1. Introduction
Mung bean sprouts are grown from mung bean seeds that have been soaked and sprouted under ambient temperature and high humidity (Warriner and Smal, 2014). Germinated sprouts can improve the nutrient quality of seeds and their digestion ability, since nutrients such as storage proteins, lipids, and carbohydrates are broken down to smaller sizes after sprouting (Ganesan and Xu, 2018). Mung bean seeds can also be eaten, both raw and cooked. Sprouts are consumed raw in western countries due to their high nutrient content and texture (Yang et al., 2013). Mung bean sprouts are also eaten in salads or sandwiches to enjoy their crispy texture in the Philippines (Salvador et al., 2021). According to the report of Gallup Korea (2022), 30.4% of the 942 participants reported consuming mung bean sprouts in their raw form (Son et al., 2013).
In the USA, the main pathogen contributed to foodborne outbreaks in sprouts was Salmonella spp. from 2011 to 2022. Additionally, 2 deaths were caused by L. monocytogenes in mung bean sprouts in 2014 (CDC, 2024). Mung bean sprouts undergo germination in environments characterized by ambient temperatures ranging from 22 to 28°C and high humidity levels, providing ideal conditions for the proliferation of microorganisms on the seed surface (Warriner and Smal, 2014).
This conducive environment increases the likelihood of pathogen internalization during germination (Martínez-Villaluenga et al., 2008). In such cases, merely washing sprouts before consumption is ineffective at eliminating pathogens. It is commonly acknowledged that outbreaks linked to sprouts from seeds tainted with pathogen rather than contamination occurring after production. Therefore, it is imperative to implement rigorous control measures aimed at reducing contamination at the seed level.
The US Food and Drug Administration recommended the use of 20,000 ppm calcium hypochlorite to treat seeds for 15 min (USDA, 1999). However, this treatment was unwelcomed in the sprout industry due to worker safety problems and unfavorable environmental impacts (Ding et al., 2013). Moreover, chlorine is ineffective at eliminating pathogens completely from seed surfaces if the initial bacterial load is high (Yang et al., 2013). Various studies have demonstrated the use of combined plasma-activated water (PAW) with ultrasound to inactivate Salmonella spp. and L. monocytogenes on mung bean seeds or the use of combined heat and relative humidity to inactivate microbial contamination in mung bean seeds before germination (Kim and Rhee, 2018; Machado-Moreira et al., 2021). A recent study by Darmanin et al. (2021) revealed a decrease significantly (p<0.01) in total aerobic bacteria (TAB) on mung bean sprouts, quantified as a 2.45 log CFU/g decrease, after four days of germination. This reduction was achieved through the combined treatment of mung bean seeds with air cold atmospheric plasma (CAP) and air PAW irrigation compared to untreated sprouts. Another research announced that treating mung bean seeds with cold plasma for 1, 3, or 6 min resulted in reductions in TAB of 0.29, 5.11, or 6.80 log CFU/g, respectively, after four days of germination (Cui et al., 2022).
When the control measures to inactivate pathogens are introduced in the food industry, the efficacy of time and cost should be considered, as well as safety. Previous studies have shown that combined treatments with various control measures, such as mild heat, SAEW, and UVC LED, can more effectively reduce various pathogens on various produce than a single treatment (Han et al., 2021; Koide et al., 2011; Luo and Oh, 2016). Moreover, Green et al. (2018) demonstrated that UVC LEDs within the 259-275 nm wavelength range are effective in significantly reducing most pathogens.
We evaluated the efficacy of multiple treatments, both individually and in combination, for reducing the presence of Salmonella spp. and L. monocytogenes on mung bean seeds during the soaking phase. Additionally, the influence of these treatments on inhibiting pathogen proliferation during germination process of mung bean sprouts derived from treated seeds was investigated.
2. Materials and methods
The sprouting equipment (24.2×24.2×32.5 cm) (ZC-A1001, Zaigle, Seoul, Korea) is shown in Fig. 1, which was utilized for germinating the mung bean seeds. Non-treated and treated seeds for disinfecting Salmonella spp. and L. monocytogenes were evenly placed on sprouting tray on the main body. The bottom of the main body was filled with sterile distilled water, and then the equipment was operated with a recycling system and irrigated with water for 2 min every 1 h. The sterile distilled water was changed daily. The sprouting equipment was placed in a temperature humidity chamber (VS-1203P1-H, Vision Scientific, Daejeon, Korea) and kept at 25°C with 85% relative humidity for 4 days.
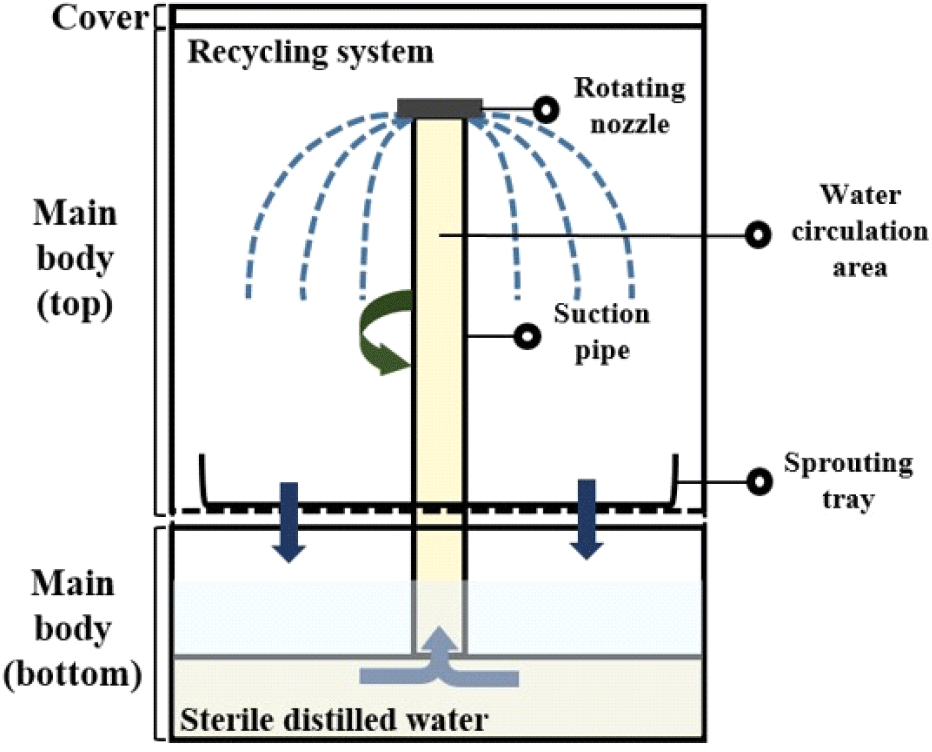
To decontaminate the microorganisms in the sprouting equipment after collecting the mung bean sprouts from the equipment, 300 mL of 3.6% hydrogen peroxide was placed into the bottom of the sprouting equipment for 4 min using a circulation system. Hygiene indicator bacteria levels in the top and bottom spaces between the sprouting trays and the water circulation area of the sprouting equipment were analyzed after each treatment (Fig. 1).
Germination rates of mung bean seeds were evaluated to determine whether the treated seeds affected the germination rate in comparison with that of nontreated seeds. The numbers of seeds before and after germination (>1 cm in length) were counted, and the germination rates were calculated with the following equation (Kim and Rhee, 2018).
Salmonella bareilly strains isolated from carrots and soybean sprouts, as well as Salmonella enterica strains isolated from iceberg lettuce, were acquired from the Ministry of Food and Drug Safety. These strains were cultured in brain heart infusion broth (BHI; Oxoid, Basingstoke, Hampshire, UK) for further experimentation. The isolated strains of L. monocytogenes were derived from enoki mushrooms and smoked salmon (serotype 1/2a) in Korea. These strains were incubated in tryptone soya broth (TSB; Oxoid) with 0.6% yeast extract (Oxoid). Each cell pellet was harvested using a centrifuge (VS-550, Vision Scientific) at 2,952 ×g for 10 min, and then washed with 10 mL of 0.1% sterile peptone water (Becton, Dickinson and Company, Sparks, NV, USA) in a 50 mL conical tube (SPL Life Sciences, Pocheon, Korea). The cocktail suspension for inoculation was serially diluted with sterile 0.1% sterile peptone water. Then, 10 mL of the diluted cocktail suspension was combined with 990 mL of 0.1% sterile peptone water in a sterilized beaker.
Mung bean seeds were obtained from Jeungan-Ri Hurb Village Co. (Yangpyeong, Korea). Seeds (20 g, noncracked and nondecayed) were washed three times with 300 mL of sterile distilled water using a sterile magnetic stir bar to remove dirt and foreign materials. Mung bean seeds were drained and then air-dried on a clean bench (VS-1400LSN, Vision Scientific) for 40 min to completely remove water from the seed surfaces. Seeds (100 g) were evenly contaminated by immersion in 1 L of each cocktail suspension of Salmonella spp. or L. monocytogenes. The plants were stirred with a magnetic stirrer (MSH-20D, DAIHAN Scientific Co., Ltd., Wonju, Korea) for 2 min. After inoculation, the mung bean seeds were placed in sterile stainless steel trays and then air-dried on a clean bench for 40 min to attach bacteria to the seed surfaces. The initial levels of contamination in mung bean seeds for Salmonella spp. or L. monocytogenes were approximately 3.5-4 log CFU/g.
Ultraviolet-C light-emitting diode (UVC LED, 275 nm), consisting with five modules (BlueLumi, Yongin, Korea), were attached to bar-type electronic printed circuit boards (PCBs, 45 cm). The treatment of UVC LED was conducted in a chamber (500×400×520 mm) equipped with two PCBs. A direct current voltage of 500 mA was supplied to the PCBs from a power supply (TY-1006, Tae Young Electronics Co., Ltd., Yongin, Korea). The intensity of UVC radiation from the UVC LEDs was measured to be 23 μW/cm2 using a UV light meter (UVC-254SD, Lutron Electronics Co., Inc., Coopersburg, PA, USA) calibrated for a wavelength range of 240-390 nm (Lee et al., 2021). Inoculated seeds (10 g) were arranged in a monolayer on a Petri dish (SPL Life Sciences Co.) for even irradiation. Then, the petri dish was placed in the UVC LED chamber for 5 min with a 6 cm distance between the UVC LED and each sample.
SAEW was generated using a SAEW generator (BC-120, Cosmic Round Korea Co., Seongnam, Korea) consisting of a non-membrane electrolytic chamber with anode and cathode electrodes. The available chlorine concentration (ACC) of SAEW (pH 5.5) was 30 ppm, which was determined using chlorine test paper (Toyo Roshi Kaisha, Ltd., Tokyo, Japan). Contaminated seeds (25 g) on the stainless steel mesh were immersed in a beaker containing 225 mL of 30 ppm SAEW for 5 min.
Ultraviolet-C waterproof light-emitting diodes (UVC W-LED; 275 nm, 48 V; BlueLumi) consisting of four modules in a stainless steel case (32.5×17.5×15 cm) were used (Fig. 2). Among these four UVC W-LED modules, two modules were equipped on opposite sides, and the others were placed at the bottom of the stainless-steel case (Kim et al., 2021). To evaluate the effect of the UVC W-LED, inoculated mung bean seeds were placed in stainless steel equipped with UVC W-LED modules and tap water for 3 or 5 min.
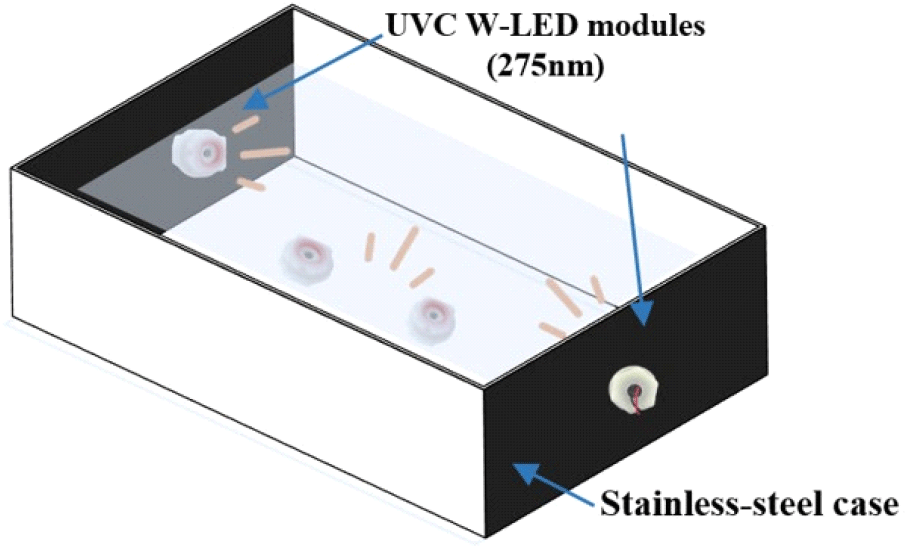
A 225 mL aliquot of sterile distilled water was placed in a 250 mL beaker, which was heated using a water bath (SB-1200, Eyela, Tokyo, Japan) to reach a temperature of 60°C. The temperature of the middle of the sterile distilled water was measured with a thermometer. A stainless mesh (T1019, Herb Garden, Daejeon, Korea) containing the inoculated mung bean seeds was then submerged in sterile distilled water (60°C) for 1 or 3 min. While the seeds were submerged, the temperature of the water bath was consistently monitored.
Combined treatment of 30 ppm SAEW with either UVC W-LED or mild heat was used for mung bean seeds. To estimate the effect of combined treatment with SAEW and the UVC W-LED, SAEW was put into a stainless-steel case equipped with four UVC W-LED modules as described above. Contaminated mung bean seeds were submerged in SAEW and concurrently irradiated for 3 or 5 min. To determine the effect of SAEW combined with mild heat at 60°C, SAEW was heated in a water bath. The temperature of the SAEW was monitored with a thermometer. Contaminated mung bean seeds in the stainless mesh were immersed in heated SAEW (60°C) for 3 or 5 min.
For microbiological analysis of mung bean sprouts, approximately 6 cm of the root was removed as a noncommercial part (personal communication with farmers of mung bean sprouts). Ten grams of mung bean seeds and sprouts were homogenized with 0.1% sterile peptone water using a stomacher (BagMixer, Interscience, St Nom la Bretêche, France) for 2 min. Serially diluted solution with 0.1% sterile peptone water was spread onto a Brilliance Salmonella agar base with Salmonella selective supplement (Oxoid) for Salmonella spp. and Polymyxin Acriflavin LiCI Ceftazidime Esculin Mannitol (PALCAM) agar with PALCAM selective supplement (Oxoid) for L. monocytogenes. After the plates were placed in an incubator (ELELA, Vision Scientific) at 37°C for 24 h and 48 h, each pathogen was calculated using a colony counter (Scan 1200, Interscience).
Hygiene indicator bacteria (total aerobic bacteria, coliform and E. coli) from mung bean seeds and sprouts were investigated in line with the methods of the Korean Food Code (MFDS, 2023). Each sample (25 g) of mung bean seeds and sprouts was pummeled with 225 mL of sterile 0.1% sterile peptone water using a stomacher for 2 min in a filter bag. The homogenized solution was diluted in 0.1% sterile peptone water. Each 1 mL aliquot from each sample was inoculated on 3M™ Petrifilm™ (3M Corporation, St Paul, MN, USA) aerobic count (AC) and E. coli /coliform (EC) plates, followed by incubation at 37°C for 48 h and 24 h, respectively. Colonies on the incubated plate were counted with a 3M™ Petrifilm™ Plate Reader Advanced (3M PPRA; 3M Corporation).
To prohibit the cross-contamination following germination of mung bean seeds, we also analyzed hygiene indicator bacteria from the top, bottom, and interior (water circulation area; see Fig. 1) of the sprouting equipment. This comprehensive analysis aimed to ensure the maintenance of hygienic conditions throughout the sprouting process and equipment usage. The bottom and top of the equipment were analyzed by pouring 0.1% sterile peptone water into the bottom of the main body and from the top to rinse the sprouting tray, respectively. To evaluate the water circulation area in the equipment, 300 mL of 0.1% sterile peptone water was added to the bottom. Subsequently, the sprouting equipment was operated for 2 min, which corresponded to one complete water circulation cycle. 30 mL of 0.1% sterile peptone water was collected from each part and put into a 50 mL conical tube for analysis of hygiene indicator bacteria.
For statistical analysis, all experiments were processed in triplicate. Significant differences between samples or among groups were evaluated using a t-test or one-way analysis of variance (ANOVA) followed by Duncan’s multiple range test, utilizing SAS software version 9.4 (SAS Institute, Inc., Cary, NC, USA), respectively. Statistical significance was regarded as p<0.05.
3. Results and discussion
Germination rates are very essential for the quality of mung bean sprouts. The effects of various treatments on the germination rates of mung bean seeds were investigated (Table 1). The germination rates of mung bean seeds in the various treatment groups were not significantly (p>0.05) different from that of untreated (control) mung bean sprouts (98.44%), except for those in the UVC LED treatment group (94%). The application of the UVC LED had a significant (p<0.05) impact on the germination rate of mung bean seeds, indicating that the UVC LED treatment was not suitable for maintaining the quality of mung bean seeds. However, when UVC waterproof LEDs were used in tap water or SAEW, there was no observable effect on the germination rate. This suggests that dipping mung bean seeds into tap water or SAEW could not only stimulate germination but also effectively sanitize the seeds. Trząskowska et al. (2018) reported that mung bean seeds treated with mild heat at 60°C for 10 min (93.59%) or 20 min (92.5%) exhibited germination rates that were not significantly different (p>0.05) from those of untreated seeds (98.2%). Similarly, Zhang et al. (2021) demonstrated that alfalfa seeds immersed in 25, 35, and 45 ppm slightly acidic electrolyzed water (SAEW) had germination rates ranging from 90-92%, which were not significantly different (p>0.05) from those of seeds soaked in tap water for 6 h. Consistent with these findings, our study also indicated that neither mild heat nor SAEW treatment significantly impacted the germination rate of mung bean seeds.
The effects of different treatments on the levels of hygiene indicator bacteria were analyzed in mung bean seeds before germination and in mung bean sprouts after germination (Table 1). Prior to germination, the contamination level of total aerobic bacteria (TAB) in mung bean seeds was 2.30 log CFU/g. Following treatment with SAEW combined with UVC W-LED for 5 min or mild heat for 3 or 5 min, TAB was not detected in the seeds. Additionally, coliform and E. coli were absent in the mung bean seeds irrespective of the applied treatments. Post-germination, the levels of TAB and coliform bacteria in the sprouts grown from treated seeds exceeded 7 log CFU/g, although E. coli remained undetectable in the sprouts. No significant differences were detected in the TAB levels among mung bean sprouts germinated from variously treated seeds. However, a combined treatment of SAEW and mild heat for 3 and 5 min on the seeds significantly reduced coliform growth to 7.17 and 7.10 log CFU/g, respectively, in the sprouts. This reduction was notable compared to that of the control group, which exhibited a coliform concentration of 7.71 log CFU/g (p<0.05).
The primary contributor to the high microbiological load in sprouts is the natural microflora of seeds, which thrive in a germination environment characterized by ambient temperatures of 22-28°C and high humidity (NACMCF, 1999; Warriner and Smal, 2014). Additionally, the high nutrient content of mung bean seeds facilitates the growth of natural bacteria during germination (Ganesan and Xu, 2018). As a result, the TAB and coliform levels in mung bean sprouts are approximately 7.9 and 7.8 log CFU/g, respectively (Mustar and Noh, 2013). A study by Rossi and Lathrop (2019) revealed that the population of TAB in alfalfa sprouts increases significantly—by approximately 5.1 log CFU/g—within five days of germination. Similarly, Tornuk et al. (2011) reported that TAB levels in wheat seeds, initially recorded at 5.77 log CFU/g, increased to 9.00 log CFU/g after nine days of germination. These findings collectively underscore the phenomenon where both TAB and coliform populations in seeds can experience substantial growth during the germination process.
In this study, mung bean seeds treated with SAEW+UVC W-LED and mild heat before germination exhibited no detectable levels of TAB or coliforms. However, despite these treatments, the growth of TAB and coliform bacteria was not prevented during the germination of mung bean sprouts. These findings indicate that while various control measures were generally ineffective at inhibiting the growth of TAB and coliform bacteria in mung bean sprouts during germination, the combined treatment of SAEW and mild heat applied to the seeds marginally reduced the proliferation of coliform bacteria during the germination process.
Castro-Rosas and Escartin (2000) reported that when alfalfa seeds were treated with sodium hypochlorite (100 mg/L free chlorine), the TAB concentration decreased slightly from 3.1 to 1.6 log CFU/g, and the coliform concentration decreased from 1.6 to <1 log CFU/g. However, despite these reductions in the microbial load in the seeds, the levels of TAB and coliforms increased up to 9 log CFU/g in the alfalfa sprouts after 3 days of germination, regardless of treatment. This finding aligns with the results of the present study, indicating that the growth of hygiene indicator bacteria was not prevented in sprouts under the germination environment, even after the effective reduction of microorganisms in the seeds. Reducing microbial levels in seeds is crucial for producing microbiologically safe sprouts, but it is equally important to control microbial contamination in the equipment used for sprouting. This dual approach helps ensure the overall safety of the sprouts throughout the production process.
Since the ambient temperature (22-28°C) and high humidity (85%) during the germination process provide favorable conditions for microorganisms, sanitizing the equipment is important after each use to prevent cross-contamination. In this study, no hygiene indicator bacteria were found in any section—top, interior, or bottom—of the sprouting equipment. Specifically, the interior, which functions as a water circulation area for germination and is treated with a 3.6% hydrogen peroxide solution, showed no detectable presence of such bacteria.
Contaminated seeds have been noticed as the primary source of foodborne diseases associated with sprouts due to the conditions present in germination environments, as noted by the National Advisory Committee on Microbiological Criteria for Foods (NACMCF) in 1999. Fu et al. (2008) conducted a study in which alfalfa seeds contaminated with Salmonella spp. (-2.28 log MPN/g) were irrigated either every 24 h or every 4 h. After two days of germination, the population of Salmonella in seeds irrigated once every 24 h increased to 1.76 log MPN/g, whereas in seeds irrigated every 4 h, it was only 0.76 log MPN/g. These findings highlight the importance of controlling irrigation frequency, as well as monitoring temperature and relative humidity, during the germination of sprouts to reduce microbial growth.
Fig. 3 illustrates the reduction effects of various treatments on populations of Salmonella spp. and L. monocytogenes in mung bean seeds before germination. In general, the populations of both Salmonella spp. and L. monocytogenes exhibited significant decreases (p<0.05) following treatment with various control measures compared to untreated mung bean seeds. Notably, the combined treatments demonstrated greater reducing effects than did the single treatments. The whole surface of the mung bean seeds was disinfected by immersion in SAEW equipped with a UVC W-LED. It was found to be more effective in reducing Salmonella spp. and L. monocytogenes than irradiation with a UVC LED alone. Combined treatment with SAEW and UVC W-LEDs was also more effective at reducing S. aureus and L. monocytogenes in fresh-cut fruits than single treatment with SAEW (Kim et al., 2021). The most substantial reduction in Salmonella spp. (2.67 log CFU/g reduction) was observed in mung bean seeds treated with the combined application of SAEW and mild heat at 60°C for 5 min, followed by the same treatment for 3 min. Conversely, no significant differences in the reduction of L. monocytogenes were observed between the combined SAEW+UVC-W-LED or mild heat treatments. However, the most pronounced reduction in L. monocytogenes (2.10 log CFU/g reduction) was achieved with the combined treatment of SAEW and mild heat at 60°C for 5 min. Overall, significantly greater reduction effects on Salmonella spp. were noted compared to L. monocytogenes with single mild heat treatments (3 or 5 min) or combined treatments with SAEW (5 min).
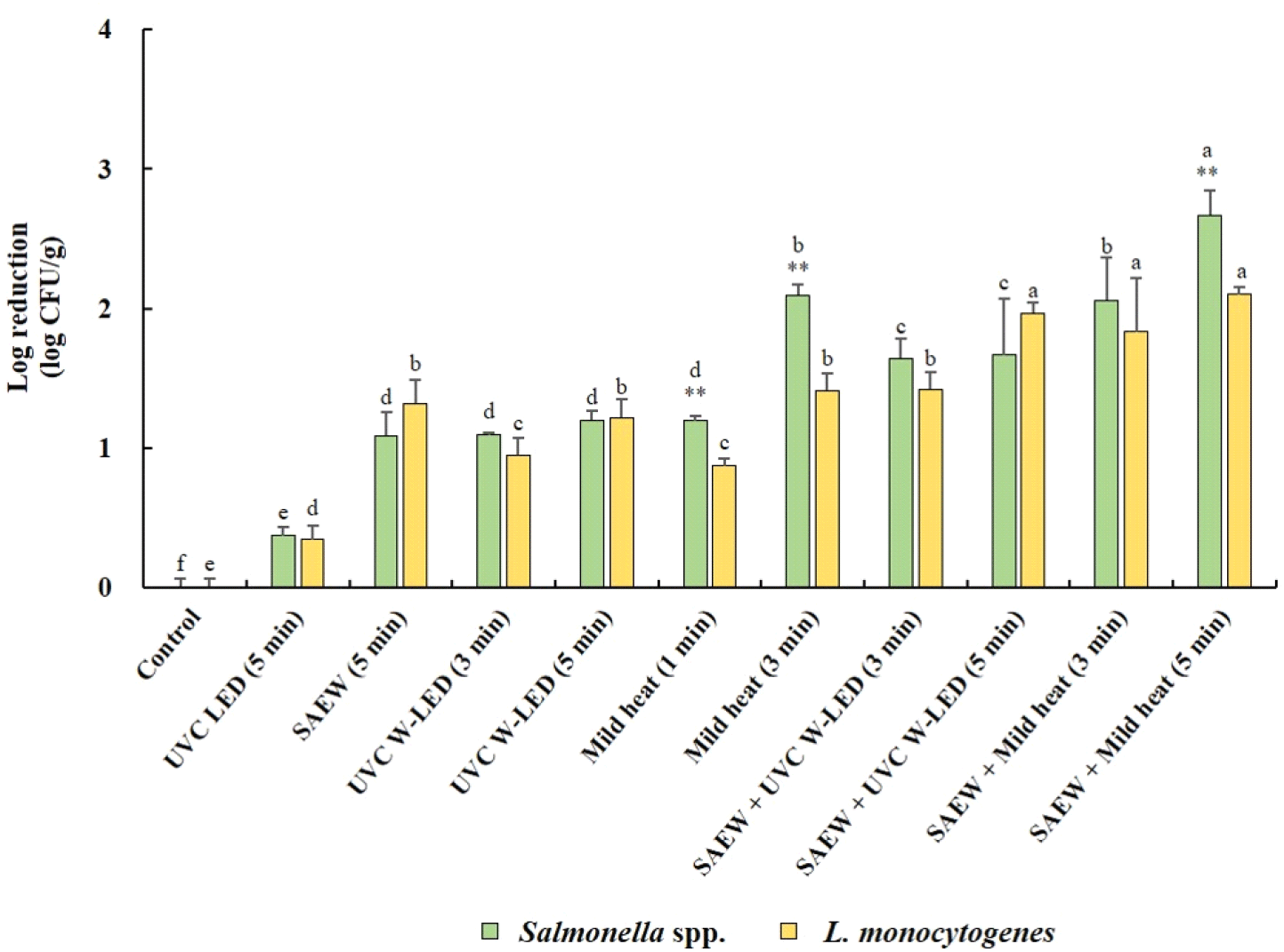
Fig. 4 shows how different treatments impact the growth of Salmonella spp. and L. monocytogenes in mung bean sprouts during a 4 days germination period. These findings revealed that despite treatment, neither Salmonella spp. nor L. monocytogenes were entirely inhibited in mung bean sprouts during germination. In untreated mung bean seeds (control), initial populations of Salmonella spp. and L. monocytogenes were detected at 3.86 and 3.67 log CFU/g, respectively, increasing to 5.34 and 3.87 log CFU/g, respectively, in sprouts over the 4 days germination period. For untreated alfalfa seeds, the numbers of S. Typhimurium and L. monocytogenes in sprouts after germination increased by 4.34 and 3.39 log CFU/g, respectively (Fu et al., 2022).
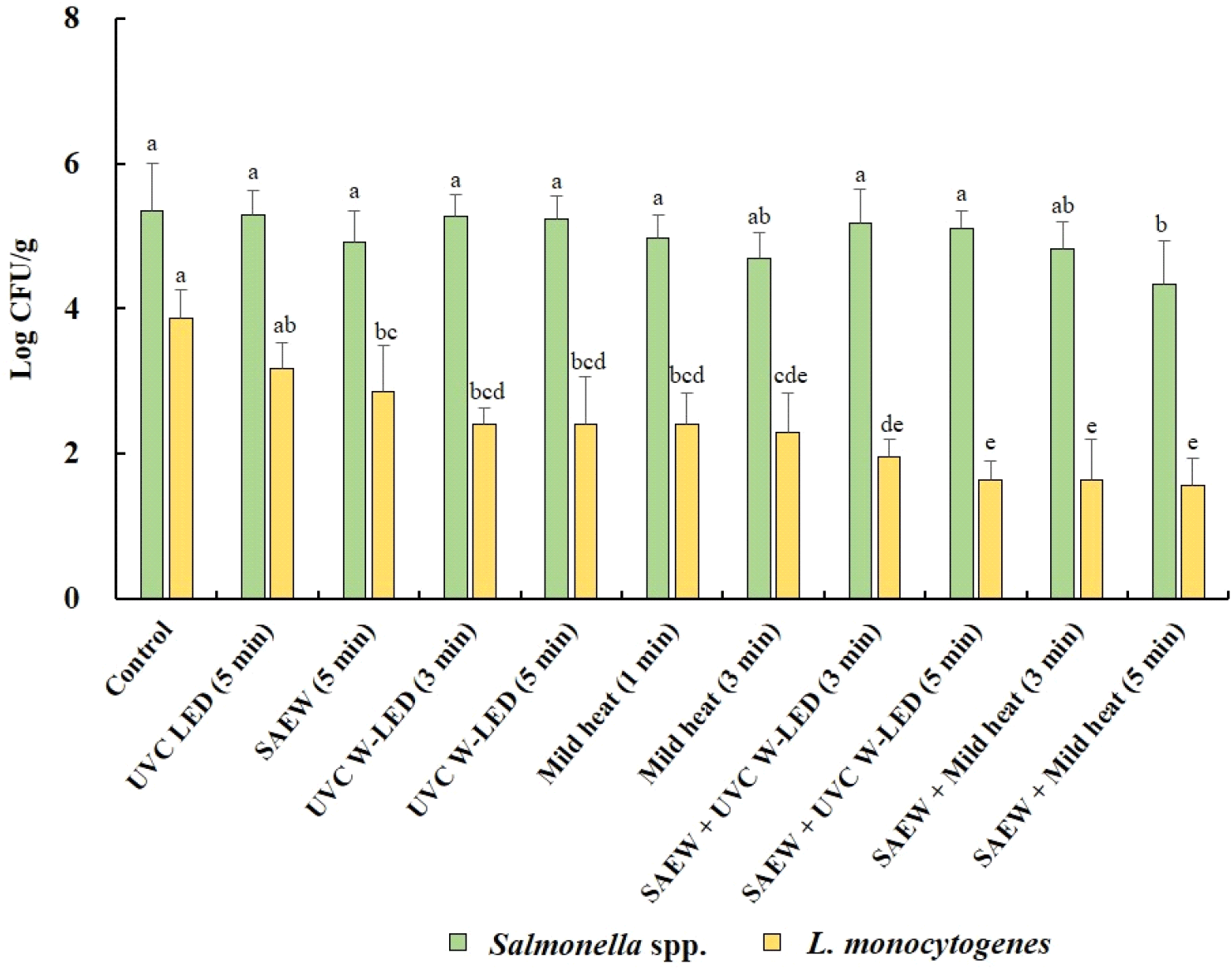
The population of Salmonella spp. also increased significantly (p<0.05) in mung bean sprouts across all treatment groups. Conversely, the populations of L. monocytogenes did not significantly increase (p>0.05) in any of the treated mung bean sprouts compared to the untreated mung bean seeds prior to germination. These results indicate that the pathogens remaining in mung bean seeds after treatment can proliferate during the germination process. Notably, while both pathogens demonstrated growth, the proliferation of Salmonella spp. in sprouts was more pronounced, indicating its support in sprouts during germination irrespective of treatment. The observed decrease in the growth of L. monocytogenes in sprouts compared to that of Salmonella spp. implies the greater potential for foodborne illness attributable to Salmonella spp. in sprouts available in the retail market. This is in line with previous studies, such as Callejón et al. (2015), which identified Salmonella spp. as the primary pathogen associated with foodborne illness outbreaks linked to sprouts. Furthermore, CDC reports (2024) on sprout-associated outbreaks in the USA from 2011 to 2023 confirmed a greater incidence of outbreaks caused by Salmonella spp. (62.50%) than by L. monocytogenes (6.25%).
Among the tested treatments, the combination of SAEW and mild heat at 60°C for 5 min was most effective at suppressing the growth of Salmonella spp. (4.34 log CFU/g) and L. monocytogenes (1.56 log CFU/g) in mung bean sprouts. Furthermore, the combined treatment of seeds with SAEW + mild heat or UVC W-LED also effectively suppressed the growth of L. monocytogenes in sprouts. Therefore, treating mung bean seeds with SAEW combined with mild heat at 60°C or UVC W-LED is a promising method for effectively inhibiting the growth of L. monocytogenes in mung bean sprouts.
Considering that the initial contamination levels of both pathogens typically range from 3.6-3.8 log CFU/g in mung bean seeds (Iacumin and Comi, 2019), the synergistic effects of combining SAEW with UVC W-LED or mild heat present a compelling approach to reduce the risk of foodborne illness associated with consuming mung bean sprouts. Moreover, the combined treatment of SAEW with UVC W-LED, which concurrently irradiates and washes the seeds, offers the additional benefit of water conservation by facilitating water reuse and averting cross-contamination issues stemming from water (Kim et al., 2021). Consequently, immersing mung bean seeds in SAEW combined with UVC W-LEDs is an effective and practical method for pathogen inactivation within the sprout industry.
In this work, it is important to note that growth inhibition in mung bean sprouts was more pronounced for L. monocytogenes than for Salmonella spp. after mung bean seeds were treated with mild heat. The main difference between Salmonella spp. and L. monocytogenes is the thickness of the cell wall surrounding the cytoplasmic membrane. When bacteria are exposed to various treatments, the sites of cellular injury and extent of resistance to stress might differ according to the cell membrane of the bacteria (Shao et al., 2023). The thicker cell walls of gram-positive bacteria make them more heat tolerant than gram-negative bacteria, rendering them less susceptible to heat treatment. Previous research has shown that bacterial resilience to mild heat stress decreases due to the weakening of hydrogen and hydrophobic bonds in cell membranes at moderate temperatures (40-50°C). Consequently, the presence of hypochlorous acid (HOCl) in SAEW under mild heat conditions may enhance its ability to penetrate bacterial cytoplasmic membranes, thereby increasing its bactericidal effectiveness (Tango et al., 2015). Luo and Oh (2016) similarly reported that treatment of fresh-cut bell peppers contaminated with both pathogens with SAEW at a concentration of 28-30 mg/L combined with mild heat at 60°C for either 3 or 5 min resulted in a more significant reduction in S. Typhimurium than in L. monocytogenes. A similar pattern was observed in the current work.
The effects of pathogens on alfalfa seeds during germination have been documented in previous research (Adhikari et al., 2019; Cui et al., 2018). Notably, Adhikari et al. (2019) reported that the concentration of L. monocytogenes on alfalfa seeds increased significantly over the course of germination. Specifically, after five days of germination at 25°C, the bacterial load increased from an initial 3.44 log CFU/g to 7.6 log CFU/g. When alfalfa seeds were inoculated with S. enterica and EHEC and allowed to germinate for nine days at 25°C, both pathogens increased the CFUs of the sprouts by more than 4 log CFU/g after germination (Cui et al., 2018). These data indicate that the growth of both pathogens was more prominent in alfalfa seeds than in mung bean seeds following germination. The difference in the growth of pathogens between alfalfa and mung bean seeds can be attributed to variations in their surface morphology. The difference in pathogen growth between alfalfa and mung bean seeds is attributed to differences in their surface morphology. Specifically, the surface characteristics of seeds influence the adhesion strength of bacteria, influencing their ability to colonize and proliferate. For example, a study by Fan et al. (2017) examined the adhesion strengths of E. coli on various seeds and reported that the lowest bacterial populations were on mung bean seeds, which have smoother surfaces than other seeds, such as red clover and fenugreek. Furthermore, treatment of mung bean seeds with gamma radiation resulted in a greater reduction in the E. coli population than treatment of the other seeds. These findings suggest that smoother surfaces, such as those of mung bean seeds, present challenges for bacterial attachment. Consequently, control measures can more easily remove bacteria from surfaces with specific characteristics. Notably, mung bean seeds have smoother surfaces than alfalfa seeds (Fan et al., 2017; Holc et al., 2022). This surface difference likely contributes to the lower incidence of Salmonella spp. and L. monocytogenes growth observed in mung bean sprouts during germination than in alfalfa sprouts.
4. Conclusions
This study evaluated effectiveness of single and combined treatments on mung bean seeds to prevent the growth of Salmonella spp. and L. monocytogenes in mung bean sprouts during the germination process. Overall, various pretreatments on mung bean seeds did not significantly (p>0.05) affect germination rates. However, although they effectively reduced hygiene indicator bacteria in mung bean seeds, no significant (p>0.05) reduction of total aerobic bacteria in mung bean sprouts was observed after 4 days of germination regardless of the kind of treatment. The growth of Salmonella spp. and L. monocytogenes in mung bean sprouts during germination was completely not prevented irrespective of treatment. However, combined treatments significantly (p<0.05) inhibited pathogen growth during germination compared to the control (no treatment) and were particularly effective in mung bean sprouts contaminated with L. monocytogenes. Treating mung bean seeds with combined treatment of SAEW+ mild heat or UVC W-LED resulted in the highest reduction of Salmonella spp. on mung bean seeds and the least growth of Salmonella in mung bean sprouts during germination among tested treatments. In case of L. monocytogenes, treating seeds by UVC W-LED in SAEW or SAEW with mild heat at 60°C significantly (p<0.05) prevented the growth of L. monocytogenes in germinated sprouts. Since UVC W-LED can disinfect commodity and washing water at the same time, preventing cross-contamination caused by washing water is possible. Especially, soaking seeds with SAEW equipped with UVC W-LED might provide dual effects of disinfection and pretreatment of seeds. Thus, it can be recommended as a practical method in the sprout industry.