1. Introduction
The postharvest fruit storage has become an essential field of research (Zhao et al., 2024), influenced by the need to maximize the quality and durability of agricultural products. This is necessary to fulfill the demands of the globalized market (Abdullah et al., 2024). In the current context, sweet lime (Citrus limetta) is a widely consumed fruit due to its refreshing sensory profile (Suri et al., 2022) and bioactive compounds. These include phenols, flavonoids, carotenoids, and vitamins such as ascorbic acid (B’chir and Arnaud, 2023). The concentration of bioactive compounds varies depending on various factors such as crop type, maturity stage, and storage conditions (Hashemi et al., 2017).
However, this fruit is highly perishable, reflected by its short shelf life compared to other fruits. Such a disadvantage is a consequence of the non-climacteric nature of the fruit, which is sensitive to temperature changes, which accelerates its deterioration process (Kandegama, 2021). Temperature plays a critical role in fruit conservation, and low temperatures have been the subject of increasing interest due to their potential benefits in quality conservation (Brasil and Siddiqui, 2018). Furthermore, the temperature effect is associated with several impacts on the fruit, ranging from microbial development, physical impacts, and infection during postharvest storage (Panwar et al., 2023).
Low-temperature storage can reduce respiration, preserve nutrient content, and delay spoilage (Tsaniklidis et al., 2014). The appropriate low-storage temperature to avoid chilling injury of citrus fruits varies significantly among their different varieties (Morales et al., 2024). Further, several studies suggest storing the fruit at temperatures between −10°C and 15°C prolongs their shelf life and preserves the quality of lemons, mandarins (Morales et al., 2024), and oranges (Habibi et al., 2023).
In this context, scientific and academic interest in finding the best conditions to preserve the quality of fruits for a more extended period has increased (Pagar et al., 2023). This interest arises to address the food waste problem, which is one of the main causes of food loss due to inadequate storage (Kour et al., 2023). Efforts in this area aim to mitigate the negative impact of waste in economic and environmental terms and meet the growing consumer demand for fresh fruits (Oladzadabbasabadi et al., 2023). Preserving the intrinsic quality of fruits and maintaining their organoleptic, nutritional, and sensory properties throughout their life cycle is a central objective (Muhammad et al., 2023).
The main objective of this study was to investigate the physicochemical characteristics of sweet limes stored at RT (21°C) and at low temperatures (2°C and 4°C). These temperatures were chosen because storage at reduced temperatures is a technique commonly used in the agri-food industry to extend the shelf life of perishable products (Badiche-El Hilali et al., 2023; Habibi et al., 2023; Morales et al., 2024). However, the effect of low temperatures on citrus bioactive compounds, such as carotenoids and vitamins, needs to be further investigated. In addition, comparative studies between different conservation methods are required to assess their impact on citrus quality, as existing studies in this area still need to be completed.
2. Materials and methods
Sweet lime (Citrus limetta) fruit was collected in the Magdalena district (6° 22’ 22’’ S; 77° 54’ 6’’ W; 1892 mosl), province of Chachapoyas, department of Amazonas. Each sample was approximately 60 grams, with a diameter of 5 cm and a moisture content of 85%. The fruits were transported to the Food and Postharvest Engineering Research Laboratory of the Universidad Nacional Toribio Rodríguez de Mendoza de Amazonas in a polystyrene box containing ice blocks for thermal insulation. Reagents used in the experiments were Folin-Ciocalteu phenol (Merck, Darmstadt, Germany), peptone water, sodium carbonate (Spectrum, New Brunswick, NJ, USA), 2,2′-azino-bis (3-ethylbenzothiazoline-6-sulfonic acid), diammonium salt (ABTS), 2,2-Diphenyl-1-picrylhydrazyl (DPPH), butanol and acetate buffer hydrate (Sigma Aldrich, St. Louis, MO, USA).
The study adopted a completely randomized design for three storage conditions: RT (21°C), 2°C and 4°C. The evaluation was conducted inter-daily for 15 days, before which the harvested material was divided into three lots. All fruits were put on an aluminum foil tray. The following variables of interest were measured: water activity (aw), pH, color index, total soluble solids (TSS), titratable acidity (TTA), and measurements of antioxidant activity (DPPH and ABTS) and total polyphenol content (TPC).
The indirect method of Mathlouthi (2001) was used to determine aw values. For this purpose, a portable aw device (HygroPalm, HP23-AW-A, Bassersdorf, Switzerland) was used. Before filling the device’s tank with pulp, the pulp skin was meticulously removed and cut into small square pieces (approximately 3 cm on each side). Data were then recorded in triplicate after 4 minutes, ensuring the utmost precision in our experiment.
The procedure described by Nur et al. (2023) was used. First, the multi-parameter device (HI2020, Hanna Instruments, Woonsocket, RI, USA) was calibrated using pH reference solutions. For evaluation purposes, approximately 10 g of pulp (without skin) was fused with 90 mL of high-purity water in a beaker. The electrode was then immersed in each sample, and the reading was allowed to reach stability before pH values were taken in triplicates.
The skin color evaluation is an essential indicator of fruit quality and maturity. Therefore, color analysis (CR-400, Chroma Meter, Konica Minolta, Osaka, Japan) was performed on the external equatorial plane of the fruit following the protocol described by Castillo-Chuquizuta et al. (2023). Results were expressed in triplicate using the CIE L*, a*, b* system, where L* indicates the lightness of the fruit color (0-100, from black to white), a* indicates redness (+) or greenish (−) and b* indicates yellowish (+) or blueish (−).
The hue angle (h°) represents the actual color perceived, such as red (0°), orange (45°), and yellow (90°), and was determined by utilizing Equation (1) (Walubengo et al., 2022):
The hue angle was calculated as Chroma (C*). This indicates color purity (Pandiselvam et al., 2023) and was calculated through the following equation:
Color accuracy was determined by the color difference Δ E* (or Δ E* ab), representing the Euclidean distance between two color coordinates in CIELAB space. This is done via the following expression:
The values of a* and b* were used to determine the degree of pitch (h) (Brainard, 2003) according to the following Equation (4):
The color difference ΔE* was used for the calculation of the hue difference Δ H* through the following expression:
The TSS was determined from the juice extracted from the fruit, which was deposited in a portable refractometer (BK-PR90, Biobase, Shandong, China). It was measured in triplicate in Brix degrees (°Brix) as described by Balcázar-Zumaeta et al. (2024).
The TTA was determined according to the AOAC 942.15 method (AOAC, 2010) using a NaOH solution (0.01 N) in the presence of a phenolphthalein indicator. The fruit samples were titrated. Subsequently, the percentage acidity was calculated using the following expression:
Where 0.064 g is the milliequivalent weight of citric acid (Arivalagan and Karunakaran, 2021).
The maturity index was calculated based on the relationship described by (Balcázar-Zumaeta et al., 2024) using the following equation:
Where TSS and TTA are Total soluble solids and Total titratable acidity, respectively.
The protocol of Medina-Mendoza et al. (2021) was followed with slight adaptations. The DPPH solution was dissolved with ethanol to achieve an absorbance of 0.7±0.02 units at 517 nm. 0.1 mL lime extracts were allowed to react with 3.9 mL of this DPPH solution for 30 min under dark conditions. The decrease in absorbance of the resulting solution was examined, where at 517 nm, the absorbance of the reaction mixture was measured. The results were expressed as μmol Trolox equivalent /mg sample (μmol TE/mg), observing a light purple color. Three repetitions of the analyses were carried out using a spectrophotometer (GENESYSTM, Thermo Scientific, Waltham, MA, USA).
The method described by Balcázar-Zumaeta et al. (2023) was used with some modifications. The ABTS radical cation was generated by reacting 5 mL of an aqueous solution of ABTS with 88 μm potassium persulfate to reach a final concentration of 2.45 mM. The mixture was kept in the dark for 16 hours before use and then diluted with ethanol to an absorbance of 0.7±0.02 units at 734 nm using a spectrophotometer. For the assays, 30 μm extracts were reacted with 3 mL of the resulting product of the ABTS radical solution, presenting a blue-green color under dark conditions. After 6 minutes, the absorbance was measured at 734 nm. The analyses were carried out three times in the spectrophotometer (GENESYSTM, Thermo Scientific), and the results were expressed in μmol TE/mg sample.
The Folin-Ciocalteu method was used as described by de Souza et al. (2014) and Singleton et al. (1999) with some modifications. Extracts (1 mL) were taken from the fruits; these were mixed with 2.5 mL of Folin-Ciocalteu reagent (10%) and 2 mL of sodium carbonate solution (4%). Each mixture was shaken and placed in the dark for two hours at RT. The absorbance was measured at 750 nm against a blank using a spectrophotometer (GENESYSTM, Thermo Scientific). TPC was estimated from a gallic acid standard curve (y= 0.0009x+0.0825; R2=0.9991) and results were expressed as mg gallic acid equivalents (GAE)/g fresh weight (mg GAE/g).
The results are expressed as mean±standard deviation (SD). Tests were performed in triplicate (Table S1), and an analysis of variance (ANOVA) was applied to evaluate the impact of the three storage temperatures (RT, 2°C, and 4°C). This was followed by a Tukey’s multiple comparisons test at a 95% confidence level. Statistical analyses and graph generation were performed using the free software RMarkdown (RStudio, version 2022.07.2+576, Boston, MA, USA).
3. Results and discussion
The storage days significantly affected all evaluated physicochemical properties of sweet lime (p<0.05). Water activity indicates water availability for microorganisms and chemical reactions in a fruit (Rivera et al., 2022). A variation of the water activity (aw) was observed after the seventh day at the three storage temperatures (Fig. 1A). The aw decreased in samples stored at 2°C and 4°C compared to those stored at room temperature.
Water activity plays a crucial role in food conservation, influencing the growth of microorganisms and the chemical decomposition rate, ultimately affecting the product’s quality (Visakh et al., 2022). The decrease at 4°C and 2°C can be attributed to the decreasing mobility of water in the lime as the temperature drops, leading to a more solid structure akin to ice (Perez et al., 2010). This structured state significantly slows down chemical and enzymatic reactions, such as the peroxidase enzyme activity that preserves organoleptic characteristics (Castellano et al., 2016), the non-enzymatic browning caused by ascorbic acid oxidation (Dussán-Sarria et al., 2020) and the degradation of carotenoids and chlorophyll pigments (Díaz-Puertas et al., 2021). Significantly, these reactions, among others, limit the growth of microorganisms like bacteria and fungi (Bora et al., 2020; Suri et al., 2022), providing a reassuring level of food safety. Therefore, lower temperatures have been observed to be more effective in preserving the quality of limes during storage (Khatoon & Gupta, 2015; Mishra & Sangma, 2017).
The TSS (Fig. 1C) showed an inverse behavior to aw; there was a decrease in TSS at RT as aw showed some increase due to the variability of fruit maturity, coinciding with the findings of Kaewsuksaeng et al. (2015). On the contrary, at 2°C, TSS increased aw decreased, aligning with the results of Arévalo et al. (2016) in sour lemon. In our study, both parameters remained almost stable at 4°C, and this may be because the greater the amount of water in the fruit, the greater the dilution of the sugar flavor present, thus contributing to the high juiciness characteristic of the fruit (Morales et al., 2024).
TTA reached its maximum value (Fig 1D) on days seven, thirteen, and nine at RT, 4°C, and 2°C, respectively. After reaching these maximum acidity levels, a decreasing trend was observed during the storage period under the three temperatures. This decline is attributed to the fruit’s continuous ripening process since the decomposition of organic acids causes a gradual reduction in TTA (Ma et al., 2021).
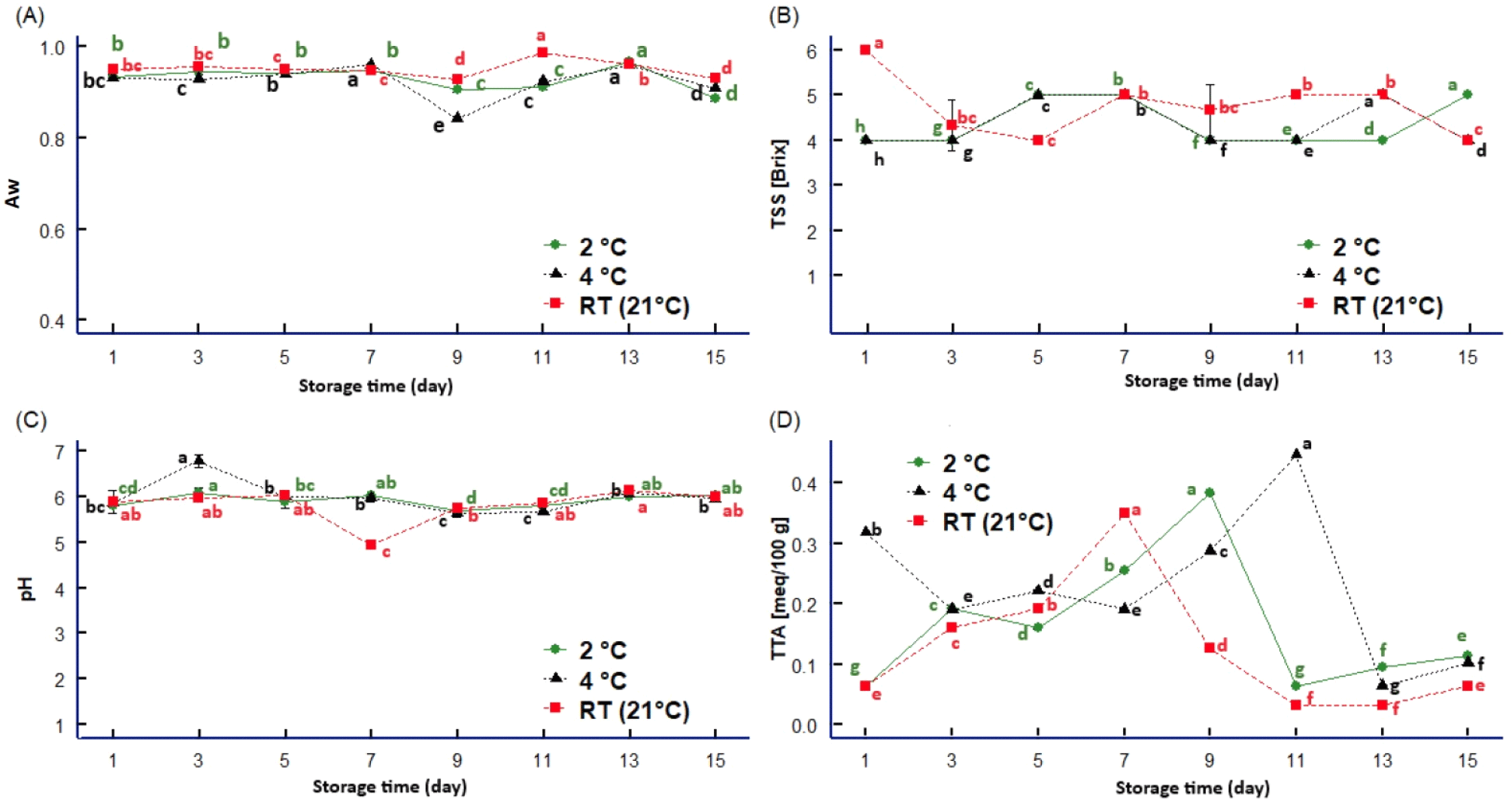
In general, the titratable acidity of a fruit is related to its pH (López-Palestina et al., 2023). In our study, as TTA decreased, there was an increase in its pH during the days of storage at the three temperatures. This is possibly due to a decrease in the content of organic acids such as citric acid, which is responsible for acidity (Garganese et al., 2019). As the days of storage pass, these acids are metabolized or degraded, leading to a decrease in their concentration (Serna-Escolano et al., 2021; Zhang et al., 2021) (Fig. 1B). However, on the third and seventh days of storage, the pH variations observed at 4°C compared to RT and 2°C are possibly due to the limes’ stage of maturity. The pH behavior in our study was similar to that reported by Domínguez and Ordoñez (2018), despite that they used a temperature of 5°C during 20 days of storage. On the contrary, it highlights that pH decreases after 20 days when stored at 5°C and −20°C (Acevedo and Montiel, 2005). The difference between the authors’ findings suggests that other factors impact pH changes over time. This requires further studies to be fully understood. It is essential to consider that individual limes may have natural variations in their composition and biochemical characteristics, which could influence pH measurements.
The enzymatic activity is a crucial factor influencing pH variation. Nayak et al. (2023) note that enzymatic activity is higher at moderate temperatures. On the other hand, this activity decreases at lower temperatures, contributing to a decrease in pH (Costa et al., 2020; Qasim et al., 2021). At lower temperatures, the rate of lime degradation may be slower compared to fruit stored at RT. This results in greater stability of titratable acidity levels, as reported by Bora et al. (2020) and Marchan et al. (2021).
When evaluating the maturity index of sweet lime, a marked decreasing trend was observed in storage at RT and 4°C in contrast to 2°C conditions (Table 1). Sweet limes undergo a natural ripening process reflected by the increased maturity index observed at RT. During this process, fruits stored at RT change their chemical composition and organoleptic characteristics (Tang et al., 2023). The lime will also acquire sweetness and a more pronounced aroma and flavor (Abdellah et al., 2022).
All values are mean±SD (n=3), and different superscript letters (a-) indicate significant differences between days in each storage conditions (Tukey’s multiple range test, p<0.05).
RT, room temperature (21°C).
The maturity index followed the method described by Balcázar-Zumaeta et al. (2024).
Color is fundamental in consumer purchasing decisions as it is associated with quality and authenticity. It can also be correlated with a specific chemical profile, such as pigments (Patsilinakos et al., 2018). During the storage of the lime, color changes were found. Initially, a light green hue, which is characteristic of maturity, was observed. However, during storage at RT, a decrease in its intensity was observed due to rapid deterioration of skin greenness, chlorophyll degradation (Kaewsuksaeng et al., 2015), and moisture content (85.20%) (Suri et al., 2022).
At 2°C, lime showed adequate color retention, followed by 4°C, as low temperatures slow the fruit’s respiration rate, improving their visual quality (Baena et al., 2023). The color index increased on days 5 and 7 when storing at 2°C, compared to 4°C and RT, due to reduced enzymatic activity (Mesejo et al., 2021; Romero and Lafuente, 2022) (Fig. 2). Similarly, color intensity (chroma) was the strongest when lime was stored at RT, 4°C, and then 2°C followed this.
Limes stored at 2°C and 4°C had a fresher appearance than those stored at RT. Despite the presence of wrinkles on the skin, possibly due to water evaporation caused by the low temperatures (Sandalio et al., 2022), the fruit remained relatively healthy. This suggests that low temperatures slowed the deterioration process and created a favorable environment to prevent the growth of microorganisms (Yao et al., 2023).
Color behavior during storage is related to the maturity index. According to our results, limes preserved their appearance after 15 days of storage at 2°C and 4°C; despite showing a certain degree of wrinkling, the fruits remained healthy. In contrast, the fruits showed more pronounced signs of deterioration at RT, such as wrinkles on the fruit surface, a yellowish tone, and greenish molds, probably due to pathogens and decomposition (Volentini et al., 2023; Yao et al., 2023). These results underscore the importance of effective storage conditions, as fruits are highly perishable and susceptible to postharvest pathogens, leading to the exploration of various treatments, such as the use of melatonin (Bose et al., 2021) and oligosaccharides, to extend shelf life and maintain fruit quality (Bose et al., 2021; Ahmed et al., 2020).
Fig. 3 shows findings of the antioxidant activity and polyphenol content. Analysis of antioxidant activity using the ABTS and DPPH methods revealed patterns of interest. Both methods showed decreased antioxidant activity at RT during the 15 days of evaluation. However, the results varied when samples were stored at low temperatures. A slight increase in the antioxidant activity was observed with the ABTS method at 2°C and 4°C. On the other hand, using the DPPH method, samples stored at 2°C showed a slight increase in antioxidant activity, in contrast to that at 4°C. A decrease was observed at RT.
Domínguez and Ordoñez (2018) evaluated the antioxidant activity of citrus juices and sweet lime by the ABTS free radical inhibition. They found that antioxidant activity decreased throughout the storage period for 20 days at 5°C. However, in our study, the antioxidant activity (ABTS and DPPH) of limes stored at 2°C showed an increasing trend as the days of storage passed compared to 4°C and RT. Abid et al. (2014) mention that low temperatures reduce the enzymatic activity and the metabolism of fruits, which can influence the reduction of flavonoids and phenols content. However, in our study, the antioxidant activity of lime at 4°C and 2°C, determined by the ABTS method, remained stable during the 15 days of storage, possibly due to low oxidation and inhibition of enzyme reaction at low temperatures (Cao et al., 2010), indicating that these two temperatures may be optimal for preserving the antioxidant properties of the fruit for 15 days (Revantino et al., 2022).
The TPC decreased significantly during three storage conditions; however, a smaller decrease was observed at 2°C. The analysis revealed a higher concentration on day 1, reaching a value of 3.80±0.145 mg GAE/g sample, as depicted in Fig. 3A. However, following this, the concentration decreased; this decrease could be attributed to the presence of non-polar compounds, as pointed out by Munir et al. (2018) and Wang et al. (2014). In addition, this phenomenon could be related to the type of solvent used for the phenol extraction. Pérez-Nájera et al. (2013) reported that using acetone and hexane as solvents can cause an altered reaction with non-polar compounds, resulting in a lower phenol extraction. On the other hand, it is observed that the phenol content remains more stable on days 7 and 9, as shown in Fig. 3B. This behavior could be attributed to the inherent properties of lime’s citric nature, as indicated by Domínguez and Ordoñez (2018).
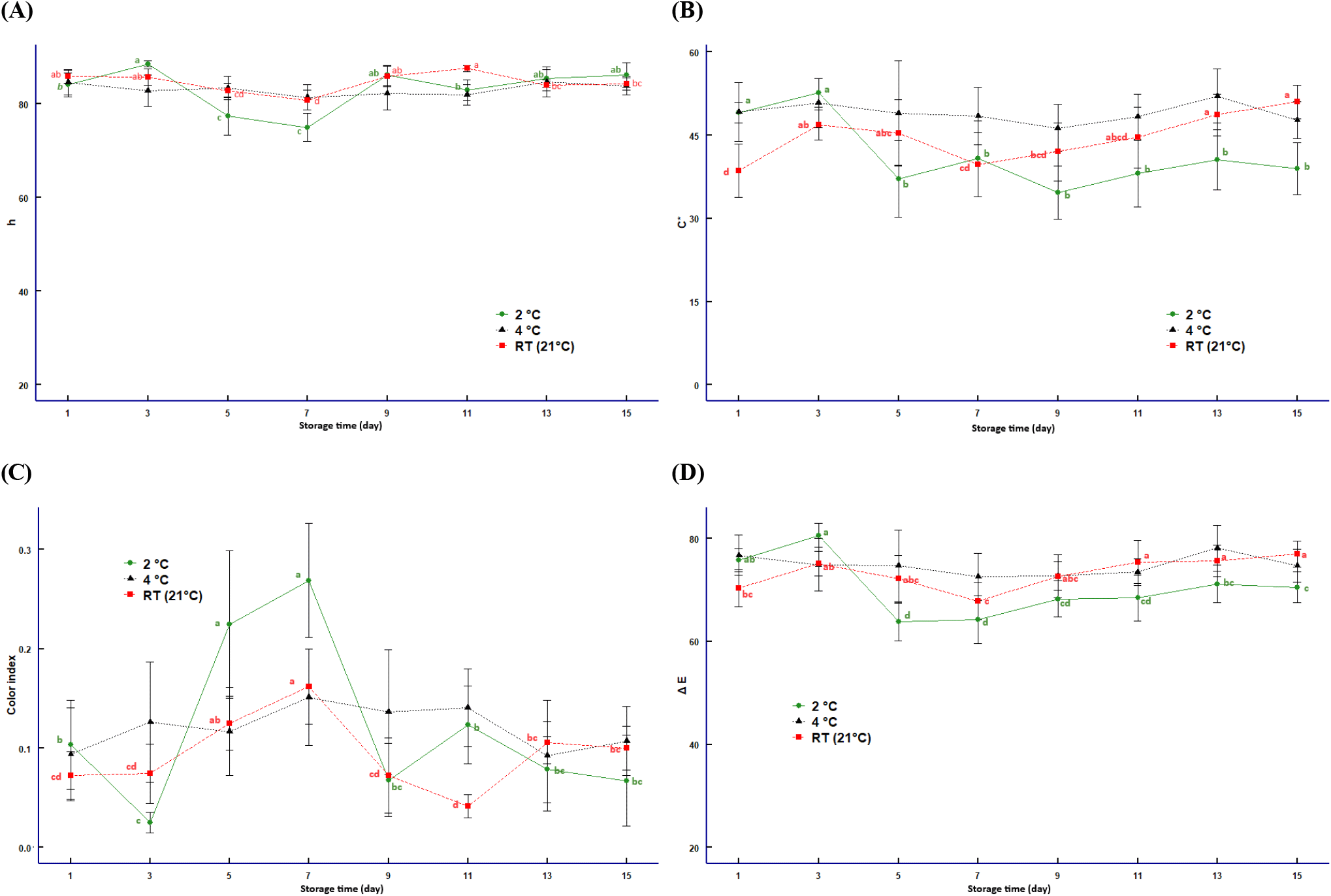
4. Conclusions
This research contributes to identifying the optimal fruit storage temperature (RT, 2°C, and 4°C) by providing a detailed comparative analysis of their effect on the physicochemical properties of sweet lime.
During the study period, a reduction in aw was observed in sweet limes stored at 2°C and 4°C. In addition, at lower temperatures compared to RT, an increase in TSS and a decrease in TTA were observed. Also, limes stored at 2°C and 4°C show a more preserved appearance than those stored at RT. Regarding bioactive compounds, total polyphenols were better preserved at 2°C than at room temperature (RT) and 4°C. However, antioxidant activity was optimally preserved at 4°C, although it was maintained at 2°C to a lesser degree. Thus, while 2°C is better for polyphenols, 4°C is superior for preserving antioxidant activity.
Compared to the other two storage temperatures, 4°C was better able to maintain the physicochemical properties of sweet lime. However, the effect of relative humidity should be considered in future studies. These findings have significant practical implications for the industry and agri-food producers. By highlighting the superiority of low temperatures for sweet lime conservation, this research provides a clear path to reducing food waste and maintaining the fruit’s quality.
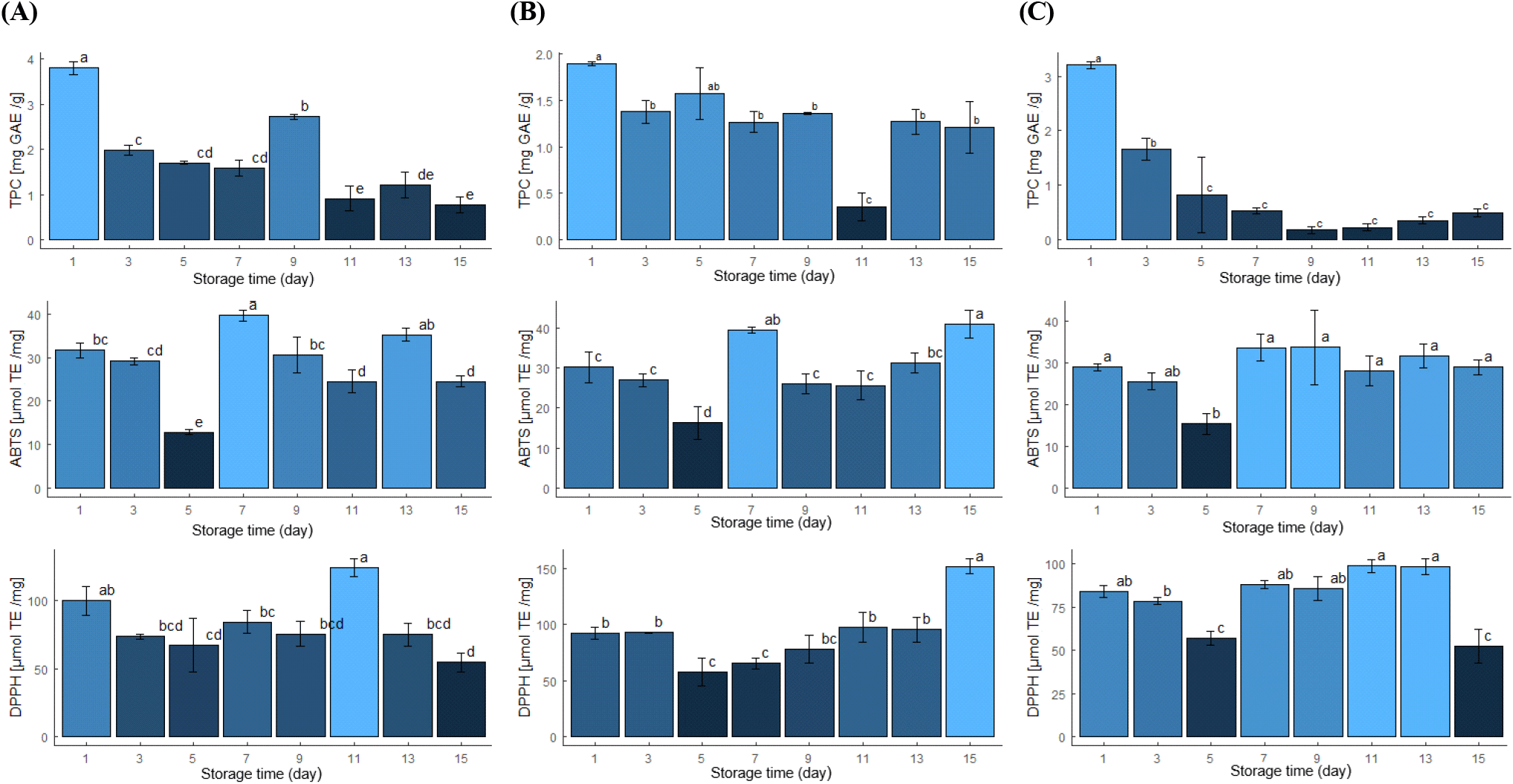