1. Introduction
Inulin fructans, the most extensively studied group of fructans, are linear polydisperse fructose polymers consisting mainly of β-(2-1) fructosyl-fructose linkages (Roberfroid, 2007; Silva et al., 2019). They are prebiotics that can be selectively utilized by gut microorganisms, thereby conferring health benefits to the host (Gibson et al., 2017). Though fructooligosaccharides and inulin both are inulin fructans, they have different degrees of polymerization (DP); between 3 and 10 and between 10 and 65, respectively (Kolida and Gibson, 2007). The lack of enzymes in humans to digest inulin fructans leads to the fermentation of these compounds in the colon by bacteria that have β-fructofuranosidase enzymes (Roberfroid et al., 2010), resulting in prebiotic properties.
Inulin fructans serve as the storage polymer in more than 35,000 plant species that belong to monocotyledon and dicotyledon clusters. More importantly, most inulin-bearing plants are utilized by humans as vegetables, such as garlic (Allium sativum), onion (Allium cepa), leek (Allium ampeloprasum), asparagus (Asparagus falcatus), globe artichoke (Cynara scolymus) and Jerusalem artichoke (Helianthas tuberosus) (Illippangama et al., 2022; Man et al., 2021; Mudannayake et al., 2015b; Singh et al., 2019). Due to the availability of inulin in these plants only in small amounts, extracting this vital compound from plant sources to be used as a prebiotic ingredient, sucrose replacer, fat replacer, fiber enhancer, and texture modifier in numerous food systems has gained the research interest of many researchers during the last few decades (Ahmed and Rashid, 2017; Gibson et al., 2010; Yousefi et al., 2018).
Inulin offers a series of biological functions on an individual by enhancing the bifidogenic effect and Saccharolytic fermentation in the colon (Mudannayake et al., 2022). These specific biological functions include immunomodulation, protection of the structural integrity of the gut mucosal barrier, and protection against pathogens, which have immense implications in many GI track-related and non-related disorders including irritable bowel syndrome (IBS), inflammatory bowel disease (IBD), colorectal cancers, obesity, diabetes, and cardiovascular diseases (Illippangama et al., 2022).
Due to the growing importance of inulin in the food industry, it is crucial to have appropriate methods of quantification of inulin in plant parts and food samples. However, the structural complexity and varying degrees of polymerization made the quantification of inulin fructans, a technically complicated, tedious, and expensive task for scientists. In this regard, several direct and indirect approaches have been developed to determine the inulin content in plants as well as in foods, including the enzymatic spectrophotometric method (Mudannayake et al., 2015a; Steegmans et al., 2004), high-performance liquid chromatography with refractive index detection (HPLC-RID; Mudannayake et al., 2015a), high-performance liquid chromatography with evaporative light scattering detection (HPLC-ELSD; Li et al., 2018; Perović et al., 2022), high-performance anion exchange chromatography with pulsed amperometric detection (HPAEC-PAD; Bedzo et al., 2021; Tran et al., 2022), high-performance thin-layer chromatography (HPTLC; Simonovska, 2000; Singh et al., 2021), and other colorimetric methods. However, each method has its typical advantages and disadvantages.
The current review evaluates the fermentability of inulin fructans in colon, health benefits, and current state of knowledge on different quantification methods of inulin fructans.
2. Inulin fructans
Inulin is a naturally occurring linear fructose polymer with β-(2-1) fructosyl-fructose linkages that constitute a majority of linkages, usually with an ending glucose molecule. Even though most inulin fructans are considered linear molecules, some native inulin has a very small degree (1-2%) of branching (Ahmed and Rashid, 2017; Hussain et al., 2019). Inulin-type fructans greatly differ in their molecular structure and molecular weight due to varying numbers (3-60) of fructose monomers (El-Kholy et al., 2020). Thus, inulins are mixtures of oligomers and polymers that are characterized by the average DP (Roberfroid, 2004). The DP of inulin is an important property since it significantly influences the functionality of the food systems and bifidogenic properties in the colon (Ito et al., 2011; Singh et al., 2018).
Inulin fructans are naturally found in many food plants such as shallot, leek, garlic, asparagus, globe artichoke, chicory, and Jerusalem artichoke (Illippangama et al., 2022; Mudannayake et al., 2015b; Singh et al., 2019). At present, commercial inulin and fructooligosaccharides are commercially produced mainly from chicory roots (Singh et al., 2018). In addition, Jerusalem artichoke (Helianthus tuberosus), dahlia (Dahlia pinnata L.), yacon (Smallanthus sonchifolius), burdock (Arctium lappa), asparagus (Asparagus falcatus), garlic (Allium sativum), blue agave (Agave tequilana), and dandelion (Taraxacum officinale) have been identified as alternative or novel plant sources to commercially extract inulin (Mudannayake et al., 2022).
Inulins in plants are synthesized using sucrose as a substrate. The two key enzymes engaged in the inulin synthesis are; sucrose: sucrose 1-fructosyltransferase (SST) and fructan: fructan 1-fructosyltransferases (FFT; Wang and Cheong, 2023). The former is considered to initiate the inulin synthesis, while the latter is required for the chain elongation process and release of inulin as the main end product (Singh et al., 2018). Fructans have been reported to act as long-term carbohydrate reserves in the storage organs of over-wintering plants. Additionally, fructans are considered to offer cryo-protection and osmotic regulation role in plants, allowing the survival and growth of plants during times of water scarcity induced by either drought or frost (Nyyssölä et al., 2020; Singh et al., 2018). Previous studies conducted on chicory plants have revealed that during times of water shortage in drought or frost season, inulin becomes hydrolyzed, resulting in an increased amount of free fructose and low DP inulin fractions (Nyyssölä et al., 2020).
3. Fermentability of inulin fructans in human colon
The gastrointestinal (GI) tract of a human is inhabited by a highly complex, diverse community of microorganisms, which are composed of more than 100 trillion bacteria (Rinninella et al., 2019). The main functions of gut microbiota include contributing enzymes for human metabolism and fermentation, reducing the colonization of potentially pathogenic microorganisms, and modulation of the immune system (Rowland et al., 2018).
The colon is the most densely colonized and metabolically dynamic region of the human gastrointestinal tract, with up to 1012 bacteria living in each milliliter of gut content (Rinninella et al., 2019). Most microbes in the colon are strict anaerobes, while facultative anaerobes are the second most common type. Commonly encountered genera in the colon include Bacteroides, Eubacteria, Fusobacteria, Bifidobacteria, Peptostreptococci, Clostridia, Lactobacilli, and Streptococci (Roberfroid, 2004).
This large and diverse population of microbiota that resides in the colon is predominantly of two categories: beneficial (potentially health-promoting) and non-beneficial (potentially detrimental) bacteria (Fig. 1).
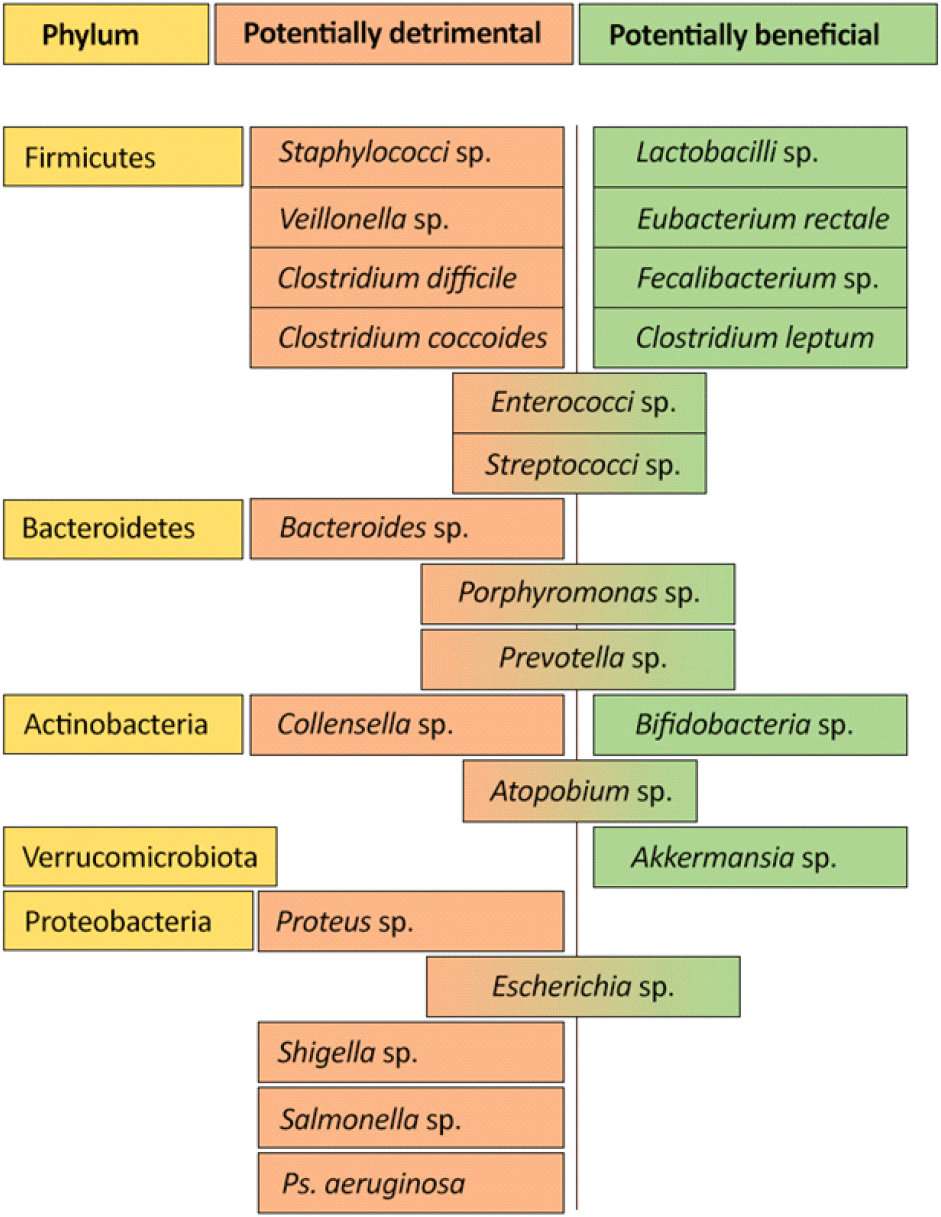
It has been hypothesized that gut microflora must be composed mainly of bacteria recognized as potentially health-promoting (such as Lactobacilli and Bifidobacteria) rather than the potentially pathogenic and detrimental microorganisms (such as Escherichia coli, Clostridia, Veillonellae, and Candida) (Roberfroid, 2004; Yang et al., 2020). However, the potentially harmful category cannot be excluded as some interactions between potentially health-promoting and potentially harmful groups essentially play a role in sustaining health and well-being.
The primary nutrient components that reach the colon for fermentation include non-digestible carbohydrates (such as inulin, oligosaccharides, resistant starch, cellulose, and pectin) as well as residual proteins (Scott et al., 2013). The two main types of fermentation carried out in the colon are saccharolytic fermentation (fermentation of non-digestible carbohydrates) and proteolytic fermentation (fermentation of protein). Saccharolytic fermentation is more beneficial to the host than proteolysis because of the nature of the metabolic end products generated during the process. (Kolida and Gibson, 2007). Saccharolytic fermentation in the colon results in the production of short-chain fatty acids (acetate, propionate, and butyrate), gases (H2, CO2, and CH4), lactate, pyruvate, etc, while fermentation of protein results in SCFAs in lesser amount as well as branched-chain fatty acids (BCFAs), ammonia, N-nitroso compounds, amines, sulfides, and phenols (Scott et al., 2013). Furthermore, some colonic fermentation end products of protein, such as ammonia and N-nitroso compounds act as tumor promoters or carcinogens, thus detrimental to health and well-being (Khalil et al., 2014; Louis et al., 2014). In contrast, fermentation end products of non-digestible carbohydrates, such as SCFAs are considered to have a vital role in the prevention of carcinogenesis and other diseases such as Inflammatory bowel disease (IBD), and thus have a positive effect on the gut and overall health (Fernández et al., 2019; Roberfroid et al., 2010). Recent research on human and animal models indicated that inulin-type fructan intake can significantly reduce the proteolytic fermentation in the colon while increasing the saccharolytic fermentation resulting in an enhanced production of beneficial SCFAs (Illippangama et al., 2022; Seesaha et al., 2020).
The presence of β-(2-1) fructosyl fructose linkage in inulin makes it resistant to hydrolysis by human digestive enzymes, such as α-glucosidase, maltase, and isomaltase. Consequently, inulin proceeds undigested through the stomach and small intestine to the colon (Shoaib et al., 2016).
Many in vivo and in vitro studies have demonstrated that supplementation of inulin and oligofructose substantially alters the composition of gut microbiota by promoting the growth of Bifidobacteria and Lactobacilli (Nagy et al., 2023; Seesaha et al., 2020). A greater Bifidobacteria concentration in the gut has been regarded as a marker of intestinal health (Roberfroid et al., 2010). Several other studies have also reported that prebiotics including inulin fructans and oligofructose can reduce the growth of some potentially harmful bacteria such as Bacteroides, Clostridia, Proteus, E. coli, and Salmonella (Azad et al., 2020; Davani-Davari et al., 2019; Hoffman et al., 2019; Kanjan and Hongpattarakere, 2017; Wang et al., 2020).
The undigested carbohydrates, including inulin fructans, are fermented in the colon by an anaerobic microbial community and produce a range of fermentation products. The major fermentation products in healthy adults are SCFAs, lactic acid, and gases. The SCFAs, which include acetate, propionate, and butyrate, are typically produced in the colon at ratios of 3:1:1, and have a crucial role in maintaining overall gut health (Louis et al., 2014). The production of SCFAs acidifies the luminal pH, thereby promoting the growth of beneficial bacteria such as Lactobacilli and Bifidobacteria (Akram et al., 2019) and suppresses the growth of pathogenic microorganisms (Önal Darilmaz et al., 2019; Vandeputte et al., 2017; Wilson and Whelan, 2017). All these SCFAs are rapidly absorbed by the gut lumen and contribute to different roles in the host. For instance, butyrate is specifically metabolized by gut epithelium cells, where it is used as the major energy source for the epithelium cells (Rowland et al., 2018). It is also found to act as a regulator for cell growth and differentiation. Propionate is metabolized in the liver and is considered to have a role in gluconeogenesis (Scott et al., 2013) and possibly inhibits cholesterol synthesis (Roberfroid et al., 2010). Recent studies have shown that propionate and butyrate reduce colon cancers (Ali et al., 2019; Fernández et al., 2019) and have the potential to reduce IBD symptoms (Illippangama et al., 2022; Louis et al., 2014; Roberfroid et al., 2010). Acetate has been reported to enter the systemic circulation and is used in lipogenesis. Acetate is mainly metabolized in human muscle, kidney, heart, and brain.
Several studies showed that the production of SCFAs is increased due to the consumption of inulin fructans (Fernández et al., 2019; Hutchinson et al., 2023; Thøgersen et al., 2018). Researchers including Kleessen et al. (2001) and Hutchinson et al. (2023) studied the effect of inulin and oligofructose on the gut microbiota of mice. They found that inulin-containing diets significantly increased the butyrate concentration in the cecum and colon. Kleessen et al. (2001) also concluded that the increment of butyrate production might be due to an increased population of butyrate producing bacteria species such as Clostridium coccoides and Eubacterium rectale in inulin supplemented diet subjects. Valcheva et al. (2019) showed that dietary supplementation of inulin-type fructan at a dose of 15 g/d for 9 wk increased colonic butyrate production in ulcerative colitis patients.
4. Health benefits of inulin fructans
Prebiotics are non-digestible ingredients that are selectively fermented, allowing specific changes, both in the composition and activity of the gut microbiota, which provide health benefits to the host (Gibson et al., 2017). Inulin fructans are also recognized as a ‘functional food ingredient’ since they have established beneficial health effects on the body beyond its basic nutrition (Roberfroid, 2007).
The health benefits of inulin fructans via modulating gut health have been widely studied over the last two decades. The better understanding of the link between gut microbiome and overall health by regular consumers has made the food industry use inulin fructans as one of the major functional ingredients of interest in recent years. Inulin fructans exert beneficial biological and physiological effects not only in the colon but throughout the entire body, helping to reduce the risk of numerous miscellaneous intestinal and systemic diseases including IBD, hepatic encephalopathy, diabetes, colon cancer, and cardiovascular diseases and increasing the bone health as depicted in Fig 2. (Du et al., 2023; Mudannayake et al., 2022).
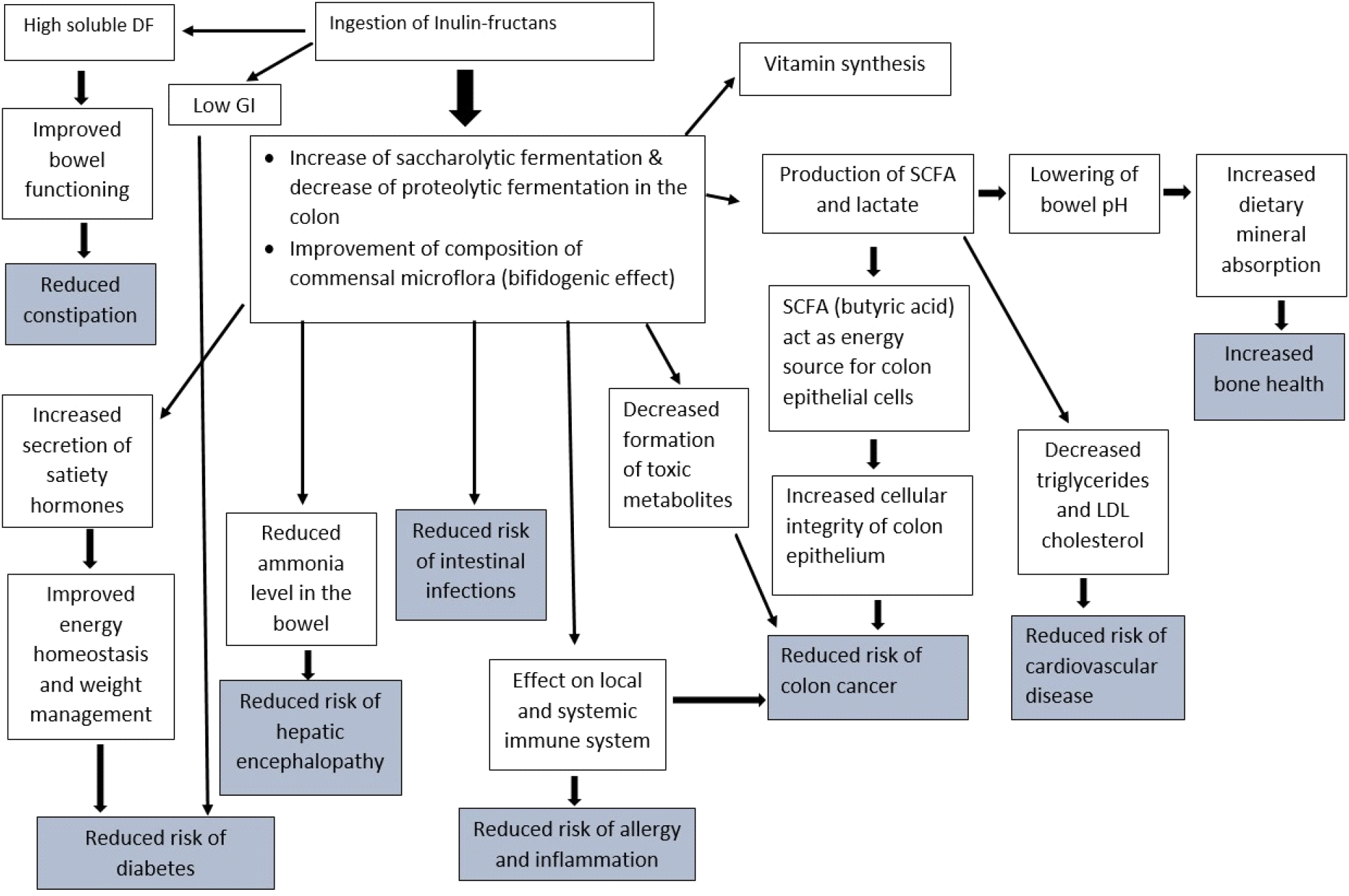
Constipation is one of the most common health impairments that can be observed among elders. It can be caused by a variety of factors, including aging, insufficient fluid consumption, a lack of fiber-rich foods in daily diet, and insufficient physical activity etc. (Illippangama et al., 2022). Researchers have investigated that fermentation of carbohydrates stimulates colonic motility, and thus, administration of inulin to a daily diet could improve constipation, abdominal discomfort, and stool frequency.
Inulin fructan intake has consistently been reported to increase fecal water content, thereby contributing to greater stool weight (Caleffi et al., 2015). A study conducted by Isakov et al. (2013) revealed that regular consumption of inulin-incorporated fermented milk beverages shows significant improvement in the consistency of stools in patients with constipation, while Watson et al. (2019) stated that supplementing chicory inulin at a dose of 10 g per day significantly increased the stool frequency.
The fermentation of inulin in the GI tract produces short-chain fatty acids (SCFAs), such as butyrate, propionate, and acetate, which help stimulate colonic motility and increase water retention in the stool. This softens the stool and facilitates its passage through the GI tract, thereby alleviating constipation. Moreover, the prebiotic effect of inulin supports a balanced gut microbiota, which plays a crucial role in maintaining regular bowel movements and overall gastrointestinal health. As a result, inulin consumption can be an effective dietary intervention for the relief of mild to moderate constipation.
IBD refers to a group of chronic inflammatory conditions formed in the GI tract. The two principal types of IBD are Ulcerative colitis and Crohn’s disease. Ulcerative colitis causes continuous mucosal inflammation that is restricted to the colon and rectum. In contrast, Crohn’s disease could cause discontinuous inflammation anywhere throughout the gut, although most cases are found in the terminal ileum (Roberfroid et al., 2010). The symptoms of Ulcerative colitis and Crohn’s disease include diarrhea, fecal urgency, incontinence, abdominal pain, rectal bleeding, and abscesses. It has been accepted that one of the major causes of IBD involves an imbalance of intestinal microbiota (Ex: overgrowth of Bacteroides and Clostridium genera in the gut over some beneficial species such as Bifidobacteria). Several studies have demonstrated that IBD can be prevented as well as treated using inulin fructans (Akram et al., 2019; Hufnagel et al., 2021; Valcheva et al., 2019).
Many researchers suggest that diet and gut microbiota play a critical role in the development of colon (or colorectal) cancers (Ou et al., 2013). It has been found that colonic microbiota can produce a variety of mutagens, carcinogens, and tumor promoters, including N-nitroso compounds, ammonia, secondary bile acids, phenols from dietary compounds, and endogenously produced precursors (Roberfroid et al., 2010). Additionally, some bacterial enzymes released in the colon, including β-glucuronidase are known to be involved in the formation of different carcinogens, such as polycyclic aromatic hydrocarbons (Roberfroid et al., 2010).
Inulin fructans are believed to possess the ability to modify the gut microflora balance to a healthier composition by increasing the number and metabolic activity of Bifidobacteria and Lactobacilli (Meyer and Stasse-Wolthuis, 2009; Mudannayake et al., 2016; Roberfroid et al., 2010). Bifidobacteria can bind the carcinogens and toxins and physically remove them via feces (Kaur and Gupta, 2002). As previously explained, inulin supplementation in diets has been found to reduce proteolytic fermentation, thus reducing the generation of harmful end products (Seesaha et al., 2020). The production of health-promoting SCFA, such as acetate, propionate, and butyrate, by fermentation of inulin in the colon has been accepted as another mechanism that reduces colorectal cancer risk. All three SCFAs are known to have anti-inflammatory and anti-proliferative properties (Ou et al., 2013). Butyrate, being the principal energy source for colonocytes, helps to maintain the structural integrity of the colonic epithelium cells and is reported to inhibit colon cancers (Louis et al., 2014).
In general, Bifidobacteria and Lactobacilli have low β-glucuronidase enzyme activities, so increasing the numbers of Bifidobacteria and Lactobacilli in the gut could reduce the level of such deleterious enzyme reactions (Burns and Rowland, 2000). Although the exact mechanisms of inulin fructans against colorectal cancer still remain to be elucidated, many studies have illustrated that inulin fructans have the potential to reduce the development and/or progression of colorectal cancers (Fernández et al., 2019; Pool-Zobel, 2005; Pool-Zobel and Sauer, 2007; Thøgersen et al., 2018; Thøgersen et al., 2020).
The incorporation of inulin fructans into the diet has been shown to decrease the risk of elevated triacylglycerol concentrations (Liu et al., 2017; Shoaib et al., 2016). Letexier et al. (2003) demonstrated that adding 10 g/day of high-performance inulin to a carbohydrate-rich, reduced-lipid diet positively impacted plasma lipids in humans by reducing blood lipogenesis and plasma triacylglycerol levels, thereby lowering the risk of atherosclerosis. Conversely, Williams and Jackson (2002) reported varied effects of inulin or oligofructose supplementation on blood lipids in ten human volunteers, with some individuals showing significant reductions in triacylglycerol and moderate decreases in LDL-cholesterol. Studies on hypercholesterolemic patients suggest that inulin can improve blood lipid profiles by modulating lipid metabolism, as evidenced by reduced triglyceridemia and cholesterolemia in experimental animals (Delzenne et al., 2002).
Studies in rats have indicated that inulin and oligofructose lower hepatic lipid synthesis by downregulating genes involved in lipogenesis, a process thought to occur similarly in humans despite ongoing debates about the exact mechanisms (Roberfroid, 2007). Overall, both inulin and oligofructose have been shown to decrease plasma cholesterol and triacylglycerol levels, counteract hepatic triacylglycerol accumulation, and exert favorable effects on hepatic steatosis, with reduced liver lipogenesis being a key mechanism (Beylot, 2005).
There is an increasing number of research evidence that inulin enhances mineral (Ca, Mg, and Fe) bioavailability and thus significantly increases the absorption of these minerals in the colon (Costa et al., 2020; Gibson et al., 2010; Krupa-Kozak et al., 2017). The remarkably increased absorption of minerals in the presence of inulin has been explained by two mechanisms; (1) the osmotic effect of inulin, which transfers more water into the large intestine which makes minerals more soluble in the colon, (2) inulin reduces the colon pH due to SCFA production, thus it forms Ca and Mg salts of these acids, which favors passive diffusion and causes hypertrophy (enlargement of cells) of the colon wall, and improved absorption (Kalyani Nair et al., 2010; Kaur and Gupta, 2002). Calcium is a critical mineral required for bone mass development, and most often inadequate quantities are delivered via the dietary intakes. Thus, the effect of prebiotics on Ca absorption has been extensively studied and proven by many researchers using animal models as well as human clinical trials (Abrams et al., 2005; Abrams et al., 2007; Krupa-Kozak et al., 2016).
5. Commercial production of inulin fructans
The global inulin market is projected to grow from $1 billion in 2021 to $2.2 billion by 2031, with a compound annual growth rate of approximately 6.3%. This can be attributed to the growing global demand for inulin as it is being widely used in food and beverages, nutritional supplements, pharmaceuticals, and animal feed (Transparency Market Research, 2021). There have been three plants, namely, chicory, Jerusalem artichoke, and dahlia, which are identified to contain ≥15% inulin (based on fresh weight) with their potential use for commercial inulin production. These three plants are known to thrive only in temperate regions of the world (Singh et al., 2018; Zhu et al., 2016).
At present, chicory is the main plant extensively cultivated for the commercial production of inulin powder in Belgium, The Netherlands, France, and Chile (Singh et al., 2018). Industrial inulin production generally involves three basic steps; namely (1) extraction of inulin by hot water diffusion, (2) purification of the raw extract, and (3) drying of the purified extract to produce inulin powder (Shoaib et al., 2016). The industrial inulin manufacturing process has been summarized in Fig. 3. Commercial inulin available in the market, however, primarily differs in origin, physical form, average DP, sweetness, and functionality (Illippangama et al., 2022).
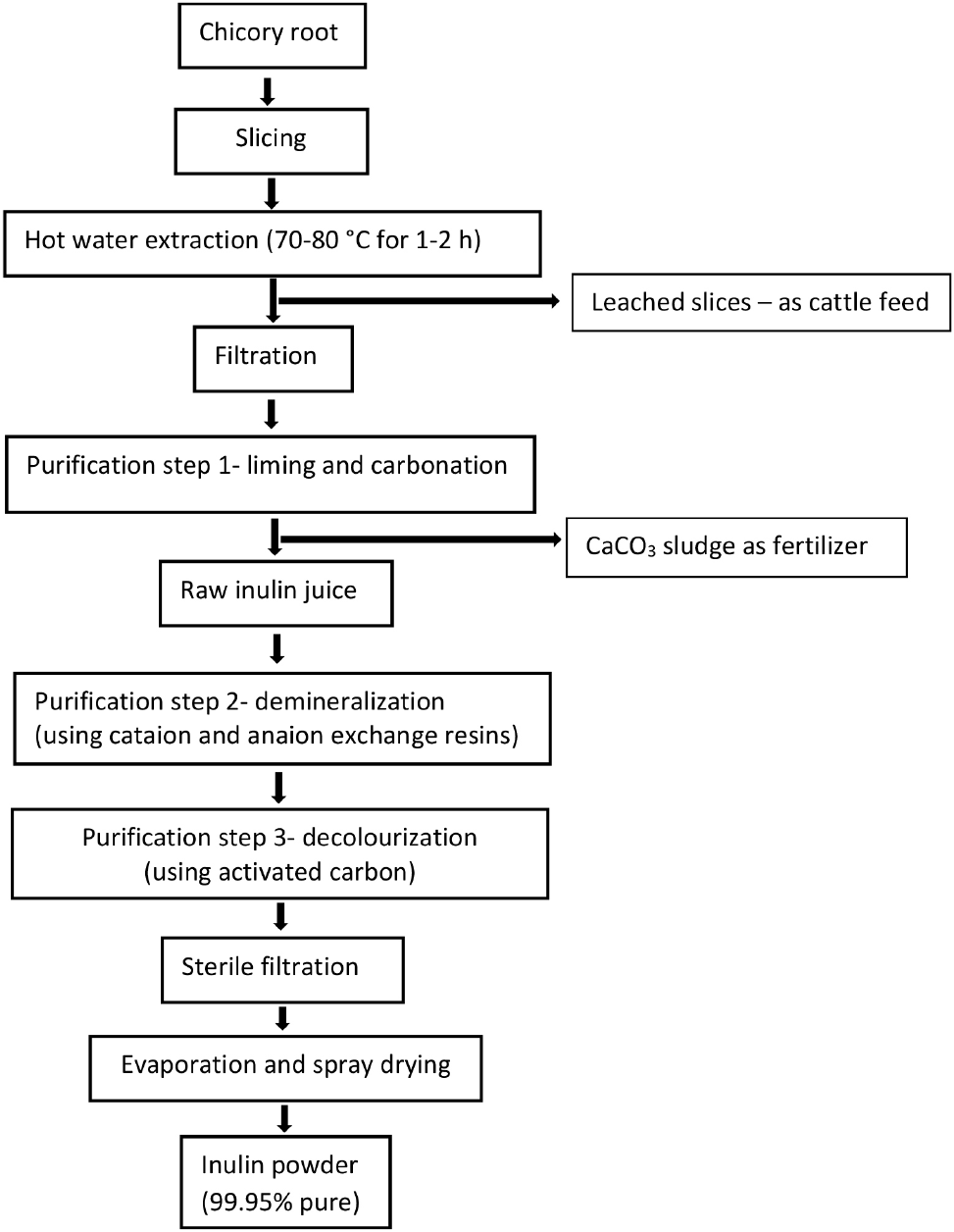
6. Extraction, analysis, and quantification of inulin fructans
Due to the structural complexity, and varying degree of polymerizations, analysis of inulin fructans has become a technically complicated and tedious task for scientists. However, inulin can be quantified using either direct or indirect approaches.
Indirect determination methods are based on acid or enzymatic hydrolysis of inulin polymers into fructose (and glucose), followed by measurement of the released fructose and glucose using various techniques including HPAEC-PAD, high-performance liquid chromatography (HPLC), gas chromatography (GC) or spectrophotometry (Apolinário et al., 2014; Benkeblia, 2013; McCleary et al., 2019; Wang and Cheong, 2023). Indirect methods are accepted as the best method for inulin determination. However, free glucose, fructose and sucrose present in samples should be either removed or measured and subtracted in these indirect methods to avoid over-estimation of fructans. Due to these reasons, quantification of inulin involves multiple or sequential extractions, acid or enzymatic polymer hydrolysis and multiple chromatographic procedures (Benkeblia, 2013). Various methods have been developed for the quantification of inulin. However, all these methods have their typical advantages and disadvantages. Therefore, researchers often use more than one method for inulin determination. The following section briefly describes the currently available common fructan analysis methods. The Association of Official Analytical Chemists (AOAC) has recently adopted two different fructan analysis methods (AOAC 997.08 and AOAC 999.03), which specifically allow the accurate quantitative determination of inulin and oligofructose in foods (AOAC, 2016).
The extraction of inulin from plant samples is a critical step for accurate quantification of inulin content. Inulin is a soluble dietary fiber that is most commonly extracted using hot water due to its high solubility water medium. The conventional hot water extraction method involves immersing sliced or macerated plant materials in water at approximately 80°C for 40-60 minutes with continuous shaking to enhance the extraction efficiency (Esmaeili et al., 2021). Ethanol has also been employed as an extraction solvent, yielding satisfactory recoveries in several studies (Ku et al., 2003; Wei et al., 2007). However, water remains the most efficient solvent for inulin extraction (Esmaeili et al., 2021).
Recent advancements in extraction techniques have introduced several innovative methods that improve efficiency and reduce extraction time. Microwave-assisted extraction (MAE) has been shown to significantly enhance the yield of inulin by utilizing microwave energy to heat the solvent and plant matrix, thus accelerating the extraction process (Petkova et al., 2018). Similarly, several studies have used ultrasound-assisted extraction (UAE) which employs ultrasonic waves to disrupt cell walls, facilitating the release of inulin into the solvent (Castellino et al., 2020; Esmaeili et al., 2021; Li et al., 2018). Accelerated solvent extraction (ASE) utilizes high temperature and pressure to increase solvent penetration and solubilization of inulin, making it a faster alternative to conventional methods (Saengkanuk et al., 2011). Recent research conducted by Mariano et al. (2023) revealed that the autoclave method can be successfully utilized for inulin extraction. These advanced techniques not only reduce the extraction time but also improve the efficiency and yield of inulin, making them valuable tools for inulin extraction from plant materials.
TLC is a simple, basic, and direct method that can be used in fructan analysis. Reiffová and Nemcová (2006) developed a rapid and simple qualitative TLC method for the identification of fructans in biological samples using silica gel TLC plates and butanol/ethanol/water mobile phase. The visualization of fructan spots in this method can be achieved by spraying a diphenylamine-aniline-phosphoric acid solution (Mudannayake et al., 2015b). As inulin is a complex mixture of different DP fractions, they are separated through a TLC plate, giving a characteristic blue-black spot pattern (Fig. 4). The main advantages of TLC methods include simple sample preparation, the possibility of crude samples, and simultaneous analysis of many samples. TLC is, however, limited to fructans with DP less than 10 and cannot be used as an accurate and reliable method for quantification of fructans (Matros et al., 2019).
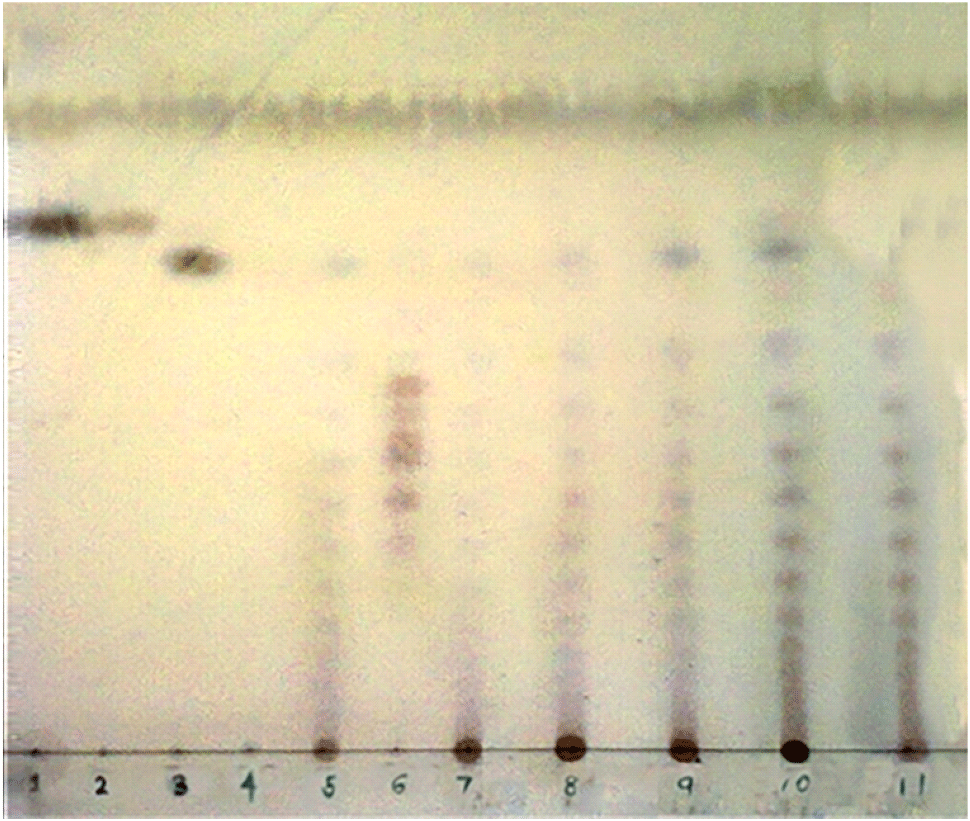
Even though TLC is used to visually track and characterize various components, technological advancements have evolved TLC into high-performance thin-layer chromatography (HPTLC), which offers significant potential for both qualitative and quantitative analysis. HPTLC is an efficient analytical technique for quantifying inulin fructans (Kirchert and Morlock, 2018; Simonovska, 2000; Singh et al., 2021). The process involves extracting and preparing inulin-containing samples in a suitable solvent, followed by applying them onto an HPTLC plate. The plate is developed in a mobile phase that separates the inulin fructans, which are then visualized using derivatization reagents like aniline diphenylamine phosphoric acid. Quantification is achieved by scanning the plate with a densitometer or appropriate imaging system, measuring the intensity of the spots, and comparing them to a calibration curve of known standards. HPTLC is advantageous for its ability to handle multiple samples simultaneously, cost-effectiveness, and provision of both qualitative and quantitative data (Singh et al., 2021; Zhou et al., 2014).
The enzymatic digestion followed by HPLC detection of sugars can be performed successfully for fructan quantification (Mudannayake et al., 2015a; Mudannayake et al., 2016). Quantification of inulin using HPLC and a refractive index (RI) detector has been reported by Bohacenko and Pinkrova (2014), Galdón et al. (2009), Redondo-Cuenca et al. (2021), Vendrell-Pascuas et al. (2000), Wang et al. (2010), and Wienberg et al. (2021). HPLC has also been used in combination with many other detection methods such as evaporative light scattering detection (Li et al., 2013; Perović et al., 2022) and charged aerosol detection (Li et al., 2014) to determine inulin content in food and plant samples. Hoebregs (1997) showed an indirect fructan quantification method using HPLC which involved treating samples with amyloglucosidase and inulinase (fructanase) enzymes and three independent determinations of the released sugars by HPLC-RID method (Fig. 5). The AOAC official method of fructan determination (AOAC 997.08) is based on the study of Hoebregs (1997). This method specifically allows the accurate quantitative determination of inulin in plant and food samples (Mudannayake et al., 2015a).
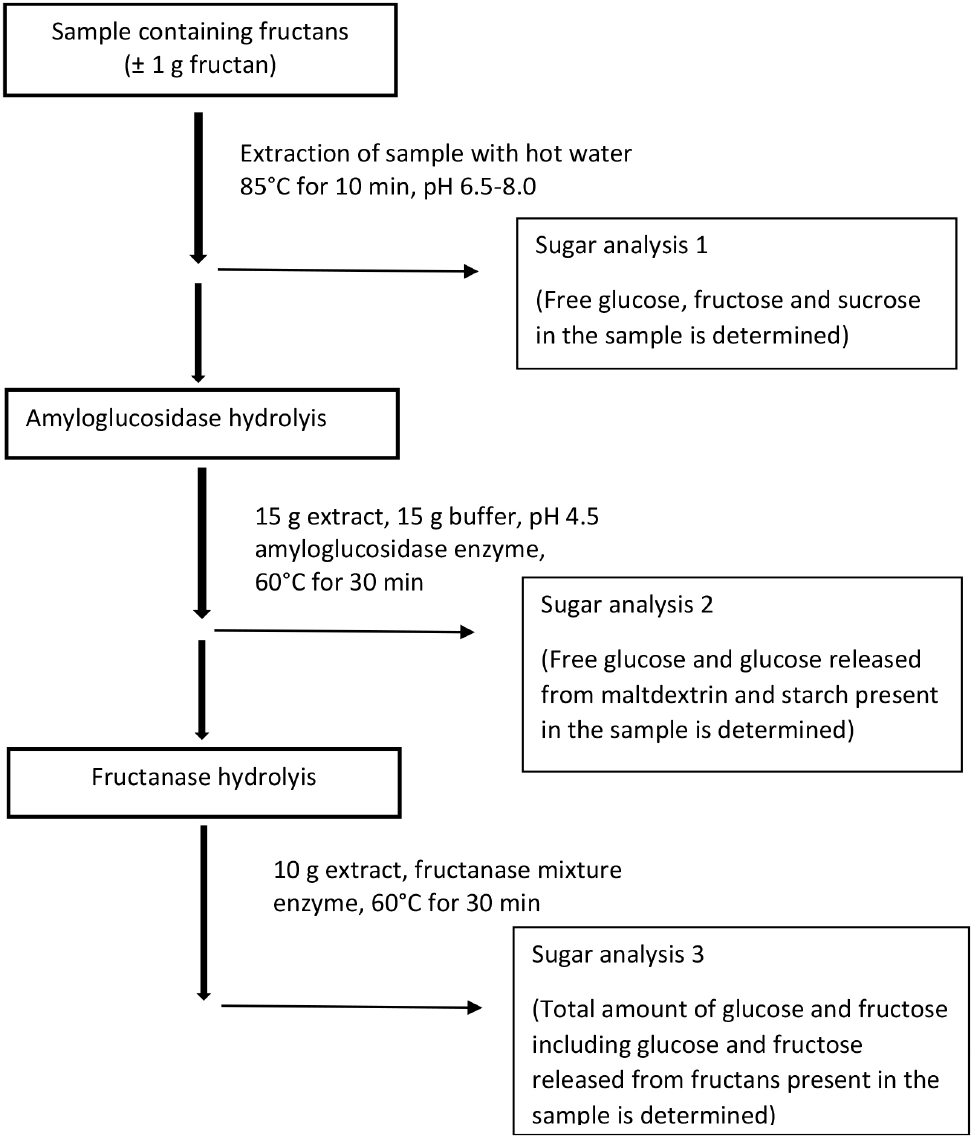
HPAEC-PAD has been accepted as the most powerful method for the determination of inulin fructans (Tran et al., 2022). It can be used for quantification as well as illustration of DP profiles of inulin (Apolinário et al., 2014; Benkeblia, 2013). Several studies have demonstrated that the HPAEC-PAD method can be used directly to obtain DP profiles of inulin from different inulin-accumulating plants. DP profiles of many plant-derived inulins, including chicory, Jerusalem artichoke, globe artichoke, dahlia, cardoon (Cynara cardunculus), and onion (A. cepa) had been elaborated using HPAEC-PAD method (Bedzo et al., 2021; Kriukova et al., 2017; Padalino et al., 2017; Pöhnl et al., 2017; Ronkart et al., 2007). However, this method is difficult to use directly for the quantification of fructans due to the less availability of appropriate standards and poor resolution of high DP polymers (Barclay et al., 2016). The HPAEC-PAD method of fructan quantification is considered time-consuming and expensive since anion exchange analytical columns are of relatively high cost (Saengkanuk et al., 2011).
Several studies have shown that fructan polymers can be successfully quantified using a UV-visible spectrophotometer. This method involves enzymatic hydrolysis of inulin into fructose and glucose and subsequent derivatization of released simple sugars with various chemical reagents (McClearly et al., 2019; Mudannayake et al., 2015a; Mudannayake et al., 2015b; Saengkanuk et al., 2011; Steegamans et al., 2004). The enzymatic-spectrophotometric method introduced by McCleary et al. (2000), based on the para-hydroxy benzoic acid hydrazide (PAHBAH) reaction, is accepted as an accurate and reproducible method for quantification of fuctans. The principle of this method involves that all the sucrose, maltose and starch present in samples are converted to simple monosaccharides by treatment with sucrase and amylase enzymes. The free monosaccharides present and derived from sucrose, maltose, and starch are then removed by converting them into sugar alcohols (borohydride reduction). Then, extracts are treated with fructanase mixture (exo-inulinase, endo-inulinase, and endo-levanase) enzyme, where fructans are enzymatically broken down to fructose and glucose. The glucose and fructose released from fructan are measured spectrophotometrically after reacting with PAHBAH (AOAC, 2016). Fig. 6 illustrates the principle of the enzymatic-spectrophotometric fructan assay method.
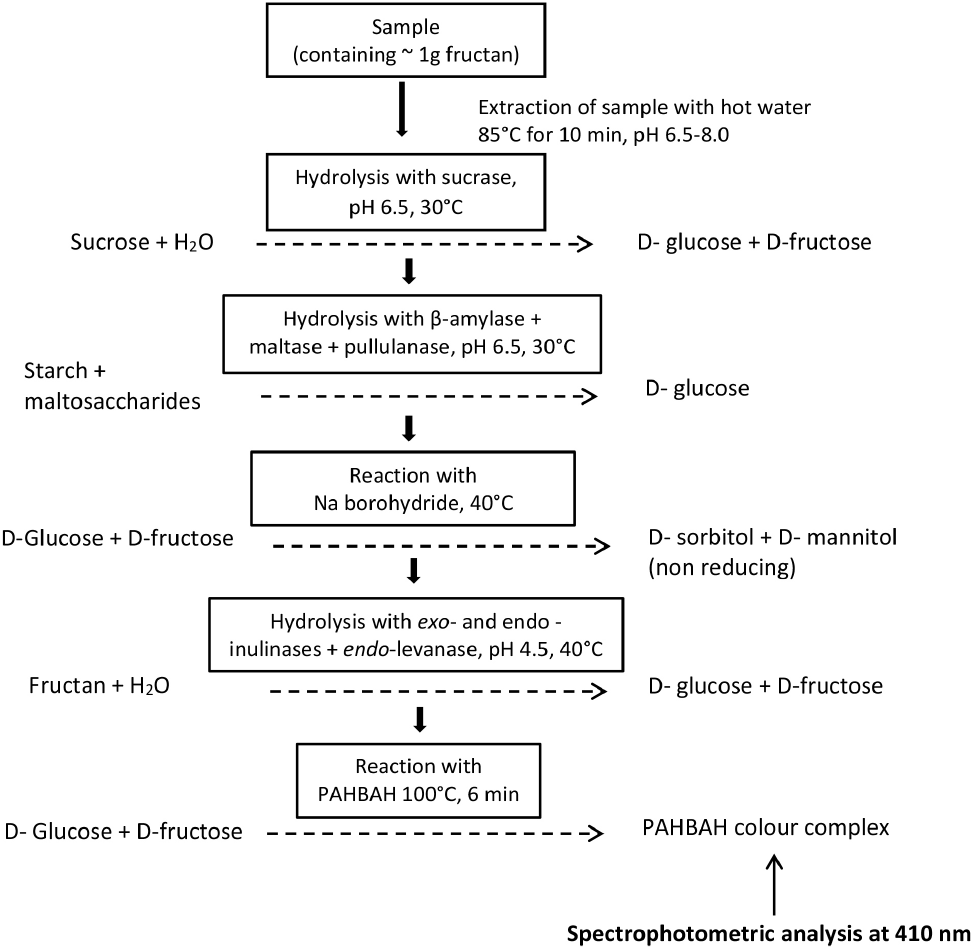
This method is being used by the AOAC as the method for the measurement of total fructans in foods (AOAC 999.03; AOAC, 2016). Furthermore, the current Megazyme fructan assay kit is based on the same method (Megazyme, 2020). This spectrophotometric method is considered an accurate, reliable, and specific method to determine fructan content in food/plant samples, provided that highly purified and specific enzymes are used to hydrolyze sucrose, starch, and fructans (McClearly et al., 2000; McClearly et al., 2019).
Quantitative determination of inulin fructans can also be conducted using gas chromatography (Joye and Hoebregs, 2000). Judprasong et al. (2011) analyzed 47 varieties of Thai food plants for quantity of inulin. They enzymatically hydrolyzed inulin according to the AOAC 997.08 method and determined the levels of released sugars using the GC technique.
GC can be easily coupled to mass spectrophotometers (GC-MS) allowing the analysis of complex mixtures. However, the relatively low volatility of carbohydrates has made GC analysis generally limited to derivatized sugars of molecular weight less than 4 DP (Matros et al., 2019).
Inulin analysis using the colorimetric method has been documented by various researchers for determining inulin content in plant materials (Li et al., 2018; Redondo-Cuenca et al., 2021; Wang et al., 2010). The colorimetric method of inulin analysis involves determining the total carbohydrate content using the phenol-sulfuric acid or anthrone method, with fructose or inulin as the standard. The reducing sugar content of the same sample is then analyzed using the dinitrosalicylic acid (DNS) method (Jain et al., 2020; Wood et al., 2012). The inulin or inulin oligomer content is calculated as the difference between the total carbohydrates and reducing sugar content (Wang et al., 2010). This method is widely used by researchers to quantify inulin owing to its simplicity and the use of cost-effective reagents. However, the colorimetric method has lower accuracy and specificity, as other sugars such as sucrose, hexoses, and glucan-like compounds present in the sample can interfere, leading to an overestimation of inulin content (Wang et al., 2010).
7. Conclusions
In conclusion, the extensive research conducted over the past two decades highlights the diverse health advantages of inulin fructans, particularly in enhancing bifidogenic effects and saccharolytic fermentation within the colon.
Inulin fructans offer multifaceted health benefits such as facilitating mineral absorption, combating intestinal infections, allergies, and inflammation, and potentially reducing the risk of colon cancer, cardiovascular diseases, and diabetes.
Beyond its health benefits, inulin exhibits diverse organoleptic and technological properties, making it a sought-after component in food systems. Its versatility as a fat and sucrose replacer, texture and viscosity modifier, dietary fiber enhancer, and sensory enhancer has spurred its incorporation across various food industries, including dairy, bakery, meat, and beverage sectors. The utility of inulin fructans extends beyond the food, with emerging applications in pharmaceuticals, pet foods, and microencapsulations.
Despite its growing popularity, the effective utilization of inulin depends upon accurate quantification, which remains a challenge due to the slow development of analytical methods for determining inulin-fructan content. Addressing this gap will be important in maximizing the potential use of inulin across diverse applications and ensuring its continued integration into functional foods and beyond.