1. Introduction
Ecklonia cava (EC), a valuable brown aquatic plant, is mainly inhabits the subtidal regions of South Korea, Japan, and China (Lin et al., 2021). Specifically, EC proliferates in the coastal areas of Jeju Island, Korea, and is processed for the manufacture of food, additive, and cosmetic products (Choi et al., 2020; Lee et al., 2010b). EC possesses various substances, including polysaccharides, carotenoids, peptides, and phlorotannins (Heo et al., 2009). Among them, dieckol, a type of phlorotannin, has gained considerable attention owing to its biological properties, such as its antioxidant, anti-diabetic, and immunomodulatory activities (Lee et al., 2010a; Park et al., 2008; Park et al., 2010).
Several extraction technologies, including water bath extraction (WB) and ultrasound-assisted extraction (UAE), have been employed to optimize dieckol extraction from EC (Park and Lee, 2021; Shin et al., 2023). In addition, diverse organic solvents, methanol, ethanol, ethyl acetate, butanol, and acetone have been utilized (Rajan et al., 2021). However, using these conventional solvents for extraction causes excessive chemical waste and environmental damage in green chemistry (Oancea et al., 2013). Therefore, the use of a green chemical to extract dieckol from EC is necessary.
Recently, the use of deep eutectic solvents (DES), which are green chemical solvents, as alternatives to conventional solvents has recently been applied to extraction of natural compounds (Dai et al., 2013). Typically, DES are synthesized based on the bonding between hydrogen bond acceptors (HBAs, e.g., choline chloride) and hydrogen bond donors (HBDs, e.g., glucose, glycerol, and lactic acid) (Francisco et al., 2013). The final eutectic solvent possesses a dramatically lower melting point than each individual component owing to the hydrogen bonding between HBAs and HBDs (Wei et al., 2015). Natural deep eutectic solvents (NADES) constitute a DES subgroup comprising sugars, alcohols, and organic acids as its HBDs (Choi et al., 2011). They have demonstrated numerous advantages, such as low toxicity, biodegradability, inexpensiveness, and greater designability with various combinations of HBAs and HBDs (de los Ángeles Fernández et al., 2018). In addition, combining NADES with UAE potentially enhances extraction efficiency (Corbin et al., 2015). However, dieckol extraction from EC via UAE using NADES is currently uncommon.
Therefore, this study is the first to investigate the extraction ability of several NADES types to decide the most optimal NADES for dieckol extraction from EC compared with conventional solvent. In addition, kinetic extraction using different NADES water content levels was also employed to improve extraction efficiency. Moreover, to provide comprehensive information regarding the NADES employed herein, their structural and rheology properties, including their Fourier-transform infrared spectroscopy (FTIR) findings and viscosity were also investigated.
2. Materials and methods
EC, originally produced on Jeju Island in January 2021, was obtained from Parajeju Co. (Jeju Island, Korea). Dried EC was ground using a grinder (RT-04; Mill Powder Tech Co., Ltd., Tainan, Taiwan), sieved through a 40-mesh sieve, and subsequently kept in a ziplock bag at −18°C for further analysis.
To prepare NADES, choline chloride and xylitol were purchased from (Daejung Chemicals, Gyeonggi-do, Korea), while glucose, fructose, ethylene glycol, sorbitol, citric acid, tartaric acid, and acetic acid were procured from Duksan Chemicals (Ansan, Korea). DPPH and ABTS+ reagents, and other chemicals for high-performance liquid chromatography (HPLC) analysis, and the standard were from Sigma-Aldrich (St Louis, Mo, USA).
The dieckol extraction was employed using a previously described method (Park and Lee, 2021), with minor modifications. Briefly, 0.5 g of sample powder was mixed with 50% ethanol (30 mL) and the resulting mixture vortexed. Thereafter, UAE (KHC-1SUMP; Kyung Il Ultrasonic Industries Co., Ltd., Ansan, Korea) was employed at 600 W, 30°C, and a 100-kHz amplitude for 20 min. After extraction, the extract was centrifuged at 4,000 rpm for 10 min and the collected supernatant was subjected to dieckol content analysis.
Different choline chloride-based NADES types, including sugar (glucose and fructose)-, sugar alcohol (ethylene glycol, xylitol, and sorbitol)-, and organic acid (citric acid, tartaric acid, and acetic acid)-NADES, were synthesized at an HBA: HBD molar ratio of 1:1. The eutectic mixtures were heated in a capped flask at 80°C with continuous stirring until the two components had formed a transparent liquid. The obtained NADES were cooled and kept at 25°C in a chemical reagent until further experimentation. Thereafter, EC powder was added to the NADES samples and the resulting mixtures were sonicated using the same method as the abovementioned traditional-UAE method. The supernatant from each NADES extract was diluted with distilled water (10 times) and subsequently subjected to dieckol content analysis.
The dieckol contents of the ethanol and NADES extracts were determined via HPLC as explained previously (Goo et al., 2010), with some modification. The ethanol and NADES extract supernatants were filtered through a 0.2-μm syringe filter (D2520; Echrome Science, Daegu, Korea). The sample was detected using a UV/VIS detector (UV-2075 plus, Jasco International Co., Ltd., Tokyo, Japan) at 254 nm following being injected the filtrate (20 μL) into an HPLC system (Jasco International Co., Ltd.) set up with an Athena C18 reverse phase column (250 mm×4.6 mm, 5 μm). The degassed mobile phases were as follows: 0.1% formic acid in HPLC water (A) and 0.1% formic acid in acetonitrile (B). The following gradient elution conditions were applied: 0-17 min, 15% B isocratic; 17-30 min, linear gradient from 15% to 27% of B; 30-40 min, 27% B isocratic; 40-45 min, linear gradient from 27% to 60% of B; and 45-50 min, linear gradient from 60% to 13% of B, used at a 1-mL/min flow rate. Dieckol (Bota Medi, Seoul, Korea) was used to construct a calibration curve (10-160 μg/mL).
The kinetic extraction of dieckol using different NADES water contents and convention solvent were investigated using a second-order model. Here, model parameters, including the extraction rate constant and extraction capacity, were determined to enable a comprehensive evaluation of the mechanism underlying this extraction method. Second-order kinetic extraction was applied using equation (1) (Harouna-Oumarou et al., 2007):
Where k is the second-order extraction rate constant (mg/g min), Ce is the extraction capacity (dieckol content) at a given time t, and Ct is the extract’s dieckol content (mg/g) at any time. Equation (2) entails integrating the rate law for this second-order model under the boundary condition t = 0 to t and Ct = 0 to Ct, as follows:
or
Equation (3) was replaced in a linear equation form to generate equation (4), as follows:
Plotting t/Ct against t in equation (4) generated a line with the linearized form of y = ax + b, reflecting a = 1/Ce and b = 1/kCe2. Based on these results, the second-order extraction rate constant and extraction capacity were calculated. The correlation coefficient (R2) between the expected model and actual experimental model was also determined.
Following NADES-UAE extraction in section 2.2.2., the initial dieckol extract (16.6 mg/mL) was collected and filtered using a fine-mesh filter (Whatman No. 2; Whatman Int., Ltd., Maidstone, UK). Thereafter, different-concentration dieckol mixtures (0-8.3 mg/mL) were prepared with distilled water and subjected to analysis.
DPPH free radical scavenging activity was investigated using a previously described method (Blois, 1958). Briefly, 100 mL of ethanol (95%) was combined with DPPH (0.0039 powder) in a flask covered with foil, and the mixture was shaken for 2 h using a stirring magnetic bar to produce DPPH solution. Subsequently, 900 μL of DPPH solution (0.1 mM) was mixed with 100 μL of dieckol extract and the resulting mixture reacted in the dark for 30 min. The sample’s absorbance was recorded at 517 nm using a UV-spectrophotometer (UV-2550, Shimadzu, Tokyo, Japan). DPPH activity (%) was calculated as follows:
Where A0 and A1 are the absorbance values for the control and sample, respectively.
ABTS+ free radical scavenging activity was measured using the ABTS+ assay according to a previously described method (Arnao et al., 2001). Briefly, 7 mM ABTS reagent and 2.45 mM potassium sulfate in distilled water were mixed to prepare ABTS+ solution in the dark for 16 h. Subsequently, 50 μL of dieckol extract and 950 μL of ABTS+ solution were reacted in the dark for 30 min. The sample’s absorbance was measured at 734 nm using a UV-spectrophotometer. ABTS+ activity (%) was calculated as follows:
Where A0 and A1 are the absorbance values for the control and sample, respectively.
The NADES samples exhibiting the best extraction efficiencies at the experimental design stage were selected for further study.
For FTIR spectroscopy, the individual components of the NADES, NADES without water content, and NADES with a certain water content were prepared to observe any changes in the spectrum. The samples’ absorbance values were measured within in a wavelength range of 4,000-400 cm−1, with a resolution of 4 cm−1, using an FTIR spectrophotometer (Frontier, PerkinElmer, MA, USA).
To observe changes in viscosity, NADES samples, both with and without water, were prepared (Section 2.2.2). After sufficient cooling, each sample was placed in a WB at 30°C again for 30 min. Thereafter, the sample’s viscosity was measured using a viscometer (DV2T, Brookfield, Middleboro, MA, USA) coupled with spindle RV 5. Measurements were performed using 30 mL of NADES. Viscosity was determined over an RPM range of 10-200, with the RPM increasing every 10 s.
3. Results and discussion
Eight NADES, including sugar-, sugar alcohol-, and organic acid-based NADES as well as a conventional solvent containing 50% (v/v) distilled water, were used in combination with UAE technology to determine the extraction efficiency of dieckol from EC at the screening stage. The screening results are displayed in Fig. 1. Organic acid-based NADES exhibited better dieckol extraction efficiency (25.38-31.47 mg/g) than sugar-based NADES (17.38-17.52 mg/g), sugar alcohol-based NADES (21.80-25.26 mg/g), and ethanol (27.34 mg/g). The results could support the advantage of using NADES for extraction of dieckol than conventional solvent. According to the previous study by shine et al. (2023), they reported ethanol showed the best extraction efficiency of dieckol among other organic solvents such as acetone and ethyl acetate, and the 50% of ethanol concentration also revealed the highest extraction yield of dieckol. Meanwhile, the organic acid-based showed the improved extraction efficiency of dieckol than 50% of ethanol in our study. Given the previous research and the obtained phenomenon from the screening of NADESs, these results suggest that using organic acid-based NADES than organic solvent is a strategy to increase the extraction efficiency of dieckol from EC.
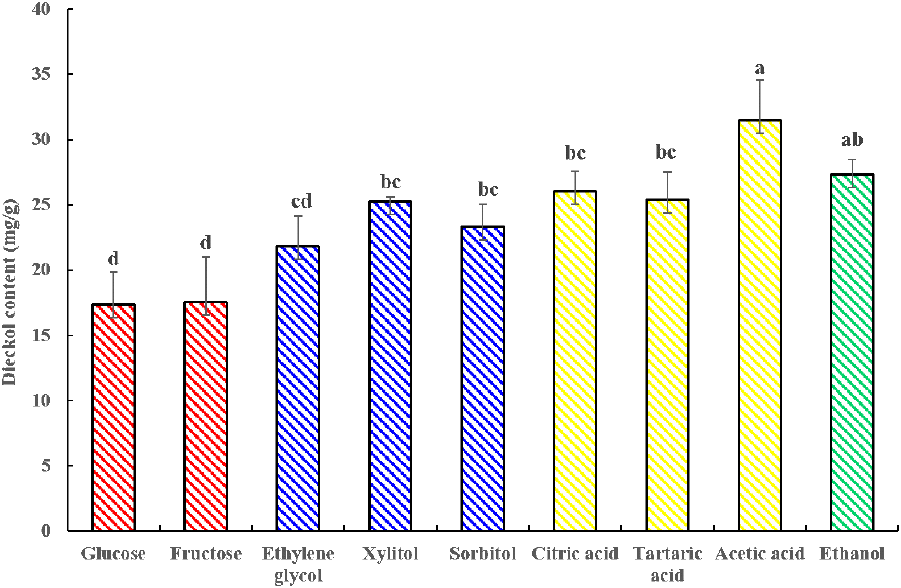
Notably, the extraction efficiency of dieckol varied with HBD type. In general, the ability of DESs to extract natural substances is substantially altered depending on the target compound and DES type (Jiang et al., 2019). In this research, organic acid-based NADES exhibited better extraction efficiency than sugar- and alcohol-based NADES, possibly because the strong polarity correlation between the HBD component and target compound (Jiang et al., 2019). In addition, the higher solvent polarity potentially amplified the extraction efficiency of phenolic compounds (Osorio-Tobón, 2020). Dieckol is recognized as a highly polar phenolic compound (Wijesinghe and Jeon, 2012). According to Dai et al. (2013), organic-based NADES are the most polar, followed by sugar- and alcohol-based NADES. Therefore, the higher polarity of organic-based NADES might have increased the molecular interaction between the solvent and target compound (dieckol), resulting in increased extraction performance. These results are consistent with those previously reported by Jiang et al. (2019), which revealed greater extraction efficiency of polar molecules with organic acid-based NADES than with sugar-based NADES. Moreover, they explained that the different extraction efficiencies potentially resulted from varying DES polarities. Taken together, the increased extraction efficiency associated with organic acid-based NADES presumably enhances intermolecular interaction between the HBD of organic acid-based NADES and dieckol. Consequently, among the organic acid-based NADES, choline chloride-acetic acid (CCAC) with 50% water content (v/v) yielded the highest dieckol content (31.47 mg/g) and was subsequently selected for further analysis.
Modulating the HBA:HBD molar ratio alters the physicochemical properties of an NADES (Lu et al., 2016). Several studies have reported that varying the molar ratio potentially affects extraction efficiency (Cao et al., 2017; Ozturk et al., 2018; Wang et al., 2024). In this study, three different CCAC molar ratios, including 50% (v/v) water content, were prepared for dieckol extraction. The influence of varying the molar ratio on dieckol content is shown in Fig. S1. The results reveal that the dieckol content slightly increased (27.84-30.72 mg/g) with increasing HBD ratio (2:1-1:2) in the NADES. This may be associated with the different NADES properties derived from different HBD ratios. Increasing HBD content potentially improves the extraction efficiency, thereby decreasing NADES viscosity (Cao et al., 2017). Therefore, the decrease of viscosity in NADES facilitates mass transfer from the matrices to the solvent, resulting in increased extraction efficiency (Fuad and Nadzir, 2023). Although no significant differences were noted among all three NADES, the highest dieckol content was observed at a 1:2 molar ratio. Resultantly, a choline chloride-acetic acid molar ratio of 1:2 was selected for the subsequent study.
The water content of NADES has a significant impact on the extraction ability of dieckol, as it varies NADES’s physicochemical properties, including viscosity and polarity (Cho et al., 2024; Xue et al., 2020). We have confirmed that the appropriate water content in NADES between 20% and 80% exhibited the improved the extraction efficiency of bioactive compound in our previous study (Cho et al., 2024). Therefore, in this study, to better demonstrate the effect of different water contents (40-80%) on the extraction method and parameters, such as the extraction rate constant, of the extraction kinetics, a second-order kinetic model was employed compared to 50% of ethanol. The extraction kinetics of dieckol are shown in Fig. 2. The dieckol content dramatically boosted in the initial 1 min, gradually increased and peaked at 15 min, and subsequently decreased until 20 min for CCAC20 (choline chloride-acetic acid with 80% water content [v/v]) and CCAC40 (choline chloride-acetic acid with 60% water content [v/v]). However, the dieckol content of CCAC60 (choline chloride-acetic acid with 40% water content [v/v]) and 50% of ethanol peaked at 20 min. The observed trends can be described by the two-stage extraction process wherein the first stage (0-1 min) entailed the immediate dissolving of dieckol surrounding the aquatic plant’s interface into the NADES, while the second stage featured a gradual diffusion from the material into the NADES (1-15 or 1-20 min) (Fuad and Nadzir, 2023; Milićević et al., 2021).
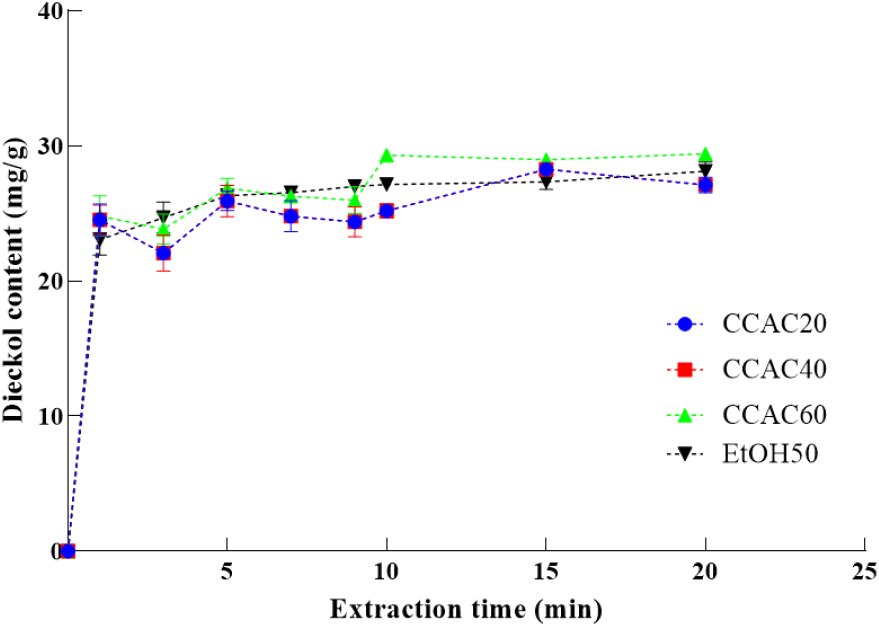
The kinetic model is widely used to evaluate extraction efficiency and yields values for the following parameters: the coefficient of determination (R2), extraction capacity (Ce), and extraction rate constant (k) (Hobbi et al., 2021). The results are shown in Table 1. All the generated models with high R2 values exceeding 0.9 exhibited a good fit to the experimental data. The following Ce values were determined: 28.41, 27.62, 29.15, and 29.67 mg/g at water contents of 80%, 60%, and 40%, respectively. In case of CCAC20, CCAC40, and CCAC60, the k value increased (0.08-0.12 mg/g min) with decreasing water content (80-60%). However, it decreased (0.12-0.07 mg/g min) when water content decreased from 60% to 40%. This suggests that a superior extraction capacity can be achieved with a water content of 40-60%. In addition, the observed increase in k value and decrease in k following with the different water content could be explained as the proper water content could reduce the NADES’s viscosity, which assists in the rapid transfer of dieckol from EC to the solvent, while the higher NADES content may increase the viscosity of the solvent, resulting in the decrease in the diffusion of dieckol (Cho et al., 2024). Moreover, it is worth noting that the CCAC40 showed a higher value of k and Ce than 50% of ethanol in kinetic assay. This obtained results also highlight the strength of using CCAC40 in dieckol extraction than conventional solvent and contribute to an increase in the adaptability of CCAC40 in food industry. Furthermore, an appropriate NADES water content should be optimized to maximize extraction efficiency. Although CCAC40 did not yield the highest Ce, it was selected for the subsequent process owing to it possessing the highest k value, reflecting greater energy efficiency.
Sample | Solvent:water (v/v) | Ce (mg/g) | k (mg/g min) | Coefficient (R2) |
---|---|---|---|---|
EtOH501) | 50:50 | 28.41 | 0.08 | 0.9865 |
CCAC202) | 20:80 | 27.62 | 0.08 | 0.9270 |
CCAC403) | 40:60 | 29.15 | 0.12 | 0.9699 |
CCAC604) | 60:40 | 29.67 | 0.07 | 0.9887 |
Several studies have reported on polyphenol compounds, such as dieckol, which possess various biological properties, such as antioxidant activity (Heo et al., 2010; Kang et al., 2012). The antioxidant scavenging ability of the dieckol extract against free radicals was investigated in comparison with that of CCAC40 only. The results are represented in Fig. 3. The DPPH radical scavenging ability of the dieckol extract ranged from 51.10% to 77.08% (p<0.05) within a concentration range of 1.7-8.3 mg/mL, while its ABTS+ radical scavenging activity increased from 65.94% to 97.20% (p<0.05) with increasing concentration (1.7-8.3 mg/mL). The observed difference between the DPPH and ABTS+ radical scavenging abilities may be attributed to the distinct sensitivities of theses assays to the molecular interactions among the phenolic hydroxyl groups of dieckol and free radical types (Chailangka et al., 2022). This indicates that the dieckol extract exhibited dominant antioxidant activity in a dose-dependent manner without interference of NADES.
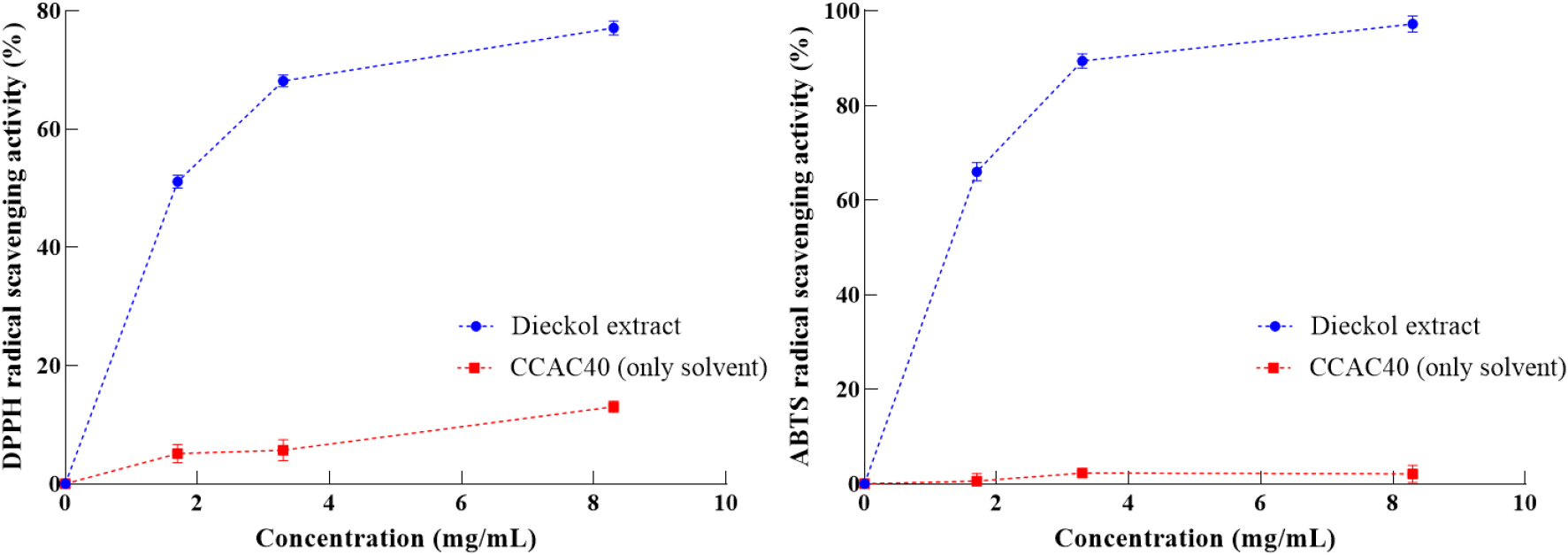
In this study, we confirmed the properties of the tailor-made NADES (CCAC40), which showed the most effective dieckol-extraction efficiency. Moreover, the varied water content in CCAC significantly influenced on the extraction ability of dieckol in kinetic assay. Thus, the effect of the addition of water on physicochemical properties of CCAC40, including FTIR and viscosity, were additionally investigated.
This analysis aimed to confirm the mechanism underlying the synthesized CCAC40 compared with that of the individual components of CCAC and the influence of the water content in CCAC on the structural configuration properties of CCAC40 (Fig. 4). Primarily, the intermolecular interaction among the HBA and HBD was examined. The lowest spectrum (red), reflecting HBA (choline chloride), revealed prominent peaks at 3,217, 3,006, 1,481, 1,083, and 629 cm−1. The peaks of the hydroxyl (-OH) and alkyl groups were observed at 3,217 and 3,006 cm−1, respectively. (Airouyuwa et al., 2023; Karimarji et al., 2024). The peaks observed within the 1,481-1,000-cm−1 range represent the amine group (C-N), C-H bending, and C=O stretching, while other peaks in the 800-500-cm−1 range potentially reflect choline chloride ions (Al-Risheq et al., 2021). The black spectrum indicates the HBD (acetic acid). The hydroxyl (-OH) group of the acid’s stretching vibration is illustrated by the 3,500-2,500-cm−1 range, while the detected peaks within the 1,750-1,690-cm−1 range reflect the C=O functional group of acetic acid (Airouyuwa et al., 2023). The vibrations at 1,450 and 800 cm−1 can be identified as C-O and C-H, respectively (Airouyuwa et al., 2023). Secondly, the synthesized CCAC (blue) differed from the two individual components’ spectra. The broader peaks between 3,500 and 2,500 cm−1 indicate the hydrogen bond, which is characterized by the intermolecular interaction between the nitrogen group of the HBA and hydroxyl group of the HBD (Fanali et al., 2021). CCAC was found to exhibit functional group peaks at 1,470 and 1,714 cm−1 for the HBA and HBD, respectively, and the synthesized CCAC evidently did not alter the peaks of the pure HBA and HBD components. Finally, CCAC40 (green) with 60% water content (v/v) was compared to CCAC. After adding water to CCAC, a considerably broader peak was observed for CCAC40 from 3,500 to 2,500 cm−1 than for CCAC. This peak could be attributed to the asymmetric and symmetric vibrations of the −OH group (Chromá et al., 2021). Moreover, while the peak of the hydroxyl group increased, those of the HBA and HBD components decreased. This finding correlates with that previously made by Chromá et al. (2021), who observed the effect of varied water contents on the structural configuration of the DES and also reported the increased peak intensities with the increased water addition to the DES.
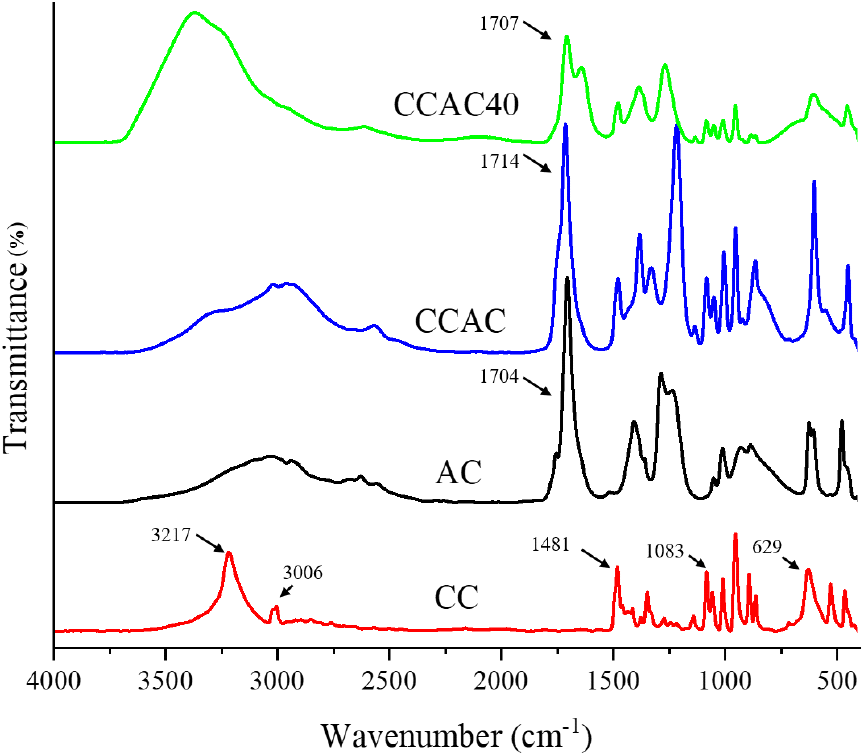
In this research, dieckol extraction using CCAC40 demonstrated the best extraction efficiency, and we confirmed that adjusting water content is a significant parameter for dieckol extraction. Therefore, the effect of water content on the viscosity of CCAC40 was investigated using CCAC. The viscosity data of the two DESs are shown in Fig. 5. The effect of adding water content was apparent in the viscosity assay. CCAC40’s viscosity index decreased from 10 to 200 rpm compared with those of CCAC (Table S1), possibly because the added water weakened the hydrogen bonds of the synthesized CCAC (Panić et al., 2019). Therefore, reducing viscosity may improve dieckol’s solubility and diffusion rate, resulting in increased extraction efficiency (Xue et al., 2020). This phenomenon could be also supported by the analysis of kinetic in section 3.3 where the increased the amount of the water resulted in the increased in the extraction constant rate (k).
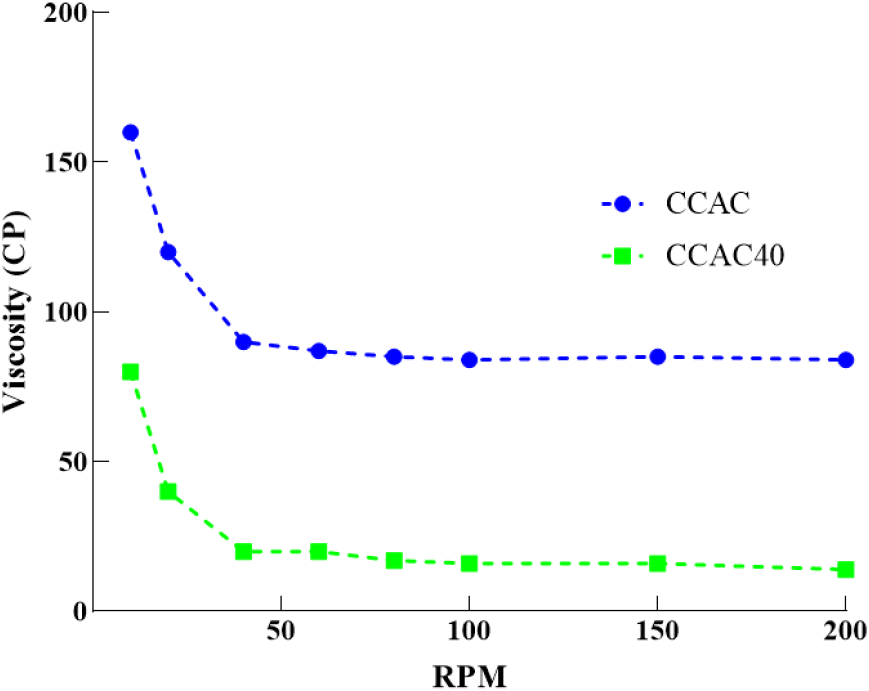
4. Conclusions
In this study, dieckol extraction from EC using emerging organic acid-based NADES combined with UAE was employed and compared with that from sugar and sugar alcohol-based NADES, and conventional solvent. Regarding performance, acetic acid-based NADES (CCAC, at a molar ratio of 1:1) demonstrated superior extraction efficiency (31.47 mg/g) over other NADES and ethanol. According to the second-order kinetic assay, CCAC40 proved to possess a better extraction constant rate and capacity than convention solvent. Furthermore, the dieckol extract exhibited potent DPPH (51.10-77.08%) and ABTS+ (65.94-97.20%) radical scavenging activities. In addition, disparities in structural and rheology properties by the addition of the water content in CCAC were observed via the results of FTIR and viscosity. In summary, this study confirmed that acetic acid-based NADES can be a substitute for conventional organic solvents in dieckol extraction.