1. Introduction
Faba bean is a leguminous crop grown in countries like Australia, China, Ethiopia, France and the UK (Dhull et al., 2022). It can offer diversity to consumers (do Carmo et al., 2021) because of its high protein content (Maluk et al., 2022), which may vary between 26 and 40% (Coda et al., 2015; Labba et al., 2021; Rahate et al., 2021). Like most legumes, faba bean has nitrogen-fixing ability and is reported to have a high yield potential compared with other grain legumes (Khazaei et al., 2021). Although faba beans hold significant nutritional and agronomic value, their potential remains largely untapped. This limited use is linked to the presence of anti-nutritional compounds like vicine, convicine, glycosides, and pyrimidine (Arese et al., 2012; Pulkkinen et al., 2019). In the UK, faba beans are the most produced grain legume, nevertheless, unlike in other parts of the world where the grains are used as a food source, very little is used for human consumption and more for animal feeding (Sepngang et al., 2019).
Faba beans are a valuable source of starch (22-45%) that could be an alternative starch source to conventional corn and potato starches (Punia et al., 2019). Starch, in its native form, is typically unsuitable for many industrial applications because it lacks resilience to harsh processing conditions like high temperatures, intense shear forces, and strong acidity.
Therefore, modification is necessary to enhance starch functionality and application in the industry. Various modification techniques, including chemical, enzymatic, genetic, and physical approaches, and sometimes a combination of these, are commonly employed to modify starch (Oyeyinka et al., 2021a). According to Jane (1995), modification confers new functionality and enhances starch use in the industry. Previous studies on FBS modification focused on crosslinking (Dong and Vasanthan, 2020; Sharma et al., 2020), lintnerization with hydrochloric acid (Buléon et al., 1987), hydrolysis with enzymes during germination (El-Shimi et al., 1980), heat moisture treatment (Piecyk and Domian, 2021), gamma irradiation (Sofi et al., 2013) and dry heat treatment (Fasakin et al., 2024). Dry heat treatment, gamma irradiation, and heat moisture treatment are physical methods currently being encouraged for starch modification. These methods are environmentally friendly and suitable for producing sustainable and clean-label starch compared to chemical methods (Maniglia et al., 2021). Fasakin et al. (2024) reported that dry heat treatment of faba bean starch (FBS) under optimized temperature and time conditions resulted in an increase in peak, final, and setback viscosities but a decrease in amylose content. Furthermore, the study showed that FBS displayed a considerably higher ability to swell and improved pasting properties compared to the corn starch reference sample.
Other promising physical starch modification methods, such as annealing (Wang et al., 2017) and USN (Wang et al., 2020a) have also been found to produce modified starch with enhanced functionality. USN alters the physicochemical and structural properties of starch due to the generation of free radicals resulting from shear force and high temperature (Zhu, 2015). The extent of these changes is dependent on the botanical source of the starch, USN power, USN frequency, USN time, and USN temperature (Zuo et al., 2009). For example, the swelling power of various starches increased with increasing USN time (Jambrak et al., 2010; Sujka and Jamroz, 2013; Wang et al., 2020a). In terms of structure, ultrasound treatment reportedly increased the relative crystallinity of waxy maize starch (Chang et al., 2021). While previous research has explored the impact of USN on starches, the majority of these studies have focused on cereal starches such as normal maize, waxy maize, and wheat, as well as tuber starches like potato starch (Zhu, 2015). Studies on legume starches, such as mung bean (Shen et al., 2021), remain limited. This gap is significant given that the impact of USN on starch structure and functionality is known to vary based on the composition and botanical source of the starch. By examining the unique characteristics of FBS under USN, our study provides new insights into how this process influences its structural and functional properties, contributing valuable knowledge to the field of starch modification and expanding the understanding of legume-based starches. In this study, we hypothesize that the use of USN at varying times will produce starch with improved functionality compared to native starch. Therefore, this study examined the pasting, functional and structural characteristics of FBS subjected to varying USN time (5-60 min).
2. Materials and methods
Faba bean seeds were sourced from Elsoms Seeds Ltd, UK, and stored at room temperature in the laboratory until they were required for starch extraction. All chemicals utilized in this study were of analytical grade.
Starch was extracted from the seeds following the method outlined by Sharma et al. (2020). The extracted starch was dried in an oven (Binder GmbH, Im Mittleren Tuttlingen, Germany) for 24 h. After drying, the starch was sieved through a 150 μm aperture sieve and stored in Ziploc bags until required for analysis.
Starch samples, prepared in duplicate, were placed in test tubes labeled A to F. Each sample was combined with twice the amount of distilled water and then subjected to a digital ultrasonic bath (PS-100A, PTSG, Shenzhen, China) with a power rating of 600 W and frequency of 40 kHz. The amount of water added was relative to the initial moisture content of the starch, which was an average of 8.5%. Starch was ultrasonicated at 50°C (below the gelatinization temperature of FBS) with a frequency of 40 Hz at varying times of 0 (A), 5 (B), 15 (C), 30 (D), 50 (E), and 60 (F) min. The USN time selected was based on the varying time reported in the literature. After USN treatment, the starch samples were dried at 40°C for 12 h.
The color and morphology of native and modified FBS were determined as described by Oyeyinka et al. (2015), while amylose content was determined using the iodine binding method (Williams et al., 1970).
To a centrifuge tube of known weight, 1 g of the starch sample and 10 mL of water were added. The mixture was allowed to stand for 30 min, then centrifuged at 4,000 rpm for 15 min, and the supernatant was discarded. The weight of the tube and the residue was recorded, with the increase in weight considered as the water absorption capacity (mL/g).
The swelling power of the starches was determined using the method outlined by Oyeyinka et al. (2015). In summary, a 1% starch sample was dispersed in distilled water within a pre-weighed centrifuge tube. The mixture was then heated in a shaking water bath at temperatures of 50, 60, 70, 80, and 90°C for 30 min. After heating, the samples were centrifuged at 4,000 ×g using an Eppendorf Centrifuge (5702R, Eppendorf, Hamburg, Germany) for 30 min, and the supernatant was discarded. The swelling power was calculated by weighing the residue after centrifugation and dividing it by the initial weight of the starch on a dry weight basis.
The amount of water released by gelatinized FBS after storage, referred to as syneresis, was determined using da Silva Costa et al. (2020) method. A starch suspension was prepared for all samples by mixing starch with distilled water to create a 2% w/v solution. The suspension was gelatinized in a shaking water bath (OLS26 Aqua Pro, Grant Instruments Ltd, Cambridge, UK) at 85°C for 30 min, then allowed to cool to room temperature. Samples were stored for analysis on day 0, day 2, and day 6, followed by centrifugation (5810R, Eppendorf Biotech, Hamburg, Germany) at 3,000 rpm for 15 min. The supernatant was collected and weighed, and syneresis was calculated as a percentage by dividing the weight of the supernatant by the weight of the starch sample.
Pasting properties, such as peak, breakdown, trough, setback, and final viscosity as well as pasting temperature and peak time of the starch samples, were measured using an RVA Tecmaster instrument (Perten, Waltham, MA, USA) following the pre-installed general pasting method (AACC Method 76-21). The analyses were conducted with 2.5 g of starch samples in about 25 mL of water (corrected based on the moisture content of the starch samples).
The X-ray diffraction patterns and FTIR spectra of the starches were obtained using the previously outlined method (Oyeyinka et al., 2021b). For FTIR measurement, spectra were obtained in the transmittance mode with 64 scans from 400 to 4,000 cm-1. To further assess the impact of USN on the degree of order within the starch granules, the ratios of 1,045/1,022 cm-1 and 1,022/955 cm-1 were compared.
The particle size of FBS granules was measured by laser diffraction analysis (Malvern Mastersizer Hydro 2000, Malvern Panalytical Ltd, Worcestershire, UK). Starch samples were dispersed in distilled water within a dispersion tank, while a stirrer rotating at 2,300 rpm ensured sample homogeneity. Measurements were performed in triplicate for each starch sample, and the mean particle sizes at the 10th, 50th, and 90th percentiles of the particle size distribution were recorded.
All experiments were performed in duplicate, while analyses were conducted in triplicate. Data were analyzed using analysis of variance (ANOVA) and expressed as mean±standard deviation. A one-way ANOVA and Fisher’s Least Significant Difference (LSD) test were conducted using SPSS Version 21.0 (SPSS Inc., Chicago, IL, USA), with statistical significance set at p<0.05.
3. Results and discussion
All the starches (native and modified) generally had a very high L* value (>93), suggesting high starch purity (Table 1). USN did not result in a significant (p>0.05) change in the lightness (L*) values of FBS. Babu et al. (2019) also reported an insignificant increase in the L* value of foxtail millet starch. However, Amarnath et al. (2023) found a significant decrease in the L* value of white finger millet starch subjected to USN. Variation in L* values may be due to the source of the starch as well as the duration used in the respective study. In this study, FBS was ultrasonicated for varying times (5-60 min), while finger millet and foxtail millet starches used in earlier studies are cereals. The endogenous lipids present in most cereal starches can significantly contribute to the variations in their color.
In terms of amylose content, modified FBS generally showed higher levels than the native starch (Table 1). Increasing USN time up to 30 min significantly increased the amylose content of FBS, but beyond 30 min, there was a slight decrease. The initial increase in amylose content following USN may be due to linearisation or depolymerization of the branched component of some amylopectin fraction, which presumably increased the ratio of amylose to the amylopectin in the modified starch. The subsequent decrease in amylose content with extended USN time might be due to the impact on amorphous regions, which are predominantly composed of amylose chains. Overall, the amylose content of native and modified FBS is within the reported amylose content of legume starches, including FBS (30.61%-33.55%) (Punia et al., 2019; Zhang et al., 2019).
The native FBS showed typical shapes characterized by spherical, oval, kidney, and elliptical shapes commonly found in legume starches (Fig. 1A) with varying size ranges between 15.28 and 45.09 μm (Table 2). These values fall within the range (8 and 48 μm) previously reported for FBS (Ambigaipalan et al., 2011; Sofi et al., 2013). The modification process significantly changed the morphology (shape and size) of the starch granules (Fig. 1B-1L and Table 2). These changes in granule morphology were pronounced with increasing USN time (Fig. 1B-1F). FBS subjected to USN showed varying levels of distortions, such as cracked regions (Fig. 1C and 1D), agglomeration, and open pores within the granules (Fig. 1F). Alterations in starch morphology have been linked to the high shear forces and pressure gradients produced during USN (Yang et al., 2019). Wang et al. (2020a) also found visible cracks and pores on the surface of sweet potato starch after USN. These authors reported that the extent of granule distortion increased with longer USN durations. In this study, USN above 15 min generally resulted in large variation in particle size of the starch granules, suggesting that the treatment increased the surface area of the granules for trapping the ultrasound waves. Earlier studies also reported an increase in particle size caused by swelling after USN (Wei et al., 2023; Xiao et al., 2021).
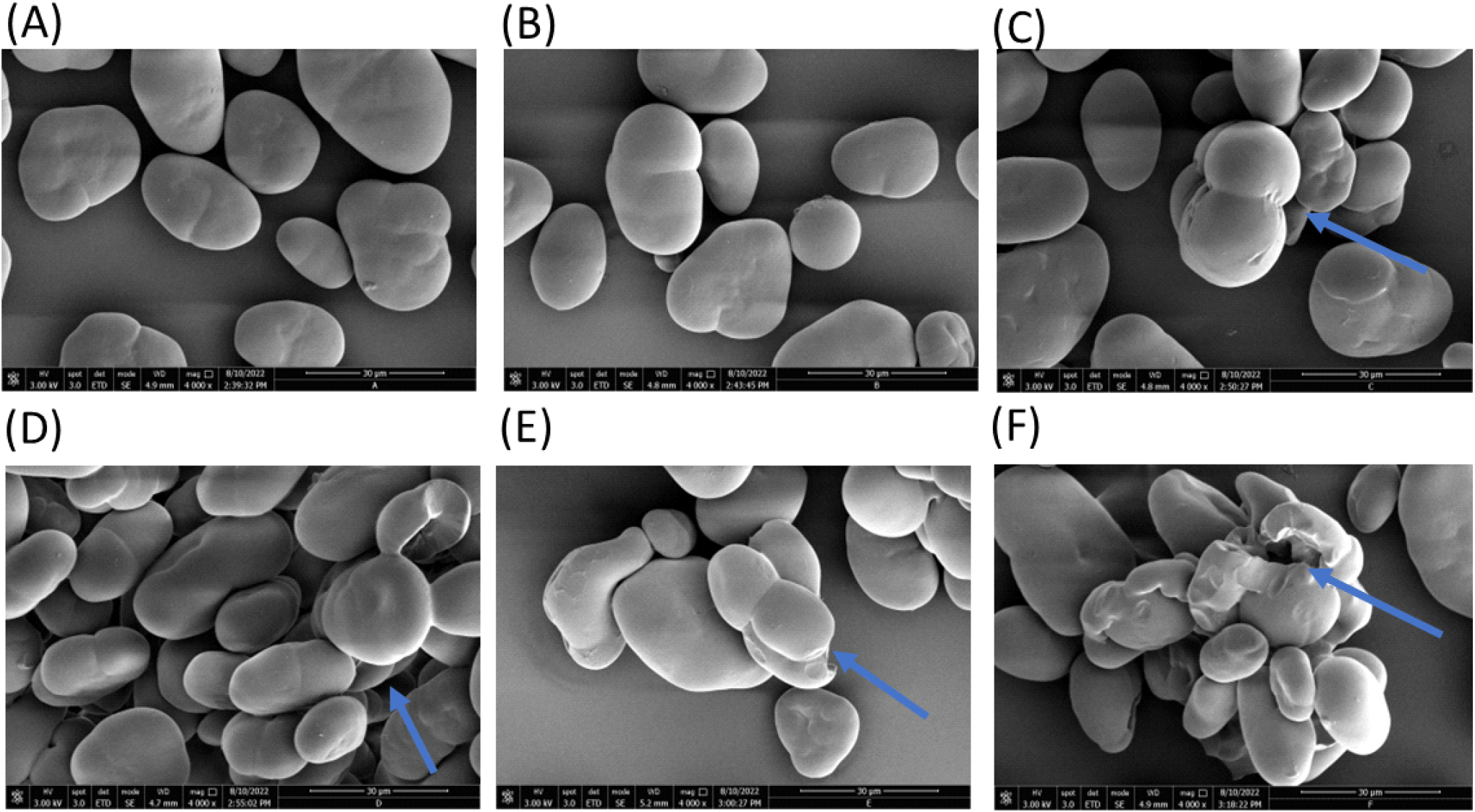
Water absorption capacity (WAC), swelling power and syneresis are key in determining the application of starch in the food industry. FBS subjected to USN generally showed a significantly higher ability to absorb water compared to the native starch, except for starch treated for 5 min (Table 2). The increase in WAC after USN could be associated with the increase in the size of the starch granules (Table 2). This seems plausible since the granule size showed a significant increase after USN for 5 min (Table 2). Previous research suggested that starch granules with a larger surface area may have a higher capacity to absorb water. An increase in WAC after USN has also been reported in corn, potato, rice, and wheat starches (Sujka and Jamroz, 2013).
The native and modified starches showed a temperature-dependent increase in swelling power (Table 3). Starches showed a substantial increase in swelling between 70 and 90°C, suggesting gelatinization of the starches, resulting from the weakening of hydrogen bonds in the crystalline regions. Gelatinization temperatures reported for FBS range between 64.2 and 68.4°C (Sharma et al., 2020; Zhang et al., 2019).
The USN treatment had varied impacts on the starch, especially at different test temperatures. It was observed that USN generally increased the swelling power of the starch granules at lower temperatures (50 and 60°C) but had no significant effect at higher temperatures (70-90°C). The reason for this is not very clear. However, Falsafi et al. (2019) noted a similar trend in ultrasonicated oat starch and suggested that the increase at lower temperatures is due to minimal breakage of hydrogen bonds by USN. This presumably expedited the loosening of the starch network by degrading the crystalline regions. Therefore, longer USN times seem to cause greater damage to the crystalline region, which may account for the lower swelling power of the starches. This is consistent with the pasting viscosity results, which will be discussed later (Table 4). Modified starches generally showed lower syneresis compared to the native FBS (Table 2). Nevertheless, the amount of water released on day 0 was not significant regardless of the USN time. USN treatment for 30-60 min seemed to have a greater effect on starch syneresis during the storage period compared to samples treated for shorter times (5 and 15 min). Additionally, it was observed that storage for 2 and 6 days resulted in a lower syneresis rate compared to both native and treated samples on day 0. Syneresis in starch is associated with the degree of retrogradation, presumably due to the reassociation of starch molecules (amylose and amylopectin). Thus, the initial reduction in the syneresis rate indicates that USN tends to reduce the rate of retrogradation in starch, depending on the extent of reassociation and possibly the molecular weight and chain length of the starch components (Charoenrein et al., 2008; Falsafi et al., 2019). Differences have been noted in how USN affects the rate of starch syneresis. For instance, some researchers observed a reduction (non-significant) in the syneresis of lentil starch gel that was sonicated (Majeed et al., 2017), while other authors found a significant increase (Falsafi et al., 2019; Hu et al., 2014). The reduced syneresis may explain the reduction in the setback viscosity (Table 3). Further details on pasting properties will be discussed in the subsequent section.
The pasting profile curves for native and modified starch are similar (Fig. 2), while Table 4 summarizes the pasting properties. The results indicate a progressive decrease in the peak, breakdown, final, and setback viscosities of FBS with increasing USN time. In contrast, both pasting temperature and peak time showed an upward trend. Literature reveals variability in the effects of USN on the pasting viscosity of different starches. For example, USN has been shown to reduce the peak viscosity of mung bean starch (Shen et al., 2021) and waxy rice starch (Zuo et al., 2009), while it has been found to increase the peak viscosity of chestnut starch (Wang et al., 2020b) and oat starch (Falsafi et al., 2019).
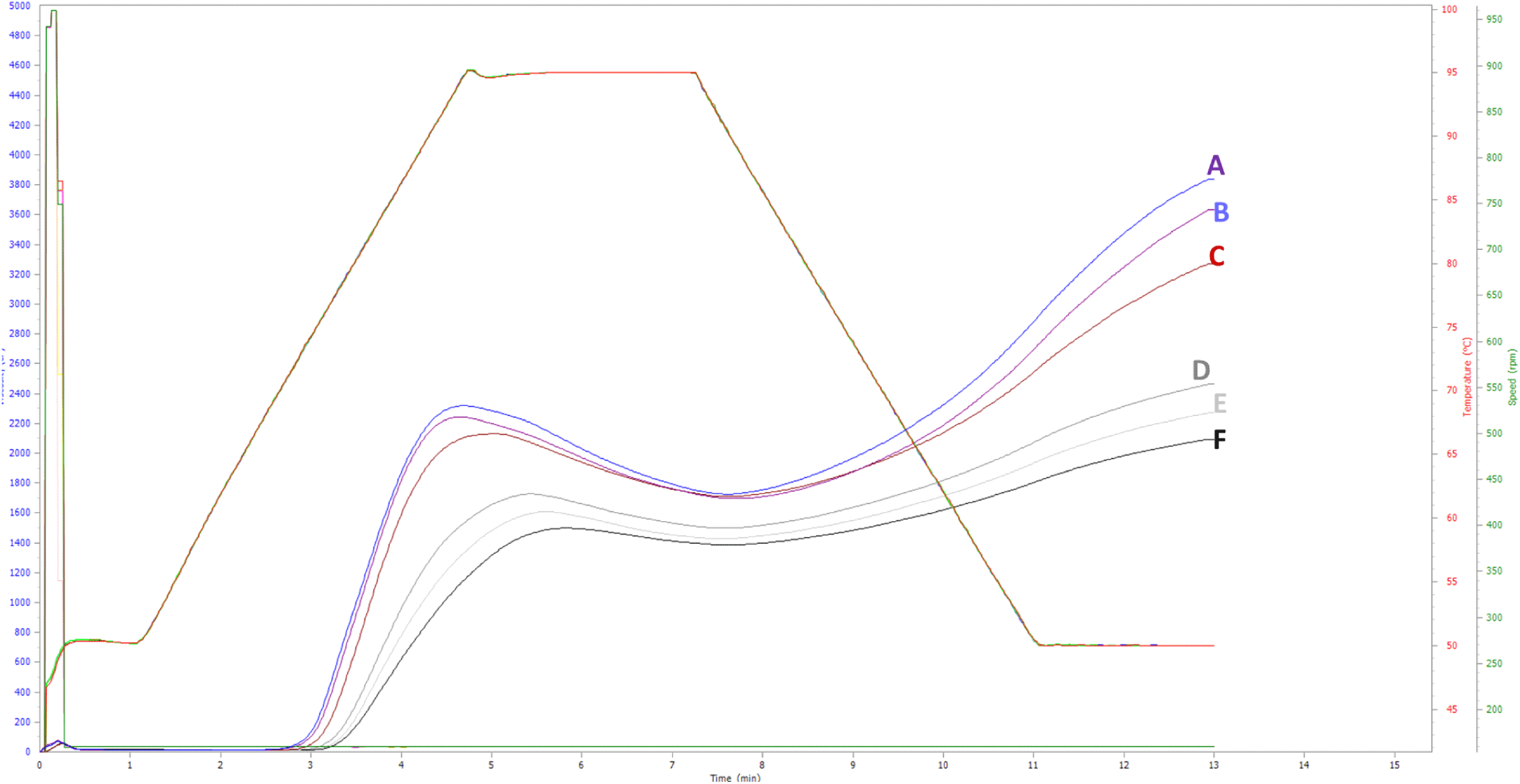
The observed decrease in peak viscosity of FBS aligns with reductions in swelling power (Table 3), increases in amylose content (Table 1), and alterations in granule morphology (Fig. 1). Amylose can create a barrier around starch granules, limiting their swelling capacity during the pasting process (Sang et al., 2008). Peak viscosity, which represents the maximum swelling of starch granules during heating and shearing, is influenced by various factors, including starch composition, structure, granule size, and the presence of non-starch components such as lipids. Starches rich in long-chain amylopectin tend to exhibit greater swelling (Jane et al., 1999).
It is hypothesized that USN leads to a reduction in the molecular weight of amylopectin, thereby affecting the starch’s ability to absorb water. Wei et al. (2021) investigated the degradation mechanisms of amylopectin under USN treatment, revealing that it damaged the amylopectin component of starch and disrupted α-1,6-glycosidic bonds. These alterations, which are likely to intensify with prolonged USN exposure, may account for the significant decrease in peak viscosity. Moreover, this degradation produces smaller molecules, which typically exhibit lower viscosity compared to unmodified starch (Majzoobi et al., 2015).
A marked reduction in setback viscosity was also observed in FBS following USN treatment (Table 4), suggesting a diminished tendency for retrogradation, which is associated with lower syneresis, as seen in Table 2. Retrogradation occurs when disaggregated amylose and amylopectin re-associate to form more ordered structures, with setback viscosity serving as an indicator of this process (Wang et al., 2015). Majzoobi et al. (2015) similarly reported a significant decrease in the setback viscosity of wheat starch after sonication.
As previously mentioned, the pasting temperature of FBS increased post-USN treatment. This change may be attributed to modifications in the amorphous and crystalline structures of the starch (Majzoobi et al., 2015). Jafari and Koocheki (2023) suggested that the increase in pasting temperature following USN is due to partial hydrolysis of the amorphous regions, resulting in a higher crystalline-to-amorphous ratio. The elevated pasting temperature for ultrasonicated starch indicates greater resistance to rupture during cooking, confirming enhanced stability associated with USN due to a reduction in the breakdown viscosity of the starches (Table 4). One limitation of native starch is its inadequate resistance to shear; thus, USN presents a promising method for improving the stability of starches under shear and thermal treatments.
Structural characterization of the native and modified FBS was assessed using FTIR and XRD techniques. Native and modified FBS showed strong peaks at 15° (2θ), 17° (2θ), and a single peak at 23° (2θ) (Fig. 3). Pulse starches generally exhibit the C-type crystallinity pattern, which may be further classified into CA-type (closer to A-type crystallinity) or CB-type (closer to B-type crystallinity) (Cai et al., 2014). The absence of a doublet in the XRD pattern of the FBS suggests a CB-type crystallinity. USN did not change the crystalline pattern (Fig. 3) and secondary structure of FBS, as revealed in Fig. 4. However, there seems to be a significant impact on the crystalline and amorphous regions, as reflected in the FTIR data (Table 1). The FTIR spectra showed typical spectra for starch molecules with sharp peaks within the broad band region of 3,000-3,650 cm-1 which may be attributed to the stretching of free, inter-and intra-molecular hydroxyl (-OH) groups (Guo et al., 2019). Furthermore, the sharp peak at 2,931 cm-1 in the native and modified starches is mainly due to the C-H stretching associated with the ring methine hydrogen atoms (Guo et al., 2019), while the peak in the region of 1,650 cm-1 could be due to the bending vibration of bound water in the amorphous region of starch (Kizil et al., 2002; Zeng et al., 2011).
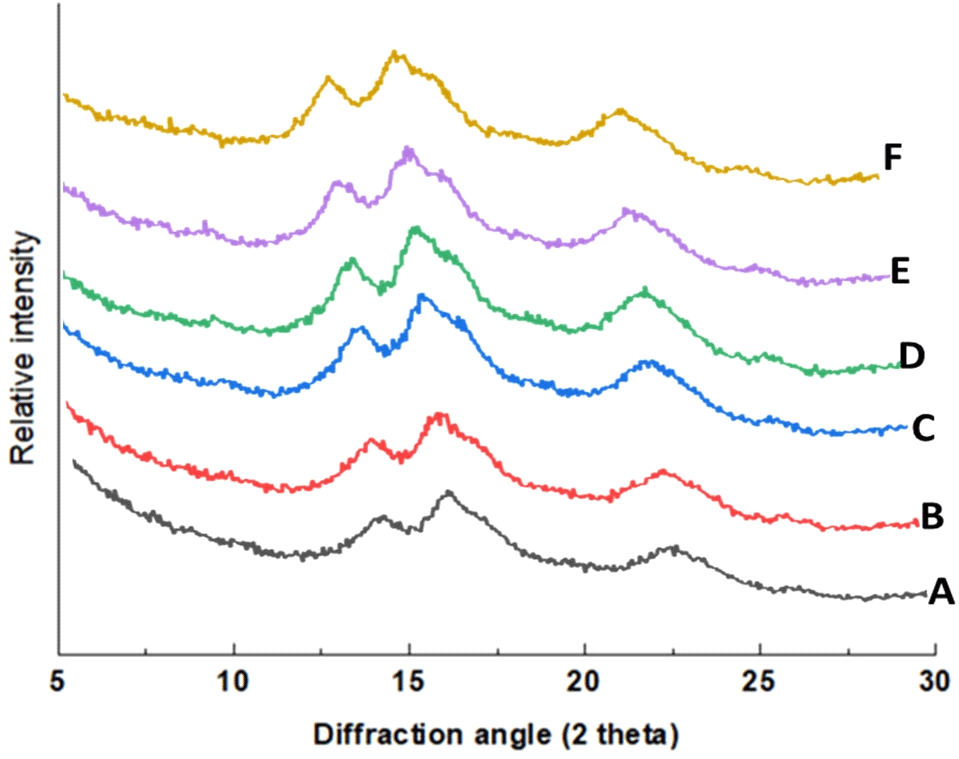
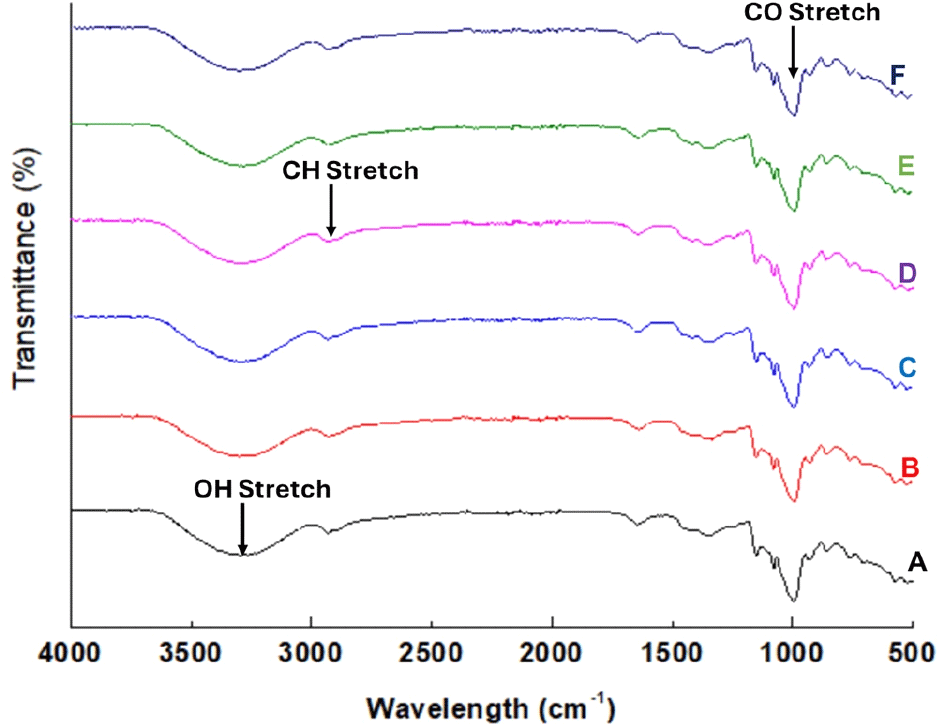
To evaluate the impact of USN on the amorphous and crystalline structures of FBS, the ratio of the bands at 1,022 and 1,045 cm-1 corresponding to the amorphous and crystalline regions in starch, respectively, was determined (Table 1). This band ratio (1,045/1,022 cm-1) serves as an estimate of the degree of crystalline order in starch (Babu et al., 2019). The ratio of these peaks gradually reduced with USN time up to 15 min, increased at 30 min, and decreased thereafter. The initial decrease in the ratio of these bands coincides with an initial increase in amylose content which also followed the same trend of increase and further decrease. The ratio of 995/1,022 cm-1, which is linked with the proportion of the amorphous region to an ordered fraction in starch, also followed the same trend with the ratio of the bands at 1,045 and 1,022 cm-1. This suggests that USN produced starch with fewer shorter side chains compared with the native starch. Further heating up to 30 min possibly caused a rearrangement leading to an increase in the ratio of these bands. Further research is necessary to comprehend the effect of USN within this time range on starches from different botanical origins to fully elucidate the mechanisms controlling changes in starch structure. Based on the findings from this study, 30 min is likely the optimal treatment time for FBS to produce starch with desirable functionality.
4. Conclusions
In the present study, FBS was modified at different USN time. Significant differences in the structure and functional properties of the modified starches were observed with increasing USN time. USN initially increased the amylose content and thereafter reduced the level of FBS. Scanning electron micrographs showed distortions in the starch granules, with granules becoming bigger with increasing USN time. The color, FTIR spectra, and XRD pattern of the starch were not affected by USN. Still, the crystalline and amorphous regions were impacted, resulting in changes in functional and pasting properties. FBS showed reduced breakdown and setback viscosities, suggesting improvement in shear and thermal stability and, hence, increased suitability in the food industries. Future research should evaluate the applicability of the modified starch in different food applications.