1. Introduction
Conventional animal farming has significant environmental impacts, including high greenhouse gas emissions, extensive land use, and substantial water consumption. The production of animal protein requires vast amounts of feed, which often involves deforestation and biodiversity loss (Tallentire et al., 2018). This unsustainable model has prompted a search for alternative protein sources that can mitigate these environmental concerns.
Insects, particularly house crickets (Acheta domestica), have emerged as a promising alternative protein source due to their sustainability benefits. Crickets require significantly less feed to produce the same amount of protein as cattle, making them a more resource-efficient option. Furthermore, insects can be raised on organic waste, contributing to a circular economy and reducing food waste (Pilco-Romero et al., 2023). The nutritional profile of crickets is also favorable, as they are rich in protein, vitamins, and minerals, which are comparable to conventional meats (Payne et al., 2016).
Despite the environmental and nutritional advantages of insect-based proteins, consumer acceptance remains a significant barrier. Many consumers are hesitant to embrace insect products due to cultural perceptions and a lack of familiarity (Grasso et al., 2019). Therefore, it is crucial to develop innovative forms of insect-based products that can appeal to a broader audience. For example, transforming crickets into protein peptides or powders can mask their appearance and texture, making them more palatable for consumers (Hasnan et al., 2023).
Cricket peptides, which are derived from the enzymatic hydrolysis of cricket proteins, represent an innovative approach to utilizing insects in food products. Peptides not only retain the nutritional value of the raw material but also offer improved functional properties, such as enhanced solubility, emulsification, and foaming capacity (Hasnan et al., 2023). Despite these advantages, the development of insect-based products still faces significant challenges, particularly in terms of sensory properties. Protein hydrolysates, including cricket peptides, are often associated with bitterness, which can limit their consumer appeal (Tagliamonte et al., 2024). Masking this bitterness and improving the overall flavor profile of cricket-based products is essential for their commercial success. Herbal extracts and natural sweeteners can be employed to counteract bitterness and enhance the overall flavor experience (Sun-Waterhouse and Wadhwa, 2013).
This study aimed to address two main challenges in developing cricket-based products. First, we compared how two different enzymes—papain and alkaline protease—affect the quality of cricket peptides. Then, appropriate house cricket peptides were combined with natural sweeteners and herbal ingredients to develop an herbal peptide beverage that meets consumer preferences. This approach aims to overcome the sensory hurdles often associated with insect-based foods, potentially increasing their acceptance in the market.
2. Materials and methods
House crickets (Acheta domestica) were obtained from Rawit Farm Interfood in Phayao, Thailand. The crickets were transported and stored frozen until use.
Alkaline protease and papain enzymes were obtained from Sunson Industry Group (Yinchuan, China). The chemicals used in this study included cupric sulfate pentahydrate (J.T. Baker, Phillipsburg, NJ, USA), potassium sodium tartrate (Univar, Australia), bovine serum albumin (Sigma-Aldrich, Ontario, Canada), O-phthalaldehyde (OPA, Sigma-Aldrich, St. Louis, MO, USA), N-acetyl-L-cysteine (NAC, Sigma-Aldrich, USA), boric acid (RCI-Labscan, Bangkok, Thailand), and leucine (Himedia, Maharashtra, India).
Monk fruit (Siraitia grosvenorii) powder, chrysanthemum (Chrysanthemum morifolium) flower powder, lemongrass (Cymbopogon citratus) leaf powder, ginger (Zingiber officinale) rhizome powder, stevia (Stevia rebaudiana) leaf powder, and kaffir lime (Citrus hystrix) peel powder were procured from TPC Herb in Bangkok, Thailand.
100 g of fresh house crickets were homogenized with 200 mL of water. The mixture was then heated in a water bath at 90°C for 15 min, a process demonstrated to effectively inactivate enzymes and reduce microbial activity in edible insects (Cacchiarelli et al., 2022; Mancini et al., 2019). The pH of the cricket homogenate was adjusted to the optimal level for enzymatic activity based on the manufacturer’s specifications: pH 10.0 for alkaline protease and pH 7.0 for papain. Peptides were prepared by adding the protease enzyme (1% w/w of the raw material weight) and incubating at 50°C for 1 hour. The enzymatic reaction was terminated by heating at 90°C for 15 min (Hall et al., 2017). The sample was filtered through muslin cloth, and the filtrate was centrifuged at 4,800 rpm for 15 min. The supernatant was reserved for further analysis.
The protein concentration of the peptide extract was determined using the biuret method. Briefly, 1 mL of supernatant was mixed with 3 mL of biuret reagent. The mixture was allowed to stand at room temperature for 10 min, and absorbance was measured at 540 nm. Bovine serum albumin was used as the standard (Weaver and Daniel, 2003).
The degree of hydrolysis was determined using OPA/NAC reagent. In brief, 1 mL of peptide extract was mixed with OPA/NAC reagent (50 mM OPA and 50 mM NAC in borate buffer, pH 9.5). The reaction was allowed to stand at room temperature for 10 min, and absorbance was measured at 340 nm. Lysine served as the standard (Spellman et al., 2003).
The color of the lyophilized cricket peptide was measured using the CIE L*, a*, and b* color space with a CR-400 Chroma Meter (Konica Minolta, Japan).
Lyophilized cricket peptide (0.75 g) was mixed with 25 mL of distilled water and solubilized by stirring for 10 min. The liquid sample (VL) was homogenized at 17,000 rpm for 2 min and immediately transferred to a graduated cylinder. Initial foam volume (V0) and foam volume after 30 min (VT) were recorded. Foaming properties were calculated using the following equations:
Papain- and alkaline protease-derived peptides were evaluated by semi-trained sensory panels. A rating scale was used to assess cricket odor, bitterness, sweetness, and umami flavors, along with a 9-point hedonic scale for odor and overall acceptance. The peptide with the highest acceptance was selected for formulating the herbal peptide recipe. Sensory evaluation was approved by the University of Phayao Human Ethics Committee (Approval number UP-HEC 1.3/053/64) on 10 January 2022.
Our preliminary study developed three herbal recipes to be mixed with cricket peptide: (1) monk fruit, chrysanthemum, and lemongrass, (2) monk fruit, chrysanthemum, lemongrass, and ginger, and (3) stevia, kaffir lime, lemongrass, and ginger. Aqueous extracts of these herbal combinations were prepared and mixed with house cricket peptide at a ratio of 8:2 v/v, followed by pasteurization. The resulting herbal peptide drinks were evaluated by 32 sensory panelists using a rating scale for cricket odor, bitterness, and sweetness, as well as a 9-point hedonic scale for color, odor, flavor, and overall acceptance.
Data were presented as means and standard deviations. A completely randomized design was employed for assessing chemical and physical properties, while a randomized complete block design was used for sensory evaluation. Analysis of variance (ANOVA) and mean comparisons were conducted using Duncan’s multiple range test in R version 4.2.2 with the ‘laercio’ package (da Silva, 2010; R Core Team, 2022). Principal component analysis (PCA) was performed using the ‘FactoMineR’ and ‘factoextra’ packages (Kassambara and Mundt, 2020; Lê et al., 2008).
3. Results and discussion
The degree of hydrolysis (DH) is a crucial indicator of the extent to which proteins are broken down during enzymatic hydrolysis. The DH of house cricket proteins, which reflects the extent of protein breakdown into peptides, showed no significant difference (p<0.05) between treatments with alkaline protease (6.99%) and papain (6.96%), as shown in Table 1. Peptides with a low degree of hydrolysis (less than 10%) exhibit enhanced functional properties compared to the original protein, such as improved solubility, emulsifying, and foaming properties. These attributes make them suitable for use in foaming foods, such as cakes, bread, and ice cream, as well as in emulsified foods like mayonnaise and sausages (Vioque et al., 2001).
The effectiveness of papain and alkaline protease in enzymatic hydrolysis has been widely debated in the literature, with conflicting results regarding their comparative performance. For instance, papain has been reported to have a higher degree of hydrolysis in the preparation of peptides from Chinese sturgeon (23.56% vs. 18.14%) and gliadin from wheat flour (Li et al., 2016; Noman et al., 2022). Conversely, other studies have shown that alkaline protease can outperform papain in terms of the proteolysis index, such as in pork liver (59.20% vs. 82.56%), casein from goat milk, migratory locust (Locusta migratoria L.) protein flour (9.8% vs. 14.1% at 1% E/S for 60 min), black soldier fly (37.8% vs. 51.4%), and chickpea protein (7.99% vs. 8.62%) (Nadzri et al., 2021; Batish et al., 2020; López-Pedrouso et al., 2020; Purschke et al., 2018; Shu et al., 2015). These discrepancies may be attributed to the distinct mechanisms of action and substrate specificity of each enzyme. Alkaline protease, being a serine protease, may exhibit broader substrate specificity compared to papain, a cysteine protease that requires specific peptide bonds to be effective.
In our study, the peptide yield from alkaline protease (7.43 g/100 g) was significantly higher than that of papain (6.50 g/100 g; p<0.05). This aligns with previous findings where hydrolysate yields from chickpea protein (32.5% vs. 13.92%), hemoglobin (87.92% vs. 78.43%), and meat (81.85% vs. 72.58%) were higher with alkaline protease compared to papain (Nadzri et al., 2021; Li et al., 2020). These results suggest that while papain may be effective in certain applications, alkaline protease generally provides superior yields and hydrolysis efficiency, especially in food protein hydrolysis. The observed variations in effectiveness between these two proteases may also be influenced by factors such as enzyme concentration, temperature, pH, and the nature of the substrate. For instance, the optimal conditions for each enzyme can vary significantly, which may affect their performance in hydrolysis (Kurozawa et al., 2008).
The 1-hour hydrolysis time used in this study aligns with previous research on banded crickets using Alcalase and sandfish roe using Collupulin MG (Hall et al., 2017; Jang et al., 2017). It is shorter than the 2-hour hydrolysis times reported for black cricket protein hydrolysates using Flavourzyme, Alcalase, or Neutrase (de Matos et al., 2024), and for two-spotted and short-tail crickets using SD-NY10 protease (Chaiongkarn et al., 2024). Hydrolysis time is known to influence functional properties, but these properties are also affected by factors such as raw material pretreatment, enzyme type, enzyme-to-substrate ratio, and other process parameters. During the 5-hour hydrolysis of cricket protein fractions using Alcalase, Flavourzyme, and Protamex, hydrolysis rates for globulin remained high throughout with Alcalase, while rates for albumin, glutelin, and prolamin peaked within the first 1-2 hours and then stabilized or slightly increased (Fashakin et al., 2023). In this study, the 1-hour hydrolysis time was selected to balance hydrolysis efficiency and to minimize peptide bitterness, a common concern associated with prolonged hydrolysis (Tagliamonte et al., 2024).
The papain-derived peptide had a significantly higher foam capacity compared to alkaline protease-derived peptide (47.59±8.75% vs. 17.27±2.28%; p<0.05). However, foam stability did not differ significantly between the two (80.44±3.95% for papain and 85.12±2.80% for alkaline protease). The foam capacity of the papain-derived peptide was higher than that reported for black soldier fly protein hydrolysates prepared with both papain and alkaline protease, which exhibited foam capacities of less than 5% (Batish et al., 2020). It also surpassed the foam capacities of migratory locust hydrolysates prepared using various enzymes (Purschke et al., 2018).
Contrasting these findings, hydrolyzed chickpea protein studies reported that hydrolysates prepared with alkaline protease had superior foam capacity and stability compared to those prepared with papain (Nadzri et al., 2021). This discrepancy may be attributed to differences in the degree of hydrolysis and the pH of the solution. Foam capacity can increase during the initial stages of protein hydrolysis, up to a certain degree of hydrolysis (15 to 20% DH), beyond which further hydrolysis results in smaller peptides with reduced foaming abilities (Leni et al., 2020).
The color of the peptide powders produced by both enzymes showed no significant difference in the L* value (lightness). However, alkaline protease-derived peptide exhibited significantly higher a* (redness) and b* (yellowness) values. Chroma and hue angle, calculated from a* and b*, indicated that the papain-derived peptide had a paler yellow color (hue angle of 92.15° and chroma of 4.01). In comparison, the alkaline protease-derived peptide exhibited a more intense yellow color with slight orange hues (hue angle of 86.09° and chroma of 5.87). This suggests that the peptide produced with papain might have a lesser impact on the color of food products when formulated, making it potentially more versatile for different applications.
The papain- and alkaline protease-derived peptides exhibited different flavor profiles. The papain-derived peptide had significantly lower bitterness and higher sweetness and umami taste (Fig. 1). This variation likely results from the different mechanisms each enzyme uses during proteolysis. Peptides and amino acids can have diverse flavor profiles, including sweet, bitter, and umami, and the enzymatic breakdown process can lead to a variety of amino acid profiles from the same raw material (Noman et al., 2022).
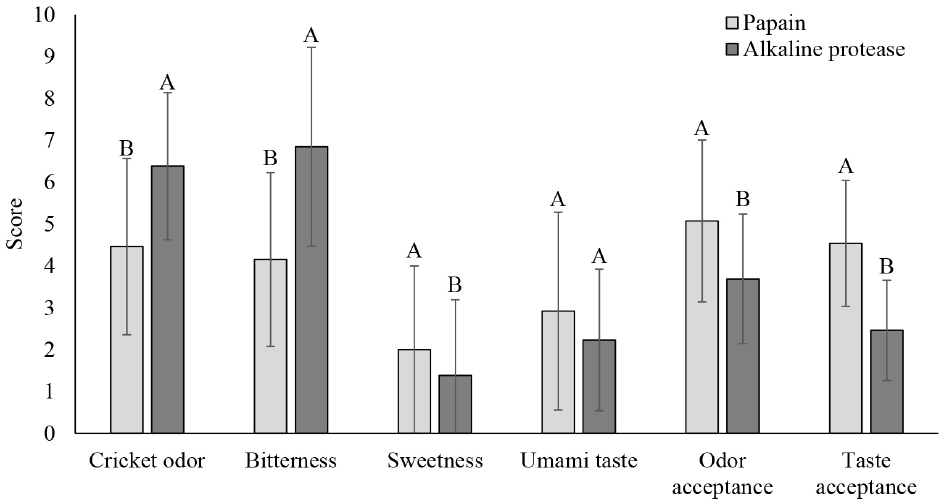
Bitterness is often unavoidable in protein hydrolysate preparation, but the degree of bitterness depends on the amino acid sequence and length. Amino acids exhibit various flavors: glutamic acid provides an umami taste; arginine, proline, leucine, isoleucine, phenylalanine, and tryptophan impart bitterness, while alanine and serine contribute sweetness. Alkaline protease, an endopeptidase, cleaves peptide bonds within the protein, targeting hydrophobic amino acids, particularly when these residues are at the P2’ and P3’ positions. This can result in peptides with greater bitterness, as hydrophobic amino acids are associated with bitter flavors when found internally in peptide chains (Song et al., 2023; Tacias-Pascacio et al., 2020).
The intensity of bitterness in protein hydrolysates is influenced by the position of hydrophobic amino acids. Bitterness is most pronounced when these amino acids are internal within peptide chains. If located at the N- or C- terminus, the bitterness is less intense, and when these amino acids are free, the bitterness is minimal (Matoba and Hata, 1972).
In contrast, papain functions as both an endopeptidase and an exopeptidase, cleaving internal peptide bonds and from the ends of peptide chains, which potentially releases more free amino acids and smaller peptides early in hydrolysis. This activity results in a combination of amino acids that are sweet, umami, and less bitter due to the presence of free hydrophobic amino acids. Additionally, due to its specificity, papain preferentially cleaves peptide bonds involving basic amino acids such as arginine and lysine. This results in peptides where hydrophobic amino acids are less likely to be internal (Storer and Ménard, 2013). Consequently, this can reduce bitterness perception, as these amino acids are more often at the termini or in the free state, where they contribute less to bitterness.
Due to its favorable flavor profile, the cricket peptide prepared with papain was chosen for formulating the herbal peptide drink. As shown in Fig. 2, recipe 1, which includes monk fruit, chrysanthemum, and lemongrass, received the highest overall acceptance scores. This indicates that this particular combination of herbal extracts effectively masked any undesirable flavors or odors from the cricket peptide, resulting in a more palatable beverage.
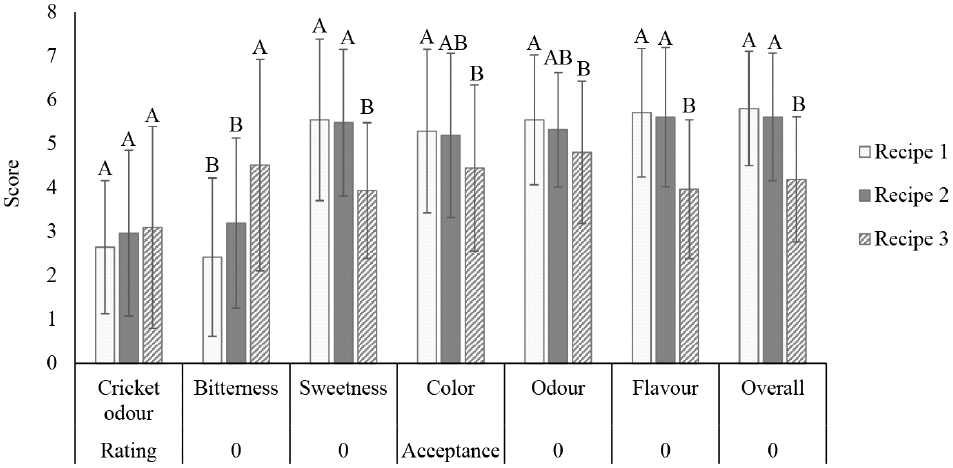
Interestingly, all three formulas received similar ratings for cricket odor, suggesting that the herbal extracts had little impact on the perception of this attribute. However, notable differences in bitterness and sweetness ratings were observed, with recipe 1 being perceived as the least bitter and having the highest sweetness. This aligns with the overall acceptance scores, as reduced bitterness and enhanced sweetness often contribute to greater palatability. Recipes 1 and 2, which both contained monk fruit, did not show a statistically significant difference in sweetness and bitterness scores.
Principal component analysis (PCA) of the seven sensory variables captured 60.67% of the total variance with the first two principal components (Fig. 3), with cumulative variance reaching 91.19% when using five PCs. The eigenvectors indicated that all consumer acceptance variables and sweetness levels varied in the same direction, while bitterness varied in the opposite direction. These findings suggest that sweetness and strong aromatic compounds synergistically mask bitterness, with sweetness being the primary factor contributing to bitterness suppression in recipes 1 and 2.
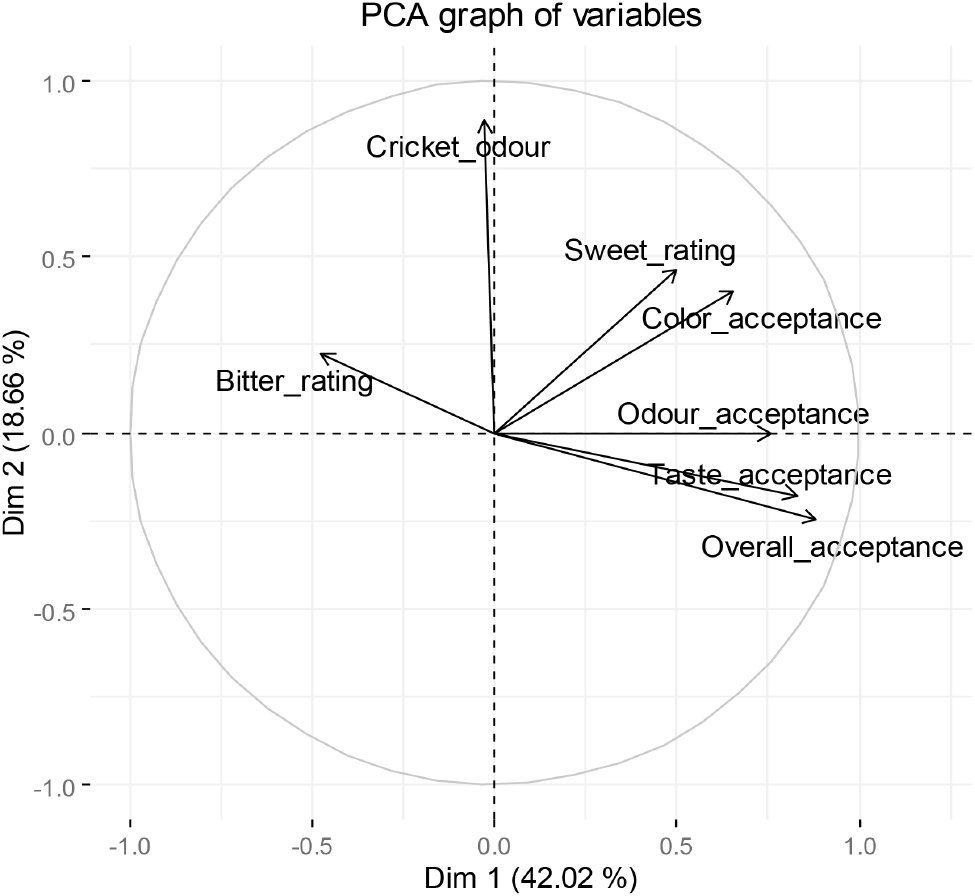
Mogrosides, particularly mogroside V, are the primary sweetening compounds in monk fruit. These triterpene glycosides are significantly sweeter than sucrose, estimated at 250 to 350 times its sweetness. Monk fruit sweeteners have a clean sweetness profile without the bitter aftertaste often associated with natural sweeteners like stevia (Khan and Aroulmoji, 2018). In the development of sugar-free protein beverages, consumers preferred formulations with higher monk fruit ratios over those with more stevia (Parker et al., 2018).
The masking effect of sweetness on bitterness is well-documented. For instance, sucrose effectively suppresses bitter compounds in Brassica vegetables, showing a negative interaction between sweet and bitter tastes (Beck et al., 2014). Sweetness not only masks bitterness but also tempers sourness and saltiness (Green et al., 2010). Studies have shown that sweeteners like fructose and glucose can reduce bitterness induced by high-potency sweeteners such as acesulfame K and saccharin, with bitter receptors (e.g., TAS2R31) suppressed by natural sweeteners (Choi et al., 2024). Neotame, another sweetener, binds more strongly to bitter receptors like TAS2R10, TAS2R14, and TAS2R46, reducing bitter perception (Ke et al., 2022).
The lower consumer acceptance of recipe 3 might be due to the bitterness associated with stevia. Stevia contains different derivatives of steviol glycosides. Among these derivatives, stevioside is a major abundant derivative with the highest bitterness perception, while rebaudioside M has the highest sweetness with the least bitterness (Tian et al., 2022). This suggests that using rebaudioside M instead of crude stevia extract may improve consumer perception.
Strong flavors can also mask bitterness by dominating the overall flavor profile. Chrysanthemum is rich in bioactive compounds that contribute to its medicinal properties and health benefits. These compounds include flavonoids, phenolic acids, anthocyanins, and carotenoids, as well as myriad volatile compounds (Ryu et al., 2019). Lemongrass is a tropical plant renowned for its aromatic properties and a rich profile of bioactive compounds, particularly in its essential oil. The primary bioactive compound in lemongrass is citral, a mix of geranial and neral, responsible for its lemon scent (Hartatie et al., 2020). Ginger is a widely used spice and medicinal herb known for its diverse bioactive compounds that contribute to its health benefits. The primary bioactive constituents of ginger include flavonoids, phenolic compounds (gingerdiol, gingerol, gingerdione, and shogaols), sesquiterpenes, paradols, and various volatile oils (Shahrajabian et al., 2019). Some phenolic compounds with low taste were reported to be bitterness-making agents, including hydroxycinnamic acid derivatives, ferulic acid, and chlorogenic acid (Sun-Waterhouse and Wadhwa, 2013).
In this study, the combination of sweeteners and flavor compounds in recipe 1 likely suppressed bitter taste receptor activity. The sweetness from monk fruit potentially reduced the activation of bitter receptors triggered by any bitterness in the cricket peptide. At the same time, a strong aromatic profile dominated the flavor, contributing to the lower bitterness perception.
A comparative study of novel dietary protein sources, including cricket, pea, and whey proteins, showed that amino acid bioavailability was highest for whey protein, followed by pea and cricket protein (Lanng et al., 2023). Processing cricket protein into peptides can enhance its digestibility and functional properties. In probiotic beverage formulations, the incorporation of cricket protein hydrolysate improved the protein efficiency ratio (PER) and net protein ratio (NPR) compared to intact cricket protein, with apparent digestibility approaching that of casein (Dridi et al., 2023).
Previous studies have successfully developed cricket-enriched beverages, such as a blueberry-fermented drink enriched with cricket peptides and a plantain beverage fortified with cricket flour (Dridi et al., 2024; Sotelo-Díaz et al., 2022). The current study offers an alternative approach by formulating cricket peptides into an herbal beverage. The herbal peptide drink contained 0.8 g of protein per 100 mL, equating to 1.6% of the Codex Nutrient Reference Value (NRV) for protein (Lewis, 2019). Although this protein level does not qualify the drink as a significant dietary source, its formulation targets functional benefits derived from bioactive peptides and herbal components (Zhao et al., 2024).
Cricket peptides hold substantial promise for antihypertensive effects through mechanisms such as enhancing nitric oxide (NO) bioavailability, reducing oxidative stress, and inhibiting angiotensin-converting enzyme (ACE) activity (Hall et al., 2020; Sangartit et al., 2024). Additionally, their collagenase-inhibitory activity suggests potential anti-aging benefits for skin health (Yeerong et al., 2024). Cricket peptides have also demonstrated antioxidant and antidiabetic properties, further supporting their role in health promotion (Chotphruethipong et al., 2024; de Matos et al., 2024; Fashakin et al., 2023).
The inclusion of herbs like monk fruit, chrysanthemum, and lemongrass is expected to contribute bioactive compounds, including polyphenols and flavonoids, which may offer additional health benefits (Chen et al., 2021; Hu et al., 2024; Tazi et al., 2024). Further research is essential to fully harness the potential of this beverage in promoting cardiovascular health and supporting targeted functional health benefits.
Cricket peptides present significant potential for scalability and commercialization in the food industry, aligning with the increasing consumer demand for sustainable and functional foods. Crickets are an eco-friendly protein source, characterized by high feed efficiency and a low environmental footprint, making them a viable alternative to conventional proteins (Rivero-Pino et al., 2024). Functional beverages incorporating cricket peptides can capitalize on the growing market for health-oriented products, offering bioactive benefits such as antihypertensive, antioxidant, and anti-aging properties (Hasnan et al., 2023). Overcoming consumer hesitations will require strategic marketing efforts that emphasize both health benefits and sustainability to enhance acceptance.
The enzymatic hydrolysis process used to produce cricket peptides is adaptable to industrial-scale operations, leveraging established protein hydrolysis technologies to facilitate efficient scale-up (Iñarra et al., 2023). Regulatory compliance and the establishment of a reliable supply chain are critical for successful commercialization. Countries like the EU have already approved insect proteins for human consumption, signaling broader acceptance and market potential (Traynor et al., 2024). Future research should focus on pilot-scale production, sensory evaluations, and consumer trials to validate the commercial feasibility of cricket peptides and their acceptance in the functional food sector. This approach will support the broader adoption of these innovative products, contributing to a more sustainable and health-focused food industry.
4. Conclusions
This study explored the preparation of cricket peptides and the formulation of an herbal peptide drink using different enzymatic methods and herbal combinations. Papain-derived peptide exhibited a more favorable sensory profile, characterized by lower bitterness and enhanced sweetness and umami flavors compared to alkaline protease-derived peptide. Although alkaline protease yielded higher peptide concentrations, papain-derived peptides offered superior palatability, making them more suitable for beverage formulations.
Among the tested herbal recipes, the combination of monk fruit, chrysanthemum, and lemongrass (recipe 1) received the highest consumer acceptance scores. This recipe effectively masked undesirable flavors associated with the cricket peptide, largely due to the sweetness and complex aroma profile provided by the herbal ingredients. The use of monk fruit as a sweetener was instrumental in achieving a balanced flavor profile, suppressing bitterness, and enhancing overall sweetness. PCA confirmed that sweetness positively correlated with consumer acceptance, while bitterness had an inverse relationship. The findings suggest that the strategic use of sweeteners like monk fruit and aromatic herbs can significantly improve the palatability of protein-based beverages.