1. Introduction
Fruits and vegetables contain essential minerals such as calcium, potassium, magnesium, and iron, as well as antioxidant vitamins like β-carotene. Additionally, they are rich in functional plant compounds phytochemicals, which contribute to their health promoting properties. These functional compounds play a role in the prevention and treatment of chronic diseases, highlighting the importance of consuming fruits and vegetables (Suh et al., 2009). In particular, the outbreak of COVID-19 there has been a shift in consumer behavior with an increasing demand for health-conscious choices and convenience which has also led to a diversification of fruit consumption patterns (Fan and Kim, 2023). It is known that dried products processed by removing only the moisture from fresh fruits and vegetables are protected from spoilage and deterioration caused by microorganisms and enzymes (Jung et al., 2012). Vegetable drying extends shelf life, preventing losses caused by overproduction and inadequate storage facilities. Dried vegetables are convenient for consumption and can be developed into various product forms. Additionally, since they maintain the nutritional superiority of the vegetables, the drying of vegetables is increasing (Jeong et al., 2021).
Lotus root (Nelumbo nucifera G.) is a perennial aquatic plant belonging to the water lily family. It is an edible bulb with large stems and has been widely used as food in raw, fried, and stewed ways since ancient times (Bae et al., 2008). Lotus root is known to possess various health benefits, including the management of hypertension, diabetes, stress reduction, anticancer effects, kidney protection, and antioxidant properties (Hwang and Park, 2024). As the useful functionality of lotus root becomes known, its consumption has been gradually increasing recently. The price of lotus root tends to decrease from September to November, which is the harvest season. Additionally, lotus root is highly sensitive to low temperatures after harvest, and cold damage below a certain threshold temperature can significantly affect its quality and storability, thereby impacting its marketability. Therefore, research on long-term storage and processing methods for lotus root is necessary (Kwon et al., 2010).
Drying is the process of removing moisture from foods with high water content. During the drying process, the growth of microorganisms and the activity of enzymes that cause spoilage or deterioration are inhibited, which enhances the shelf life and transportability of the product, making it suitable for new food product development. The most common drying methods for foods include sun drying and hot air drying. In recent years, due to the activation of dried products, various drying techniques have been introduced, including freeze-drying, vacuum drying, microwave drying, and far infrared drying (Hwang and Kim, 2024). Sun drying requires minimal investment and is free from the harmful effects of chemicals. However, it has the disadvantage of producing inconsistent product quality depending on weather conditions (Park et al., 2018). Hot air drying, while fast, simple, and cost effective, can lead to issues such as shrinkage due to moisture loss, surface hardening, low rehydration capacity, and browning reactions. These factors contribute to problems such as reduced color, texture, flavor, and nutritional value of the dried products (Jegal and Chung, 2023). Freeze-drying is a method that involves freezing the product below its freezing point and then sublimating the ice without the movement of moisture. This technique causes minimal changes in the texture, aroma, and composition of the dried product, and it allows for rapid rehydration of the dried food, making it widely used. However, it has the disadvantages of long drying times and high costs (Choi et al., 2015). On the other hand, cold air drying is a process that involves drying at low temperatures. It minimizes component degradation due to heat, is not affected by climatic conditions, allows for precise control over final moisture content, and offers cost-saving advantages (Hong et al., 2006). Vacuum drying involves a process where the oxygen concentration is very low, preventing spoilage, deterioration, and browning of fruits during drying (Park et al., 2017). As a result, cold vacuum drying, which combines the principles of cold air drying with vacuum functionality, allows for the drying of fruits and vegetables at relatively low temperatures. Since the process occurs at lower temperatures, it helps suppress heat-induced degradation of components and browning, while also reducing drying time. Therefore, cold vacuum drying is considered one of the suitable methods for fruit drying.
Therefore, this study aimed to provide fundamental data on vegetable drying by comparing the quality of lotus root dried using freeze-drying and cold vacuum drying methods.
2. Materials and methods
The lotus root used in the experiment were purchased from a Hayang market in Gyeongbuk and were cleaned and prepared. Before osmotic dehydration, the lotus roots were sliced into 4 mm thick pieces. The solutes used in the osmotic dehydration process included sucrose (Baeksul, Hayan sucrose, Seoul, Korea), vitamin C (Kanto Chemical Co., Tokyo, Japan), and calcium (KMF, Natural fermented water-soluble calcium, Daegu, Korea).
Osmotic treatment conditions were determined following the method of Jung (2013). To evaluate the quality characteristics according to osmotic concentration, the sliced lotus roots were immersed in solutions with varying concentrations of sucrose (0, 10, 20, and 30%) and supplemented with 0.1% vitamin C and 0.5% calcium. The immersion was carried out at 20°C for 20 min with a 1:5 (w/v) ratio. After immersion, the samples were dried by cold vacuum drying (drying temperature: 35°C), and their quality characteristics were measured.
The osmotic-treated lotus roots were dried using a vacuum cold dryer (Kumkang Tech Co., Daegu, Korea) with a cold air temperature of 35°C. The drying process was conducted with periodic pressure cycling, alternating between 2 min of pressure and 8 min of vacuum, for a total duration of 15 h. As a control, freeze-drying was performed at -45°C using a freeze dryer (FD SFDSM12, Samwon, Seoul, Korea) until a constant moisture content was achieved.
Water activity was measured using a water activity meter (RS 232, Novasina, Lachen, Switzerland) by placing 0.3 g of dried lotus root on a circular measurement disk.
Moisture content was measured using an infrared moisture analyzer (HG53, Mettler Toledo, Columbus, Ohio, USA) by drying the sample at 105°C until no further weight loss occurred. Soluble solid content was determined by adding 40 mL of distilled water to 4 g of the sample, followed by homogenization using a homogenizer (AM-11, Nihonseiki Kaisha Ltd., Tokyo, Japan) at 10,000 rpm for 5 min. The mixture was then filtered, and soluble solids were measured using a digital refractometer (GMF-703F, G-WON, Seoul, Korea).
pH was measured by adding 40 mL of distilled water to 4 g of the sample, followed by homogenization using a homogenizer (AM-11, Nihonseiki Kaisha Ltd.) at 10,000 rpm for 5 min. The mixture was then filtered, and the pH was determined using a pH meter (HS53, Toledo GmbH, Greifensee, Switzerland). Titratable acidity was determined by titrating with 0.01 N NaOH to pH 8.3, and the amount of NaOH consumed was converted to citric acid percentage.
The browning degree was measured by adding 40 mL of distilled water to 2 g of dried lotus root and adding 10 mL of 10% trichloroacetic acid, leaving it at room temperature for 2 h, then filtering, and measuring the absorbance at 760 nm using a spectrophotometer (UV1601, Shimadzu., Kyoto, Japan).
Color measurements were performed using a colorimeter (CR-200, Minolta Co., Tokyo, Japan). The Hunter color value of L* (lightness), a* (redness), and b* (yellowness) were used to represent the color attributes. The values for the white standard were set as L*=94.5, a*=0.3132, and b*= 0.3203.
To measure strength and hardness, texture was measured using a rheometer (Compac-100, Sun Scientific Co., Tokyo, Japan) equipped with a 15 mm diameter probe. The measurement conditions were set with sample depth and width at 3 mm and 50 mm, respectively. Instrument parameters were set as follows sample movement of 10 mm, table speed of 60 mm/min, and plunger diameter of 5 mm. The measurements were repeated three times.
Ascorbic acid content was determined by first diluting 2 mL of the sample with 5% metaphosphoric acid solution to a 20-fold dilution. Then, 2 mL of the diluted sample was mixed with 1-4 drops of 0.2% 2,6-dichlorophenol indophenol (Sigma-Aldrich Co., St. Louis, MO, USA) solution until the solution turned purple. After this, 2 mL of 1% SnCl2 ․ 2H2O solution and 1 mL of 2,4-dinitrophenylhydrazine solution (Kanto Chemical Co.,) were added, and the mixture was incubated at 50°C for 1 h. The reaction mixture was cooled, and 5 mL of 85% sulfuric acid was slowly added. After about 30 min of color development at room temperature, absorbance was measured at 540 nm, using a blank prepared by adding 2,4-dinitrophenylhydrazine solution after the sulfuric acid addition. A standard solution of L-ascorbic acid (Kanto Chemical Co.,) was prepared by diluting with 5% metaphosphoric acid, and a standard curve was constructed to calculate the ascorbic acid content in the sample.
The total polyphenol content was determined following the method of Dewanto et al. (2002). A 5 g sample was extracted with 50 mL of 70% ethanol and homogenized for 2 min. The extract was filtered, and 100 μL of the filtrate was mixed with 2 mL of 2% sodium carbonate and 100 μL of 50% Folin-Ciocalteu reagent. The absorbance was measured at 720 nm, and the polyphenol content was calculated based on a standard curve prepared using gallic acid (Sigma-Aldrich Co.).
The total flavonoid content was determined following the method of Abdel-Hameed (2008). One mL of sample was mixed with 0.15 mL of 5% sodium nitrite and vortexed, then left to stand at 25°C for 6 min. Next, 0.3 mL of 10% aluminium chloride was added, and the mixture was vortexed and left at 25°C for 5 min. Afterward, 1 mL of 1 N NaOH was added, and the absorbance was measured at 510 nm. The flavonoid content was calculated based on a standard curve prepared using rutin hydrate (Sigma-Aldrich Co.).
All experiments were performed in triplicate, and results were expressed as mean±standard deviation (SD). Statistical analyses were conducted using SPSS Statistics 12 (SPSS Inc., Chicago, IL, USA). Differences among groups were evaluated using followed by Duncan’s multiple range test and a t-test was conducted for comparison. Statistical significance was considered at p<0.05.
3. Results and discussion
To determine the optimal drying time for lotus root, slices of 4 mm thickness were dried using a cold vacuum dryer, and changes in moisture content were measured during the drying process. At 12 h of drying, the moisture content was 3.69%, and at 15 h, it was 2.0% (data not shown). For the lotus root dried for 15 h, the drying process was more advanced, resulting in a higher surface strength of the dried product. Based on preliminary experiments, the optimal drying time for cold vacuum dried lotus root was determined to be 12 h, with a moisture content of 3.50±0.50% (data not shown).
The results of moisture content, water activity, sugar content, titratable acidity, and pH of lotus root pretreated with osmotic treatment followed by cold vacuum drying are shown in Table 1. Regarding moisture content, osmotic treatment resulted in lower moisture content compared to the control. Even without the addition of sugar, the treatment with ascorbic acid and calcium alone resulted in a lower moisture content, confirming the effectiveness of osmotic treatment. Water activity was also lower in the osmotic-treated samples compared to the control, indicating the osmotic effect. Sugar content increased with higher concentrations of osmotic sugar solutions, while titratable acidity was higher in the osmotic-treated samples due to the use of ascorbic acid. A significant difference in soluble solids was observed in the 30% sugar concentration osmotic solution, while no significant differences were observed in other quality characteristics based on sugar concentration. In the case of osmotic-treated pumpkin, the quality characteristics showed a similar trend to previous studies, where an increase in the sugar concentration of the osmotic solution led to a decrease in moisture content (Hong and Lee, 2004). Also, in research utilizing Hardy kiwi, it was also observed that as the sugar concentration of the osmotic treatment solution increased, the moisture content decreased, while the solid content tended to increase (Choi et al., 2021).
As for changes according to sugar concentration, a significant difference was observed at 30% concentration, so the sugar concentration was set at 30%. After soaking the lotus roots for 20 min, freeze-drying and cold vacuum drying were performed. Quality characteristics according to drying method are shown in Table 2. The moisture content of the freeze-drying lotus root was approximately 1.5% higher. However, the water activity was lower. The ascorbic acid content, soluble solids, and titratable acidity of the dried lotus root were higher in the freeze-drying compared to those dried by cold vacuum drying, indicating that osmotic treatment had a more pronounced effect with freeze-drying. However, no significant differences were observed in pH and browning between the drying methods. The results of the significance test showed that there were significant differences between drying methods for moisture content, titratable acidity, and ascorbic acid content, while no significant differences were found for other quality characteristics. Youn and Hong (1999) reported, it has been reported that higher sugar concentrations minimize the loss of ascorbic acid during drying due to the protective effect of sugar. Additionally, higher sugar concentrations lead to rapid diffusion of moisture during drying, leading to a decrease in moisture content, which is like the observed results.
The results of color measurements for the drying lotus root are presented in Table 3. Compared to freeze-drying, cold vacuum drying showed a lower L* value and higher a* and b* values, indicating that some color changes occurred during the drying process. The significance test results also showed that there was a significant difference depending on the drying method, and the freeze-drying lotus root maintained a brighter color (p<0.001). Kwon et al. (2013) reported as drying progresses the L* value generally decreases. This showed similar results to a study in which the L* value decreased as definition increased while maintaining the chromaticity of lotus root, rather than a decrease in brightness due to quality deterioration. Kim et al. (2013) reported that palatability may decrease due to browning during the drying process, but it is believed that added value can be increased if a method appropriate for the characteristics of the product is used.
The texture of the lotus root was measured and the results are shown in Table 4. The hardness and strength of lotus root dried under cold vacuum were found to be higher than that of lotus root freeze-drying. It was found to have stronger physical properties upon drying. Significance tests also showed significant differences between drying methods (p<0.001). Young and Wo (1999) reported that during the drying process, heat increases moisture diffusion and at the same time, moisture evaporates from the surface into the air. When the moisture content of the food drops below 40%, if the drying speed is too fast or the heat is too high, surface hardening tends to occur, leading to an increase in hardness. Excessive surface hardening can negatively affect the texture and cause excessive external collapse, resulting in nutritional loss during the drying process. Freeze-drying is superior in quality compared to cold vacuum drying. However, considering the texture, cold vacuum drying may be applicable depending on the characteristics of the dried vegetables.
Hardness (kg/cm2) |
Strength (kg/cm2) |
|
---|---|---|
Freeze drying | 0.18±0.021) | 1.75±0.65 |
Cold vacuum drying | 5.31±0.84***2) | 314.6±130.63*** |
The measurement results of total polyphenol content and total flavonoid content of dried lotus root are shown in Table 5. The total polyphenol content was 4.94 mg/mL for freeze-drying and 4.33 mg/mL or cold vacuum drying, with freeze-drying showing higher content than cold vacuum drying. Similarly, the flavonoid content was 0.60 mg/mL for freeze-drying and 0.18 mg/mL for cold vacuum drying, with freeze-drying again exhibiting a higher concentration. The results of the significance test also showed differences between the drying methods. Flavonoids are recognized for their antioxidant properties, as well as their ability to prevent cardiovascular diseases, reduce inflammation, alleviate allergies, exhibit antimicrobial and antiviral effects, lower lipids, enhance immunity, and strengthen capillaries. It has been reported that the content of flavonoids varies depending on the drying and pretreatment methods (Jeong and Youn, 2016). In terms of total polyphenol and flavonoid content, freeze-drying showed relatively higher levels compared to cold vacuum drying. Hwang and Nhuan (2014) reported that polyphenolic compounds exhibited the highest content in freeze-drying. Also, Kim et al. (2018) reported polyphenol compounds in fruits and vegetables are known to oxidize into quinones or their derivatives through reactions with oxygen and polyphenol oxidase, which are factors contributing to discoloration and quality deterioration in food. Therefore, it is believed that this difference arises due to the higher drying temperature and exposure to oxygen in cold vacuum drying compared to freeze-drying.
Total polyphenol (mg/g) | Total flavonid (mg/g) | |
---|---|---|
Freeze drying | 49.4±1.01) | 6.0±0.1 |
Cold vacuum drying | 43.3±0.85***2) | 1.8±0.1*** |
Fig. 1 shows the appearance of lotus root dried by freeze-drying and cold vacuum drying. The freeze-drying treatment had a positive effect on maintaining both shape and color, whereas, although osmotic treatment was applied in the cold vacuum drying process, some changes were observed compared to freeze-drying.
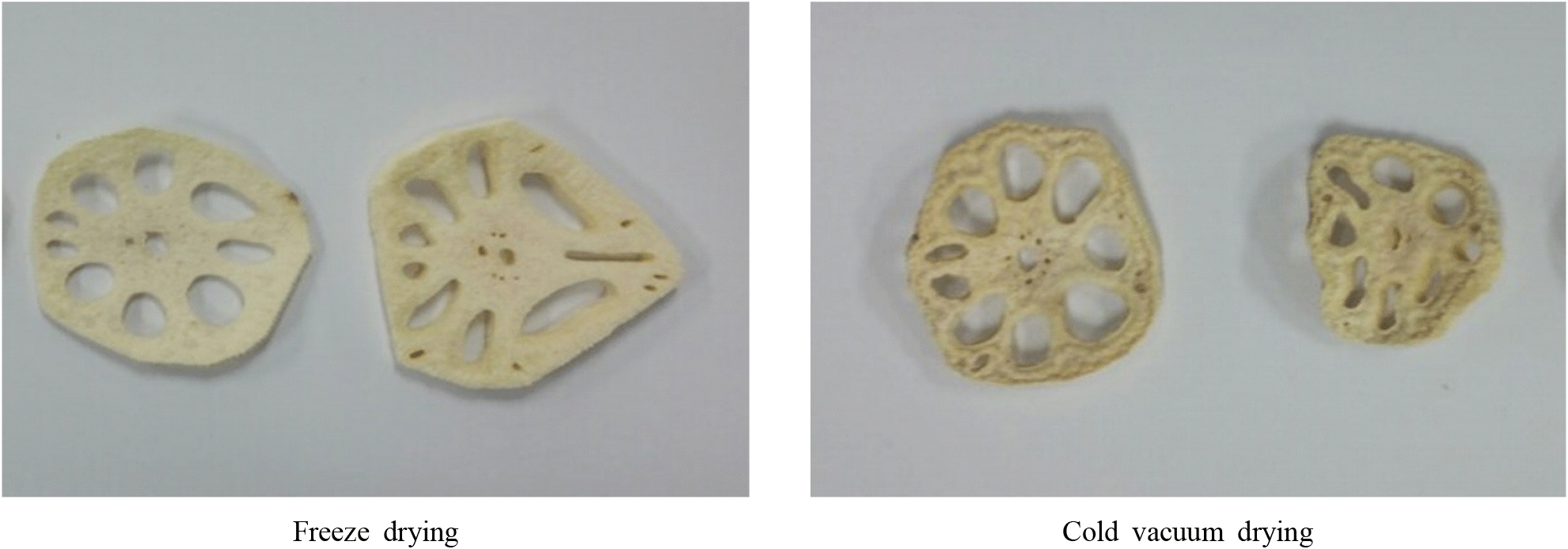
4. Conclusions
To determine the optimal drying time for dried lotus root chips, lotus root slices 4 mm thick were dried using a cold vacuum dryer, and moisture content was measured. The optimal drying time was found to be 12 h, with a moisture content of 3.50±0.50%. Lotus root slices immersed in a 30% osmotic solution were subjected to both freeze-drying and cold vacuum drying. The results showed that the moisture content of the freeze-drying lotus root was approximately 1.5% higher than that of the cold vacuum drying, while the water activity was lower in the freeze-drying. There were no significant differences between drying methods in terms of titratable acidity, pH, and browning degree. In terms of color, the cold vacuum drying process resulted in a lower L* value and higher values for both a* and b* compared to freeze-drying, indicating that browning occurred during the drying process in the cold vacuum drying. Total polyphenol content was 4.94 mg/mL for freeze-drying and 4.33 mg/mL for cold vacuum drying, which showed a higher content than cold vacuum drying. Similarly, total flavonoid content was 0.60 mg/mL in the freeze-drying 0.18 mg/mL in the cold vacuum drying, indicating that the freeze-drying had a higher content. As a result of the above findings, it was shown that freeze-drying is superior to cold vacuum drying for lotus roots drying.