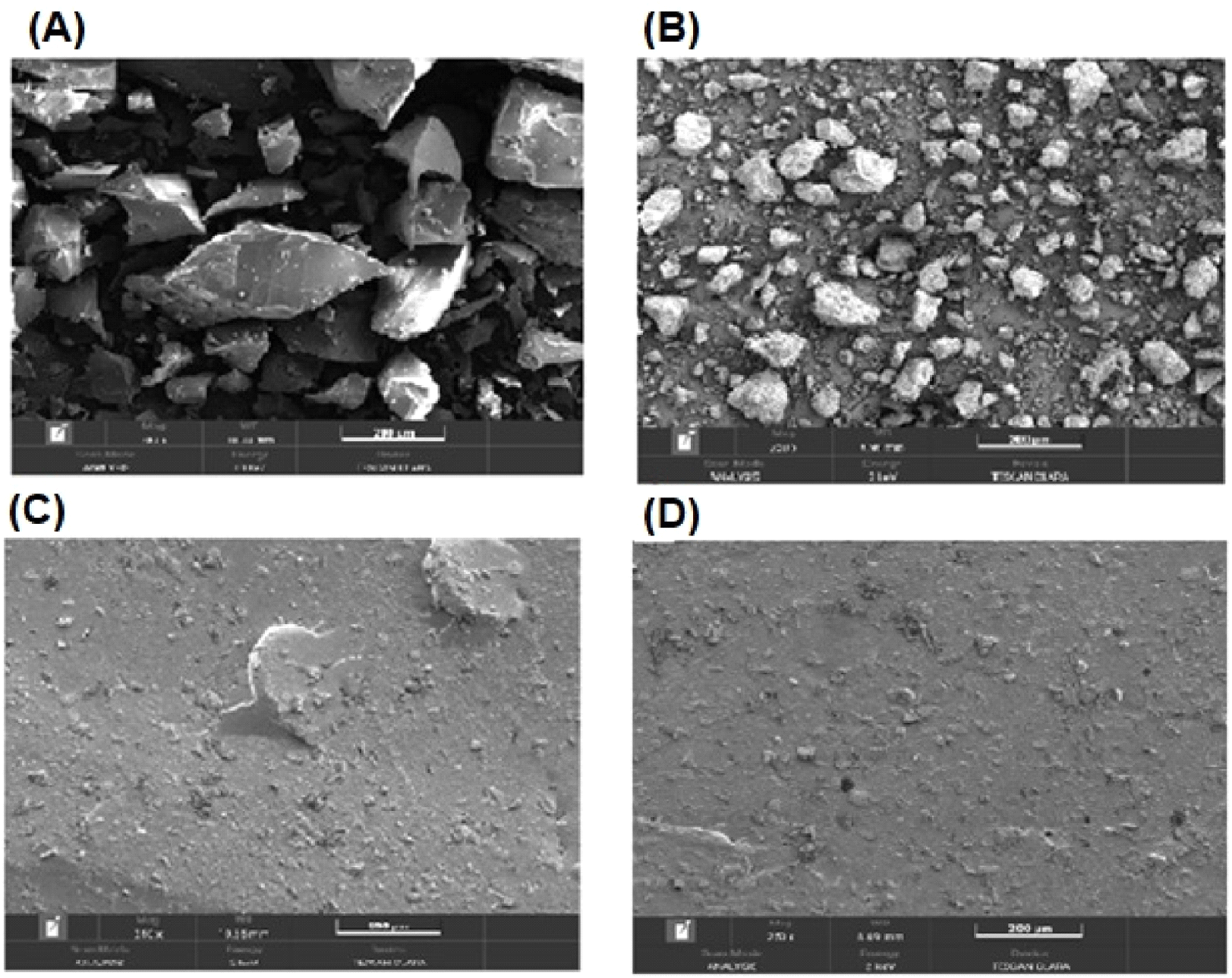
The major absorption bands indicating the functional groups present in GA, EC, and GA-EC composite films within the frequency range 4,000 cm−1 to 600 cm−1 are shown in Fig. 2. GA showed major characteristic peaks at 3,332.4 cm−1 for O-H stretching, 2,921.6 cm−1 for C-H stretching, 1,601.6 cm−1 for C×O stretch of Amide-I, 1,409.7 cm−1 for C-H stretching, 1,019.2 cm−1 for N-H stretching of Amide-II and C-N stretch of Amide-III band, and 804.2 cm−1 for C-H stretching (Fig. 2). EC displayed characteristic absorption peaks at 1,796.4 cm−1, 1,410.4 cm−1, 872.6 cm−1, and 711.6 cm−1. The GA-EC0.5 and GA-EC1.0 composite films have similar peak patterns. However, the absorption peaks in the GA-EC composite films were longer and sharper than those of GA. The absorption bands at 3,360-3,000 cm−1 of GA and GA-EC composite films correspond to the NH2 of primary amine and the OH group related to the pyranose hydroxyl group. According to Daoub et al. (2018), the presence of sugars such as galactose, arabinose, and rhamnose could explain the occurrence of weak bands at 2,920 cm−1 suggestive of C-H stretching of aldehydes. The most notable absorption peak seen in EC occurred at 1,401 cm−1, corresponding to the presence of carbonate (CO3−2) ions. Furthermore, the two peaks occurring at 711 cm−1 and 872 cm−1 are related to asymmetric stretching of the C-H band, out-of-plane bending, and in-plane bending stretching vibrations of CO3−2 ions. Although the carbonate peaks were visible in the GA-EC composite films, their intensity was diminished. The formation of the GA-EC composite is thought to increase the number of hydrogen bonds between GA and the EC particles (Sivakumar et al., 2022). This could lead to the vibrational frequency of the pyranoid ring skeleton shifting the absorption bands to a higher wavelength and lower intensity.
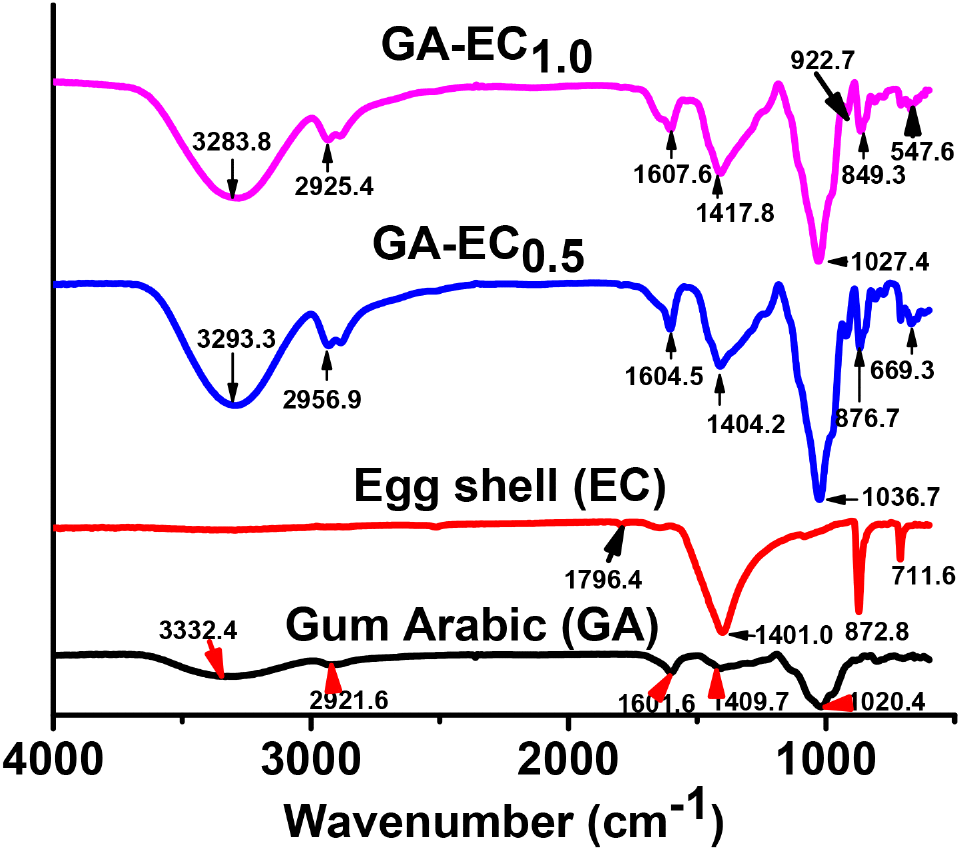
The X-ray diffraction chromatogram of GA revealed a semi-crystalline appearance with distinct crystalline peaks. The highest peaks were at 18.64° and 29.95° (Fig. 3A). The ultrastructure and crystal formation of EC particles revealed three distinct peaks in the X-ray diffraction spectra. The first band peaks occurred between 23.18° and 29.54°, and the second band peaks occurred between 36.1° and 39.48°. The third band had peaks at 43.24° to 47.62° (Fig. 3B). These peaks were significantly influenced by the development of the GA-EC composite film (Fig. 3C and 3D). The main peaks in GA were identified at 18.64° and 29.95°, which could be attributed to the strong interaction between the hydroxyl groups in GA molecules. There were significant differences in the diffraction peaks between the pure GA and EC powder and the GA-EC composite films. The crystalline peaks observed in neat EC powder disappeared in the GA-EC composite films, demonstrating the high compatibility between GA and EC powder. This observation is consistent with the reports of Dweiri (2021) and Sun et al. (2022).
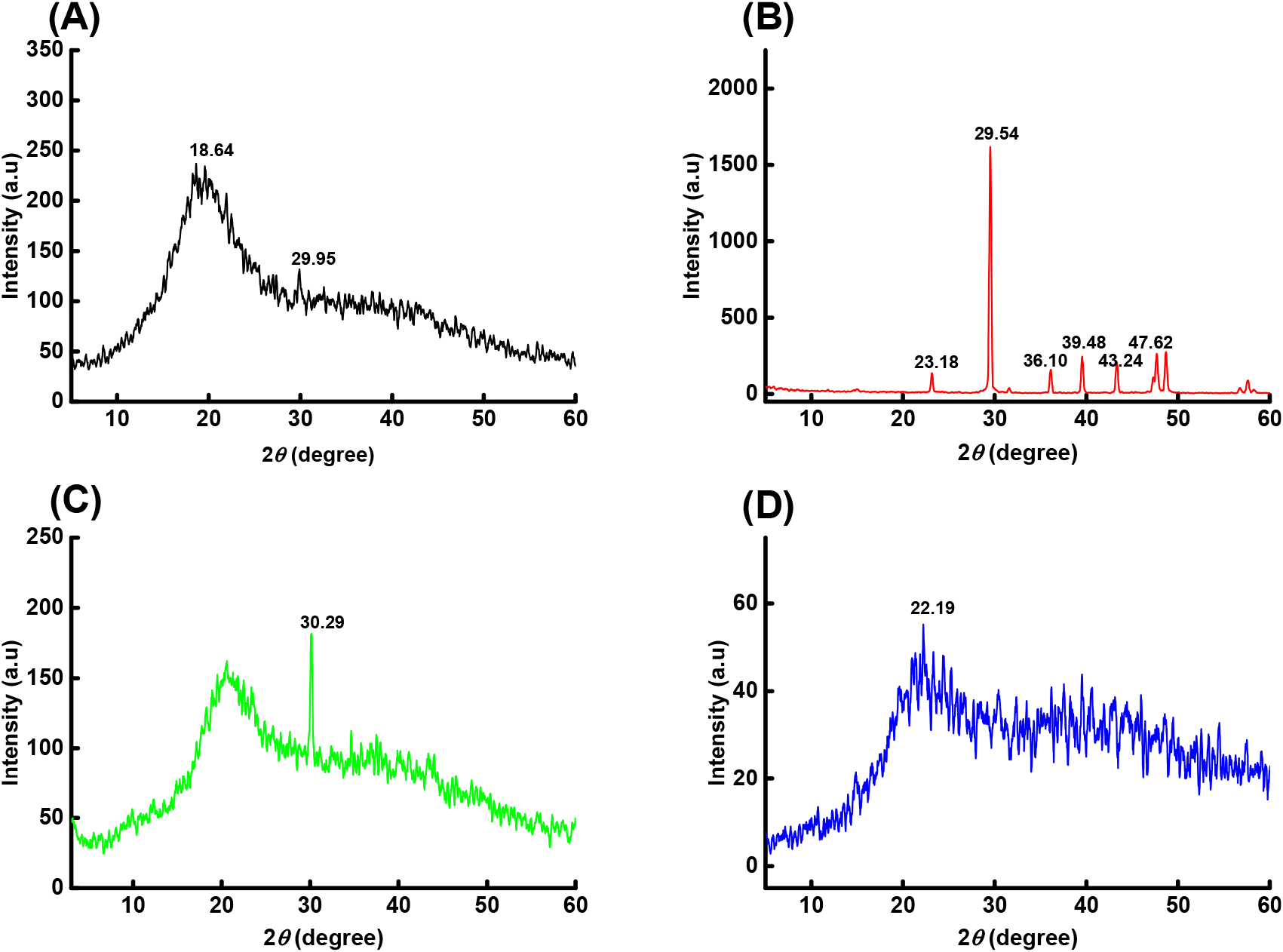
Fig. 4 depicts the TGA and derivative thermogravimetric analysis (DTG) chromatograms of GA, EC, and GA-EC composite films. The degradative profile of GA was two-stage. Up to a temperature of 123.0°C, a 4.1% weight loss was observed. At 292.3°C, the weight decreased by approximately 33.8% in the second phase of the degradation profile (Fig. 4A). The EC degradation profile maintained a consistent mass over the full temperature range of 30°C to about 700°C where about 9.0% decrease in mass was observed (Fig. 4B). The deterioration profiles of the GA-EC0.5 and GA-EC1.0 composite films were four stages. The weight of the GA-EC0.5 composite decreased by 6.6%, 32.5%, 58.0%, and 77.9% at 152.6°C, 242.5°C, 297.8°C, and 697.8°C, respectively (Fig. 4C). At 163.2°C, 243.5°C, 300.9°C, and 706.7°C, the GA-EC1.0 decomposed by 6.7%, 22.5%, 57.1%, and 75.2%, respectively (Fig. 4D). A decrease in weight of about 4.1% was observed in GA at 123.0°C which may be attributed to water loss. At 292.3°C, a further reduction of 33.8% in weight was noted; this may be related to eliminating the functional groups in GA, such as OH, CHO, CO, and COOH, which could explain this drop. The mass of the EC was constant over a temperature range from 30.0°C to 700°C. Degradation-related weight loss of approximately 32.1% was observed in the GA-EC composite film at 242.5°C. Further loss of organic components inside the composite molecules resulting in a 58% weight reduction was observed above 300°C. The degradative temperatures of GA-EC composite films comprising 0.5 g and 1.0 g EC powder, respectively, were higher than those of neat GA (292.3°C).
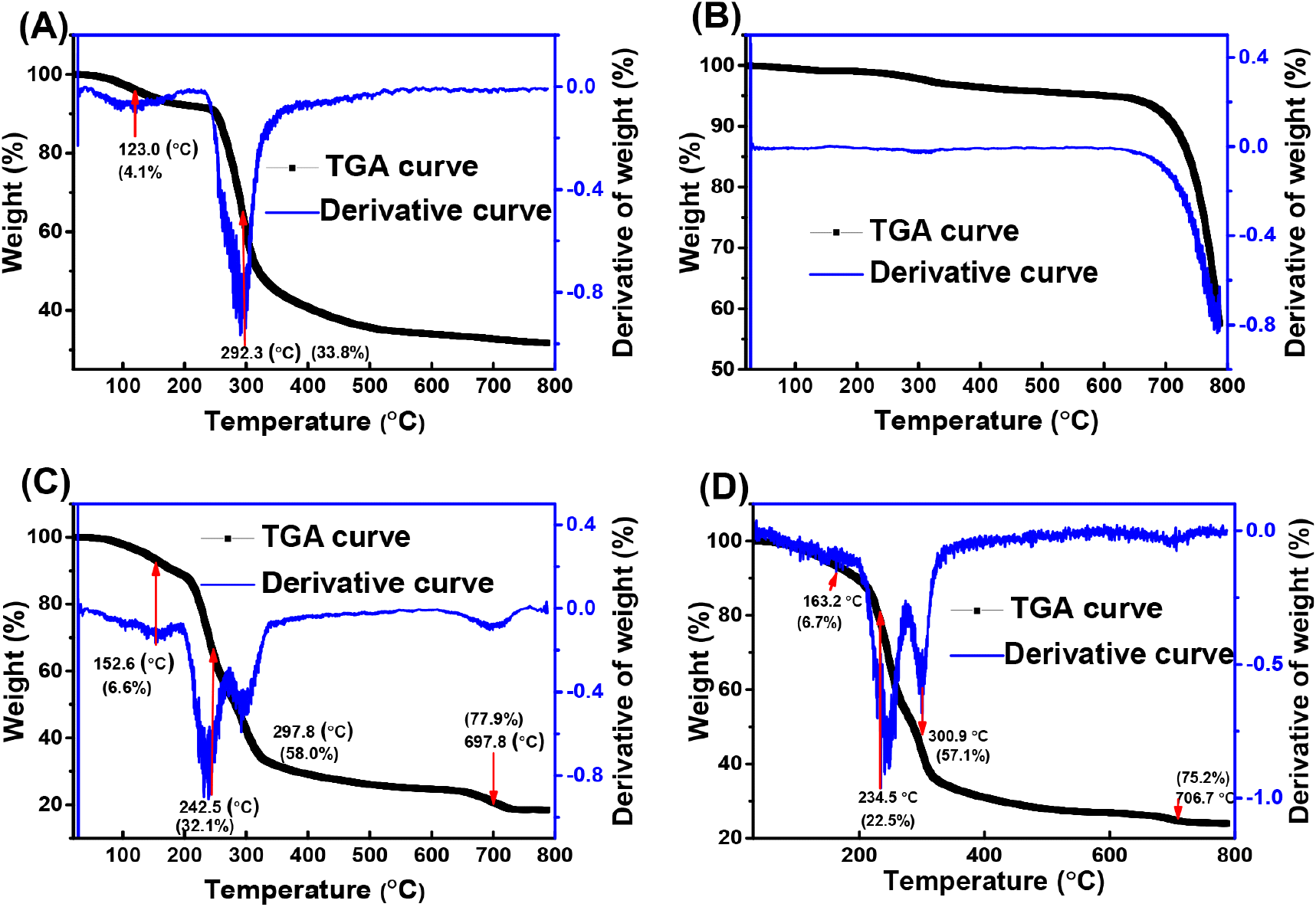
Fig. 5 shows the DSC chromatograms of GA, EC powder, and GA-EC hybrid composite film. The onset temperature of GA occurred at 177°C while the melting temperature was 178.7°C. EC powder remained thermally stable over the entire temperature range evaluated. On the other hand, the GA-EC hybrid composite on set temperature was 125.8°C, and the melting temperature was recorded at 138.1°C. This study’s data on the thermal stability of GA-EC composite film revealed that the weight loss at temperatures below 300°C was less than that achieved for GA. Furthermore, the thermal degradation of the composite films was reduced more in the film containing more EC powder (GA-EC1.0). The inclusion of EC powder improves the films’ thermal stability. It could be argued that the polymeric chain of GA cannot withstand high temperatures. The dispersion of EC particles into the GA matrix, on the other hand, gradually increases the thermal stability of the GA-EC composite film. EC powder can absorb more heat energy (as shown by TGA measurements), lowering the energy acquisition of the composite film. Furthermore, the improvement in thermal stability of the GA-EC composite film observed in this study could be explained by the increased interfacial adhesion between the particulate matter of the EC powder and the GA film matrix caused by the presence of certain organic molecules on the EC surface, which contributes to the degree of compactness of the composite molecules. As previously reported by Vonnie et al. (2022), EC could improve hydrogen bond formation and minimize the number of active sites accessible for water absorption, reducing interstitial space within the film network structure and increasing the film’s compactness.
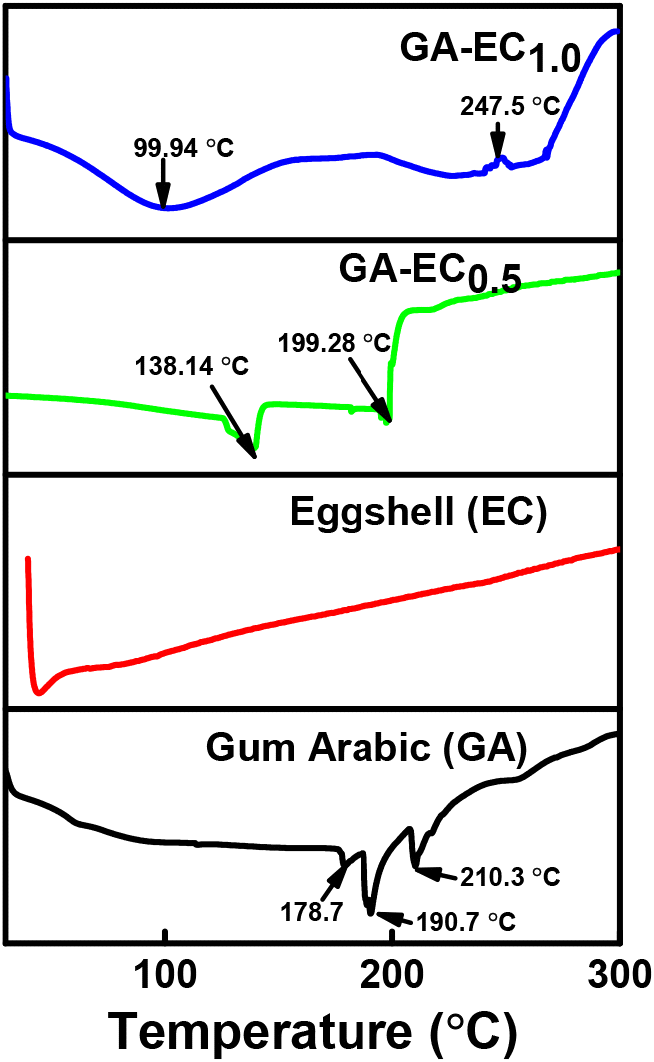
The GA-EC0.5 composite film had a mass reduction of 64% compared to the starting mass, whereas the GA-EC1.0 composite film had a weight reduction of 40.5%. Given the slow breakdown of natural EC waste in the soil, the degradability data revealed in this study is encouraging. In this investigation, increasing the EC content from 0.5 g to 1.0 g reduced the deterioration of the GA-EC composite film by 23.5%. On the other hand, no appreciable change in mass was observed in the eggshell (control) after a 25-day soil burial experiment (Fig. 6). The increased compact packing (density) between GA molecules and EC powder may explain the decreased decomposition rate in the composite with more EC. The organic compounds on the surface of EC powder aid in this process (Jiang et al., 2018).
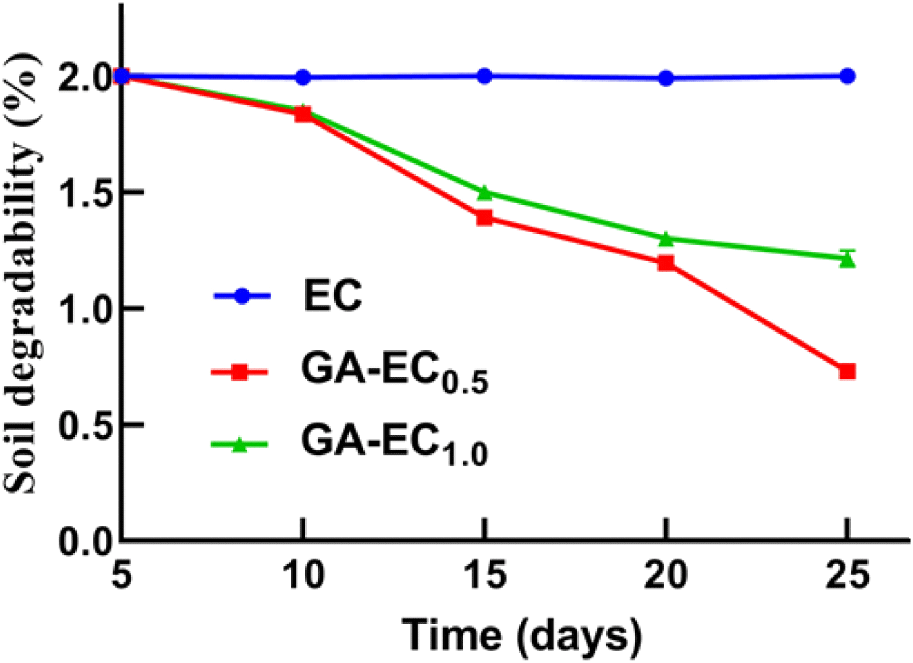
Postharvest fruit and vegetables are prone to dehydration, leading to a decline in the quality and market value of fresh produce (Hasan et al., 2019). Applying an edible membrane to wrap or coat a product can effectively minimize moisture loss and prolong its shelf life. In the present study, weight loss commenced on the 10th day of storage, as anticipated. There was a significant (p<0.05) disparity in weight loss between the control group (17.08%) and the groups with fruit wrapped in GA-EC0.5 (5.87%) and GA-EC1.0 (4.52%). The control fruit exhibited a consistent decrease in weight during the storage period. By day 20, the control fruit had lost 26.17% of its initial weight, 30% and 43.3% greater than the weight loss observed in the GA-EC0.5 and GA-EC1.0 wrapped fruit, respectively (Fig. 7A). The significant rise in weight loss seen in the control fruits in this study can be attributable to insufficient barrier characteristics and increased oxygen permeability. In this work, the GA-EC composite film was utilized as a protective barrier to provide an environment with decreased oxygen levels and limited moisture permeability for the packaged fruits. The decrease in weight loss observed in the fruit wrapped with GA-EC composite films demonstrates the semi-permeable characteristics of the GA-EC composite film, effectively reducing the transfer of moisture and mass from the fruit surface. Kang et al. (2021) conducted a study where they noticed a comparable decrease in weight in strawberry fruit that was covered with a cellulose nanocrystal-reinforced gum Arabic film. They observed that the coated fruit experienced a lower weight loss than the uncoated control group. Water is crucial for the shelf life, quality, and market value of fruits (Kumar et al., 2023). Minimizing water loss is essential, and our research demonstrates that applying a starch coating effectively reduces water loss in stored tomatoes.
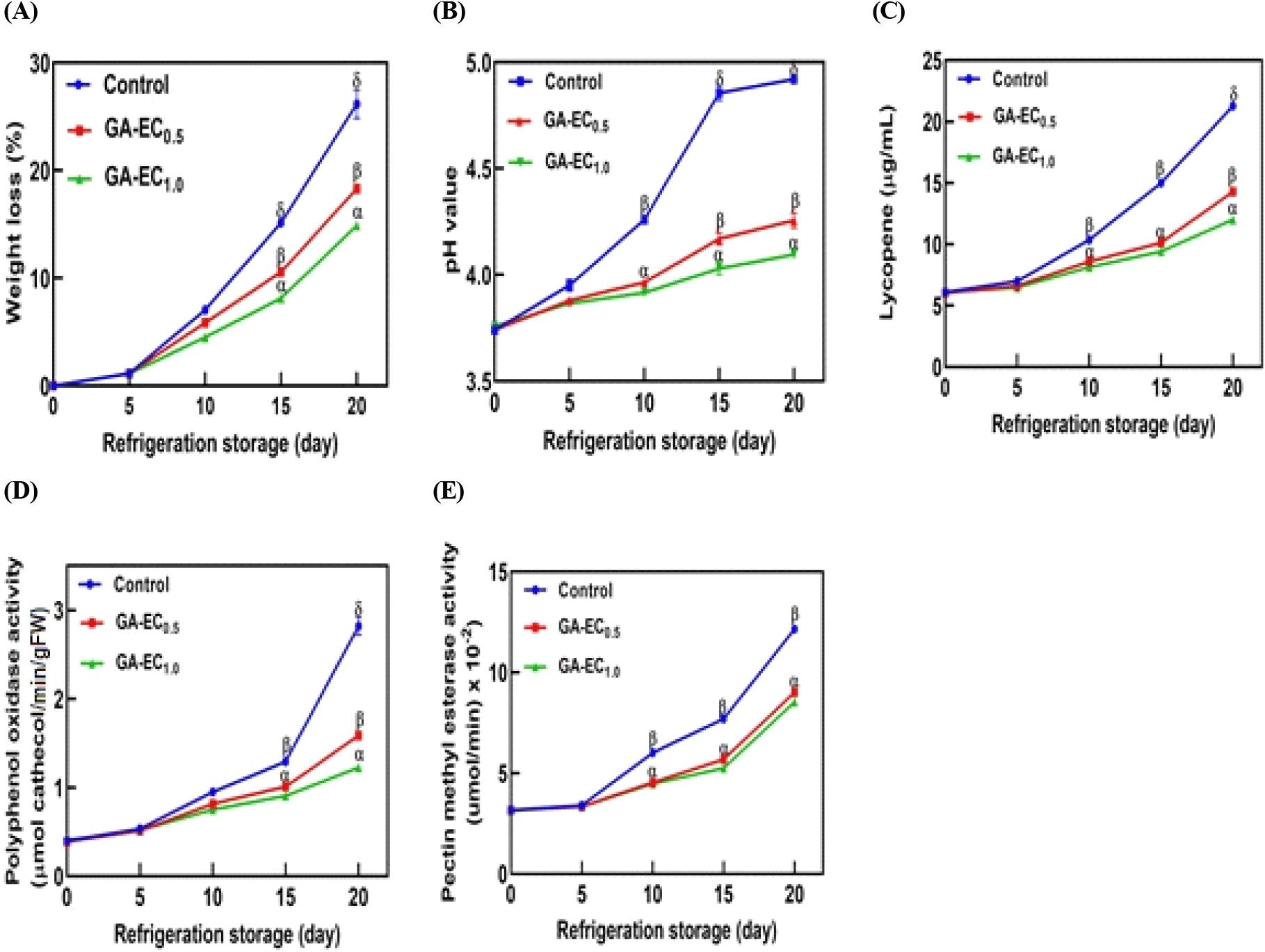
The increase in pH observed during the ripening of the fruit is caused by a decrease in acidity, which results from the conversion of organic acids into sugars that are used as respiratory substrates. The tomato fruit contains citric acid as its primary organic acid (Li et al., 2022). On the tenth day, the pH of the control fruits was 6.8% and 8.0% higher than those wrapped in GA-EC0.5 and GA-EC1.0, respectively (Fig. 7B). At the end of the storage period, the pH (4.92) of the control tomato fruits decreased by 13.4% and 16.7% in the GA-EC0.5 (4.26) and GA-EC1.0 (4.10), respectively. The rise in pH levels of both the control and GA-EC-treated fruits is directly linked to the rapid rate of respiration, which is facilitated by the presence of oxygen and other respiratory substrates. Conversely, the GA-EC composite film’s semi-permeability allowed for a decreased oxygen environment, resulting in a deceleration of respiration in the treated fruits. Our findings are consistent with the report of the study conducted by Emamifar and Bavaisi (2020) and Jahanshahi et al. (2018), which demonstrated that the application of tragacanth and Aloe vera gum coatings on strawberries and apples, respectively, effectively inhibited the increase in pH during the storage period. The fruit’s acidity influences its quality and acceptance (Kyriacou et al., 2018). Furthermore, Ju and Curry (2005) observed that the pH of apples coated with maize oil was elevated compared to the control group. The findings of the current investigation closely aligned with their observations.
The concentration of lycopene in the tomato fruits increased in all treatments over the entire storage period, as seen in Fig. 7C. Starting on day 10, a statistically significant (p<0.05) disparity in lycopene concentration was noted between the tomato fruits wrapped with GA-EC and the control group. The concentration of lycopene in the control group, which was initially measured at 10.36 μg/mL, was reduced by 21.4% in the fruits wrapped with GA-EC. The GA-EC0.5 and GA-EC1.0-wrapped fruits reduced 32.4% and 37.2%, respectively, in lycopene compared to the control (10.14 μg/mL) on the 15th treatment day. On the 20th day, the lycopene level in the control tomato fruits was 32.8% and 43.5% greater than the content in the GA-EC0.5 and GA-EC1.0-treated tomato fruits, respectively. The lycopene content in the control tomato fruits was measured at 14.31 μg/mL. The red color of ripe tomato fruit results from the gradual generation of lycopene as it matures (Li et al., 2021). The accumulation of lycopene in tomato fruits is enhanced by increasing the activity of its biosynthetic enzymes and inhibiting the enzymes responsible for accelerating the production of cyclic carotenoids. The rapid increase in lycopene levels in the control (unwrapped) fruits in this study could be attributed to their accelerated ripening process. The decrease in lycopene concentration in tomato fruits that were covered with GA-EC composite films in this study aligns with the findings of Taher et al. (2020) and Ali et al. (2013), who observed that coating of 10% GA hindered the synthesis of lycopene without causing any negative impacts on the quality of the tomato fruits. This indicates that the concentration of lycopene in the treated tomato fruits is adequate to effectively function as an antioxidant in the diet. Similarly, Dávila-Aviña and colleagues (2014) discovered that the application of carnauba and mineral oil waxes separately caused a noticeable delay in the accumulation of lycopene in pink tomatoes during a 28-day storage period, when compared to tomatoes that were not coated.
Ripening in tomato fruits is accompanied by increased PPO activity, as well as a change in color from green to red. In line with this fact, the PPO activity recorded in this study showed an increasing trend with the storage period (Fig. 7D). However, beginning from day 10, the enzyme activity in tomato fruits wrapped with GA-EC0.5 (1.01 μmol · catechol · min−1 · g−1FW) and GA-EC1.0 (0.91 μmol · catechol · min−1 · g−1FW) composite film was significantly lower compared to control [1.30 (0.91 μmol · catechol · min−1 · g−1FW)]. At the termination of the storage experiment, a decrease of about 43.6% and 56.4% in PPO activity was recorded in GA-EC0.5 and GA-EC1.0 treated fruits, respectively. This observation agrees closely with Oluba et al. (2022) who reported a reduction in PPO activity in tomato fruits coated with keratin-starch composite impregnated with polyphenolic-rich extract from avocado peel. PPO catalyzes the conversion of phenolics into highly reactive electrophilic molecules (quinones), which covalently crosslink with other cellular elements to produce adducts (the brown color) (Kroll et al., 2003). The production of these adducts is believed to be the most damaging consequence of PPO in postharvest storage and food processing technology. The low-oxygen-modified atmosphere created on the fruits’ surface due to GA-EC composite film may be partly responsible for the reduction in PPO activity. This assumption is premised on the fact that the browning process in fruits catalyzed by PPO is oxidatively mediated.
The effects of the GA-EC composite film on the activity of PME of tomato fruits stored at 4°C are shown in Fig. 7E. The PME activity increases with storage duration across all treatments. Specifically, the untreated tomatoes (control) showed significantly (p<0.05) higher PME activity compared to the GA-EC-wrapped tomatoes. This finding could suggest a possible inhibition of PME activity by GA-EC composite film. Although the process of cell wall softening during ripening varies between species and cultivars, it is heavily influenced by the modulating activities of cell wall modifying enzymes including PME, which have been identified as one of the major pectin-degrading enzymes in fruits (Defilippi et al., 2018). The cell wall must be stiff and robust to provide structural support for the fruit and to endure anisotropic cell expansion in a controlled manner. Thus, the downregulation in PME activity observed in the GA-EC-wrapped fruits in this study is desirable for extending tomato fruit shelf life. In this investigation, the inhibitory activity of the GA-EC composite film on PME activity could be attributed to the formation of a modified atmosphere on the fruit’s surface. This modified atmosphere restricts the release of CO2 from the high respiratory rate during ripening, limiting ethylene synthesis and, as a result, the cell wall disintegration by PME.
4. Conclusions
The purpose of this study was to see how the addition of EC as a filler to GA in the development of a GA-EC composite film influenced the film’s physicochemical, mechanical, and thermal properties. GA-EC composite film with more EC powder (GA-EC1.0) demonstrated better mechanical and barrier properties than GA-EC0.5 composite film. The level of EC powder had a significant impact on the surface shape and functional groups of the composite films. The thermal stability of the composite film improved as the EC content increased. Overall, the use of EC powder as a bio-filler in bio-composite technology offers a high potential for increasing the mechanical, barrier, and thermal characteristics of films without the use of a crosslinking agent or other modification. Furthermore, when the GA-EC composite was used as a covering for tomato fruits and stored at 25±2°C for 20 days, it effectively reduced weight loss, prevented an increase in pH, and decreased lycopene content, polyphenol oxidase activity, and pectin methyl esterase activity. The GA-EC composite film is believed to have a considerable effect on the transport of moisture and gas mass over the surface of the tomato fruit. This, in turn, influences the activity of important enzymes in the ripening process. Nevertheless, additional research is necessary to validate this claim and comprehensively evaluate the influence of the film on sensory characteristics and consumer acceptance. Based on the degradability results achieved in this study, using EC powder as a bio-filler in bio-composite technology provides a value-added solution and a sustainable waste management strategy.