Introduction
Soybean (Glycine max L.) is widely cultivated in the world. Soybeans are used in a variety of foods, such as soybean pastes, soybean sauces, soybean curds, soybean sprouts, and soy yogurts. Soybean sprouts have been traditionally consumed in oriental cultures as a vegetable (1-3). The germination of soybean can be an alternative means to enhance the nutritional qualities of targeted phytochemical components (2). The sprouting of soybeans is expected to be an economical and effective technology that hydrolyzes macromolecules, improving digestibility and increasing its nutritive value (4). In particular, the soybean sprouts can increase the γ-aminobutyric acid (5-7) and isoflavone contents (8) and antioxidant effects (9).
Gamma-aminobutyric acid (GABA) is widely found in eukaryotic and prokaryotic organisms. GABA is a non-protein amino acid that is produced mainly by the decarboxylation of glutamic acid catalyzed by the enzyme glutamate decarboxylase (GAD) (10) and under the presence of coenzyme pyridoxal 5´-phosphate (PLP) and converted to succinate semialdehyde by GABA transaminase. GABA is an important inhibitory neurotransmitter in the central nervous system of mammals, triggering a series of metabolic functions including tranquilizing and allaying excitement, decreasing blood pressure (11,12) and cholesterol, and improving human health (6). However, its content in animals is very low. GABA production can be increased in the soybean by stimulating the activity of GAD through various environmental stresses (7,13). Soaking, sprouting and lactic acid fermentation have been reported to be effective for the production of GABA in cereals such as germinated soybean (14) and Lactobacillus paracasei with fermented foods (15).
Glutamic acid (GA) is used to produce the GABA that is found in most soybeans. GABA synthesis by plants through supplementation with glutamic acid or glutamate has been reported (16,17). However, the yield of GABA accumulated by fruits and vegetables is low. GABA production through fermentation, believed to be convenient and efficient, has been applied in the food industry. Several microorganisms generally recognized as safe including lactic acid bacteria (LAB) such as Lactobacillus brevis (18-20), and Lactobacillus paracasei (15) have been widely studied and applied in GABA production over the last few years. In general, soybean cultivars are 35-40% proteins, including 20% GA. However, the high-protein soybean cultivar (HPS, Glycine max L., cv. Saedanbaek) contains approximately 45% to 48% protein, including 30% GA (3). Because the Saedanbaek soybean cultivar contains a higher ratio of GA, the production of GABA from the Saedanbaek soybean would be higher.
Researchers have developed various GABA-rich fermented foods by using Lactobacillus sp. (21-23). Recently, GABA-enriched soybean paste without aflatoxin produced by Apergillus oryzae and Lactobacillus brevis was reported (24). Wang et al. (6) reported that the levels of GABA, isoflavone and antioxidant activities were enhanced by the germination of Chinese soybean cultivars. There have also been several reports on GABA in soybean sprouts. Although some studies have been carried out to improve the GABA content in soybeans under germination conditions, few reports have studied the enhanced levels of GABA and isoflavone aglycones in fermented soybean sprouts using L. brevis.
The objective of this study was to investigate the effect of sprouting on the levels of selected health-promotion functional factors (GABA and isoflavone aglycones) in the high-protein soybean (HPS). This paper reports the methods and results for the production of the HPS sprouts with high levels of GABA, free amino acids and isoflavone aglycones.
Materials and Methods
The high-protein soybean (HPS), Saedanbaek cultivar, was collected from the National Institute of Crop Science (Miryang, Korea) in 2013. Lactobacillus brevis KCTC 3320 was collected from the Korean Collection for Type Cultures (Daejeon, Korea). Three isoflavone glycosides and aglycones, namely, daidzin, glycitin and genistein, daidzein, glycitein, and genistein were purchased from Sigma-Aldrich Chemical Co. (St. Louis, MO, USA). Three malonyl and acetyl isoflavone glycosides (malonyldaidzin, malonylglycitin, malonylgenistin, acetyldaidzin, acetylglycitin and acetylgenistin) were obtained from LC laboratories (Woburn, USA). Monosodium glutamate (MSG), glutamic acid (GA), γ -aminobutyric acid, glacial acetic acid, Folin-Cicalteu phenol reagent, 2,2-diphenyl-1-picryl-hydrazyl (DPPH), 2,2-azinobis (3-ethyl-benzothiazoline-6-sulfonic acid) diammonium salt (ABTS), and potassium persulfate were purchased from Sigma-Aldrich Chemical Co. (USA). HPLC-grade H2O, methanol, and acetonitrile were purchased from Fisher Scientific (Fairlawn, NJ, USA). All other reagents were of analytical grade.
The sprouting of the HPS was following partially modified methods of Huang et al. (4). Briefly, whole HPSs were washed and soaked in water at room temperature for 12 h. After soaking, the soybeans were put into a semi-automatic sprouting machine (model HANCELL, Gwangmyeong control electronic, Gwangmyeong, Korea). The HPSs were automatically watered every hour for 8 h, and were sprouted for 0 h (0 cm), 48 h (1 cm), 72 h (2 cm), and 96 h (4 cm) hr in an incubator at 20℃ (Fig. 1). The sprouted HPS samples were steamed for 30 min at 121℃. The steamed HPSs were harvested and stored in a deep freezer at -70℃ until further analysis.
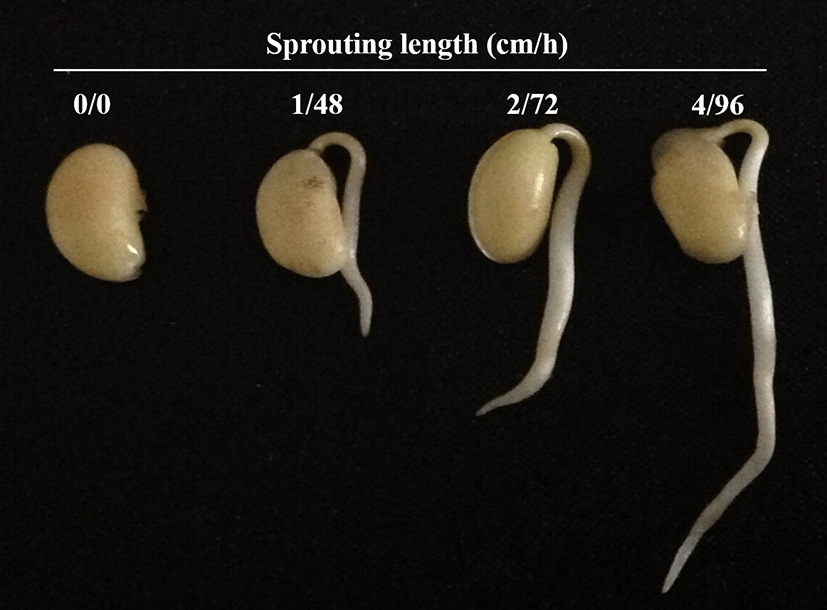
The different processing conditions of HPSs, namely, raw soybean, steamed soybean (0 cm) and sprouting of steamed HPSs (1, 2, and 4 cm), were ground for 3 min. 10 g of soy-powder was mixed with 100 mL of a 2% sucrose solution in different containers. This mixture, namely, unfermented HPS (UFHPS) and unfermented steam-HPSs (UFSHPS), were then sterilized in an autoclave at 121℃ for 15 min. After the seed culture contained approximately 108 CFU/mL with L. brevis, UFHPS and UFSHPSs were fermented at 30±1℃ for 72 h [fermented HPS (FHPS) and fermented stem-HPSs (FSHPSs)], and sampling were carried out at 0 and 72 h. The samples were stored at -70℃ until analysis.
Free amino acids (FAAs) were analyzed according to the method of described by Kim and Ji (24). 1 mL of sample was added to 4 mL of distilled water, and then a heating block (HB-48P, DAIHAN Scientific, Wonju, Korea) was used to drive hydrolysis at 60℃ for 1 h. After 1 mL of 5-sulfosalicylic acid (10%) was added, the mixture was vortexed for 1 min and maintained at 4℃ for 2 h. After centrifuging at 3,000 rpm for 3 min, the supernatant was collected and syringe filtered using a rotary vacuum evaporator at 60℃. The lithium buffer (pH 2.2) was dissolved by applying membrane filtration. The free amino acids content was determined using an amino acid analyzer (Hitachi L-8900, Hitachi, Tokyo, Japan).
The UFHPS, UFSHPSs, FHPS, and FSHPSs samples were dried in freezing dryer (Rikaikai Co., Ltd., Tokyo, Japan) and were ground for 3 min. Each of the ground powders (1 g) was extracted with 10 mL of 50% MeOH by shaking at room temperature for 12 h; the extracts were subjected to membrane filtration (3).
The 0.5 mL of 50% methanol (MeOH) extract was mixed with 0.5 mL of a 25% sodium carbonate (Na2CO3) solution and 0.25 mL of Folin-Cicalteu reagent in a test tube and was kept at 30℃ for 1 h. The absorbance of the mixtures was determined at 750 nm. A gallic acid equivalent (GAE) standard curve was prepared according to the method of Lee et al. (3).
The quantification of isoflavone in 50% MeOH extracts was carried out by a high-performance liquid chromatograph (HPLC, 1200 series, Agilent Co., Forest Hill, Australia) equipped with a diode array detector (DAD). The extracts were separated on a 100 RP C18 column (4.6×250 mm, 5.0 μm, Merck, Darmstadt, Germany) at 30℃. The injection volume was 20 μL for all extracts, and the flow rate was 1.0 mL/min. The following binary mobile phase consisting of (A) 0.2% acetic acid in water and (B) 0.2% acetic acid in acetonitrile was used for the separation of isoflavone: 0 min, 100% A; 15 min, 90% A; 25 min, 80% A; 35 min, 75% A; 45 min, 65% A; 50 min, 65%. All isoflavone peaks were detected and monitored at 254 nm (3).
The DPPH radical scavenging activity of fermented 50% MeOH extracts was performed according to the method described by Hwang et al. (25). Specifically, DPPH solution (1.5×10-4 mM, 0.8 mL) was mixed with the soy-powder milk (SPM) and SPY extracts. After standing at room temperature for 30 min, the absorbance of the mixture was determined at 525 nm using a spectrophotometer (Spectronic 2D, Thermo Co., Petaluma, CA, USA).
The ABTS radical scavenging activity was following the modified methods of Hwang et al. (25). The ABTS stock solution diluted 50 times with sample extract (0.1 mL) was added to 0.9 mL of ABTS•+ solution. After being kept in the dark at room temperature for 3 min, the absorbance was determined at 730 nm using spectrophotometer(Spectronic 2D, Thermo Co.).
Results and Discussion
Comparison of free amino acids (FAAs), glutamic acid (GA) and γ-aminobutyric acid (GABA) contents
In addition to nutrients, certain foods contain a number of substances that do not have a defined nutritional function but may have a significant impact on health. Recent studies have reported the bioactive functions of the non-proteogenic or FAAs, GABA and ornithine. As a highly nutritious plant food material, the soybean (Glycine max L.) has been germinated for human consumption because germination can decrease the level of antinutritional factors (26), while increasing the amounts of some nutrients and phytochemicals, such as amino acids, vitamins, isoflavones, and tocopherols (27). In this study, the individual and total FAAs in the unfermented and fermented HPSs are presented in Table 1. The results differed to some extent for the different lengths of sprouting. The total FAAs were 63.11, 497.98, 813.66, 1,039.72, and 860.32 mg/100 mL in the unfermented HPS (UFHPS) and unfermented HPS with 0 cm (UFSHPS-0), 1 cm (UFSHPS-1), 2 cm (UFSHPS-2), and 4 cm sprouting length (UFSHPS-4) and 79.53, 489.93, 877.55, 780.53, and 979.97 mg/100 mL in the fermented HPS (FHPS) and fermented HPS with 0 cm (FSHPS-0), 1 cm (FSHPS-1), 2 cm (FSHPS-2), and 4 cm sprouting length (FSHPS-4), respectively. The GA concentrations increased from the unfermented raw HPS (UFHPS) to the soybean sprouts of 0 to 1 cm length (UFSHPS-1), and after that, it gradually decreased up to UFSHPS-4. The alanine, aspartic acid, aminoadipic acid, GABA, glycine, isoleucine, lysine, leucine, methionine, serine, threonine, and ornithine concentrations similarly increased from the unfermented raw to fermented sprouting HPS sprouts of 0 to 2 cm length and decreased for the fermented HPS sprouts of 4 cm length. However, the β-alanine, cysteine, citrulline, and valine concentrations gradually increased from the raw to fermented sprouting HPS sprouts of 4 cm in length. Importantly, the arginine concentrations non-linearly increased in the unfermented HPS with varied sprouting lengths, but it was not detected in the fermented HPSs. Conversely, ornithine concentrations were low in the unfermented HPSs but greatly enhanced in the fermented HPSs.
1) Abbreviation: UFHSP, unfermented high-protein soybean; FHSP, fermented high-protein soybean; UFSHPS, unfermented steam high-protein soybean; FSHPS, fermented steam high-protein soybean.
Previously, the study reported the sedative and hypnotic effect of the oral administration of L-ornithine in neonatal chicks exposed to stress (28). Ornithine has also been used to treat cirrhosis because it facilitates the removal of toxic ammonia from the liver (29). Here, the concentration of ornithine is 24.4 mg/kg, which is higher than that found by Pinho et al. (30) (from traces to 4 mg/kg). Ornithine is formed by the decarboxylation activity of LAB metabolism through the precursor’s arginine and citrulline during the early stages of the ripening of cheese (18). Thus, the higher concentration of ornithine in the SPY from HPSs with varied sprouting lengths is due to the decarboxylation of arginine and citrulline. In addition, from SPYs (FSHPS-2 to FSHPS-4), this amino acid’s concentration decreased, possibly because of degradation by arginase activity and proportionally lower concentrations of arginine in UFSHPS-2 to UFSHPS-4, in partial agreement with Diana et al. (18).
Importantly, the concentrations of GA were decreased in the fermented raw and fermented sprouting HPS sprouts compared to the unfermented HPSs, while the GABA concentrations were enhanced in the fermented raw and sprouting HPS sprouts (Fig. 2). As seen in Fig. 2, the UFHPS exhibits 4.19 and 1.81 mg/100 mL of GA and GABA, respectively, while the fermented HPS (FHPS) exhibits 2.93 and 3.75 mg/100 mL of GA and GABA, respectively. Interestingly, the sprouting of HPSs at 0 to 4 cm length showed increased levels of GA and GABA compared to the raw HPSs. In particular, at the 0 cm of the HPS sprouts, the UFSHPS-0 exhibits 23.35 and 17.85 mg/100 mL of GA and GABA, respectively. However, after fermentation (FSHPS-0), the free GA was reduced to 13.19 mg/100 mL, and consequently the GABA markedly increased to 32.46 mg/100 mL. Importantly, the GA and GABA were exhibited to maximums of 100.31 and 29.31 mg/100 mL at UFSHPS-1, while the GA markedly decreased to 45.09 mg/100 mL and the GABA increased to a maximum of 101.6 mg/mL in FSHPS-1. Similarly, the GA and GABA exhibited 92.66 and 50.46 mg/100 mL in UFSHPS-2, 17.33 and 77.32 mg/100 mL in FSHPS-2, 68.45 and 36.92 mg/100 mL in UFSHPS-4, and 21.88 and 85.94 mg/100 mL in FSHPS-4. The highest GA appeared in UFSHPS-1, while the highest GABA was found in FSHPS-1 among all the HPS sprouts tested in this study. However, there are significant differences in the GA content in SPY from varied sprouting lengths.
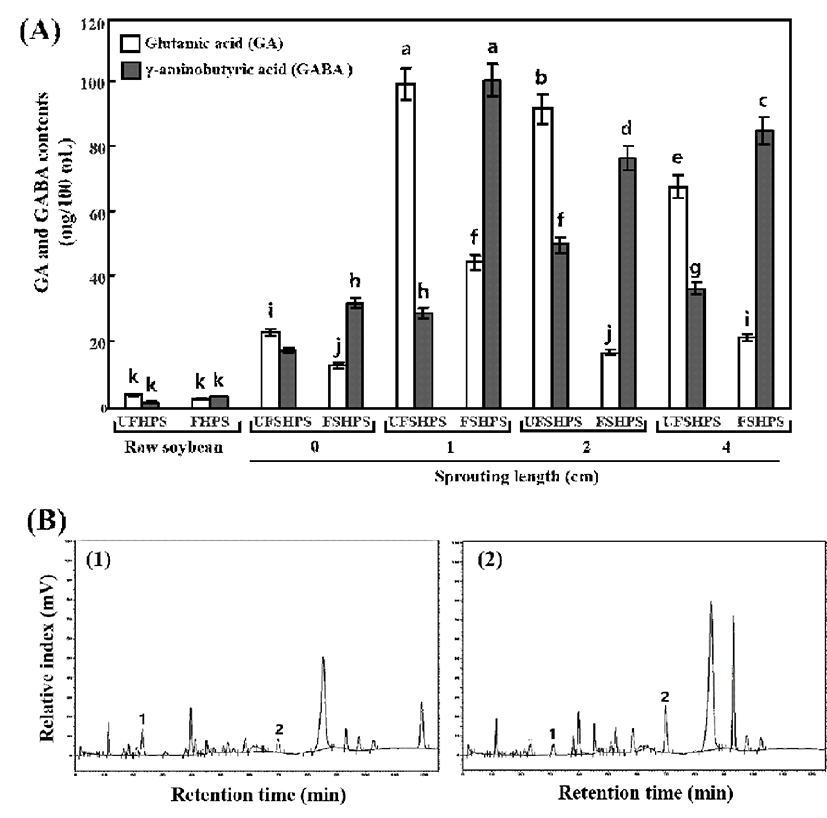
GABA-enriched black soybean milk produced by L. brevis FPA 3709 was reported (31). Some studies (32) reported that the glutamate decarboxylase reaction may be stimulated with an increase of GA, indicating that the increase in the GA level may be one of the reasons for the increase of GABA, which is in agreement with the present results. The GABA is mainly from the conversion of GA, while the GA is mainly derived from the breakdown of proteins during germination (33,34). The levels of GABA and GA are continually changing during germination, based on complex metabolic pathways. One explanation for this result is that GA is being produced while being converted and that the conversion of GA to GABA may have some influence on its production during germination, namely, the higher the conversion, the more production. Therefore, the highest concentration of GA in UFSHPS-1 was responsible for the highest concentration of GABA in FSHPS-1. Bai et al. (35) reported that there was a gradual increase in the GABA yield with the increasing substrate concentration, but a decrease occurred when the GA concentration exceeded 1.5 mg/mL in foxtail millet (Seratia italica L.) during germination process. Komatsuzaki et al. (15) suggested that the glutamate decarboxylase and GABA contents are regulated by GA addition. Makno et al. (36) reported that the relationship between GABA and GA was unclear in a study on vine-ripe tomato fruits under modified atmospheres. Thus, the complexity of the relationship between GABA and GA supports our result to some degree.
The total phenolic contents (TPCs) in the UFHPS, UFSHPSs, FHPS, and FSHPSs samples of raw and HPS sprouts are displayed in Fig. 3. The contents of TPCs increased after fermentation for raw and sprouting HPSs and were markedly higher in the sprouting of HPS yogurts compared to the raw HPS yogurt. The value linearly increased over the sprouting length of the HPS and reached a peak value at a 1 cm HPS sprouting length before decreasing with the extension of the sprouting length. In particular, the TPC levels were 1.2, 1.42, 1.76, 1.44, and 1.58 mg/g in UFHPS, UFSHPS-0, UFSHPS-1, UFSHPS-2, and UFSHPS-4, respectively, while they were 1.43, 1.63, 2.92, 2.30, and 2.54 mg/g in FHPS, FSHPS-0, FSHPS-1, FSHPS-2, and FSHPS-4, respectively. The soybean sprouts can non-linearly increase the TPCs value in the HPS.
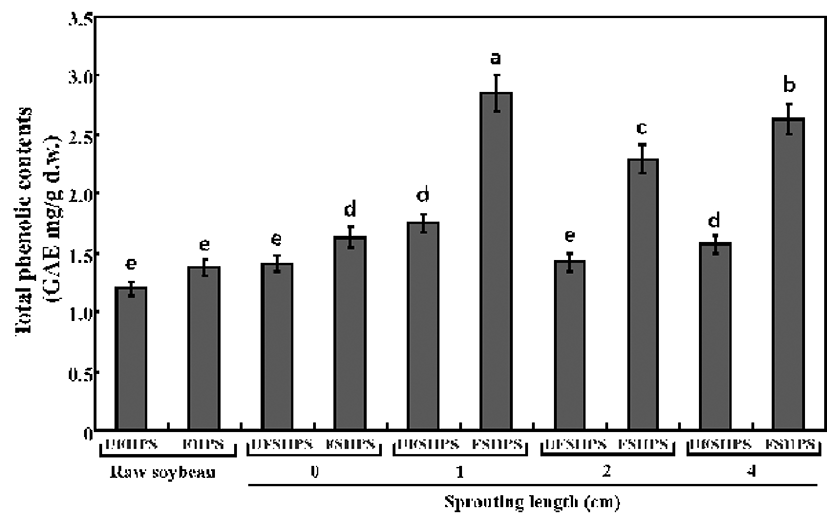
The maximum increase appeared in the 4-day-germinated soybean (14 cm sprouting length), an approximately 330% increase compared to the non-germinated soybean (4). In a related study, Lin and Lai (37) reported similar results that germination enhanced the phenolic content in most soybean cultivars, with the highest content exhibited in the 4-day-germinated bean. In this study, the TPCs were found to be maximum in UFSHPS-1 and FSHPS-1 and thereafter decreasing according to the increased sprouting length, which is in partially agreement with Huang et al. (4). In addition, our previous study reported that the TPCs were increased in SPM by fermentation with L. plantarum P1201 (25). The above results suggest that the L. brevis probiotic played a vital role in the biotrans formation of the SPY biopolymers into beneficial phenolics.
Germination can increase the isoflavone content and change the isoflavone composition of the HPS. Paucar- Menacho et al. (8) reported that the reduction of the 7-O-β -glycoside isoflavone after germination might be contributed to the β-glycosidases activated in germination, which could catalyze the aglycones released from the glycosides. In this study, the isoflavone glycosides were decreased slowly, accompanied by the accumulation of aglycones during the fermentation of SPY by L. brevis (Table 2 & Fig. 4). In fact, the total isoflavone glycosides are markedly increased according to the increased length (cm) of the sprouting of unfermented HPSs. Importantly, the total levels of isoflavone glycosides are proportionally decreased in the sprouting of fermented HPSs. In particular, UFHPS, UFSHPS-0, UFSHPS-1, UFSHPS-2, and UFSHPS-4 showed 852.6, 914.47, 909.65, 998.9, 1,062.65 μg/g of total isoflavone glycosides, which decrease to 88.97, 219.81, 53.36, 38.16, and 53.95 μg/g in FHPS, FSHPS-0, FSHPS-1, FSHPS-2, and FSHPS-4, respectively. Though the concentrations of daidzin, glycitin and genistin fluctuated in the fermented HPS sprouts, the overall tendencies were downward. The result indicates that UFSHPS-4 showed the highest levels of isoflavone glycosides compared to the other UFSHPS at various sprouting lengths (cm). Similarly, the malonyl-β-glycoside levels reached its highest level of 140.99 μg/g in the raw UFHPS compared to the other UFSHPSs. Slight changes in the acetyl-β-glycosides are observed after the fermentation of UFSHPS at various sprouting lengths. Interestingly, the total levels of isoflavone aglycones are increased after fermentation in FSHPS-0, FSHPS-1, FSHPS-2, and FSHPS-4. The levels of isoflavone aglycones are detected as 350.34, 289.15, 361.61, 445.05, and 491.25 μg/g in FHPS, FSHPS-0, FSHPS-1, FSHPS-2, and FSHPS-4, respectively. The total isoflavones reached maximums of 1,239.99 and 601.84 μg/g in UFSHPS-4 and FSHPS-4, respectively (Table 2). The ratio of isoflavone contents is shown in Fig. 4A. The glycosides are the highest in UFSHPS-0 (89.29%), followed by UFSHPS-1 (87.24%), UFSHPS-4 (85.7%), UFSHPS-2 (84.71%) and UFHPS (81.83%). As seen in Fig. 4A, the isoflavone aglycone concentrations are markedly increased after the fermentation of the raw and HPS sprouts of various lengths compared to the unfermented HPSs. In particular, after 72 h of fermentation, the isoflavone aglycone concentrations (%) were increased to 15.88-, 9.94-, 16.57-, 10.37-, and 12.52-fold for FHPS, FSHPS-0, FSHPS-1, FSHPS-2, and FSHPS-4, respectively, from their initial values. It is clearly shown in the typical HPLC chromatograms that the isoflavone glycoside levels decreased, while the isoflavone aglycones increased, during the fermentation of HPSs with various sprouting lengths (Fig. 4B). This result suggests that FSHPS-1 exhibited more isoflavone aglycones than the other fermented HPSs tested.
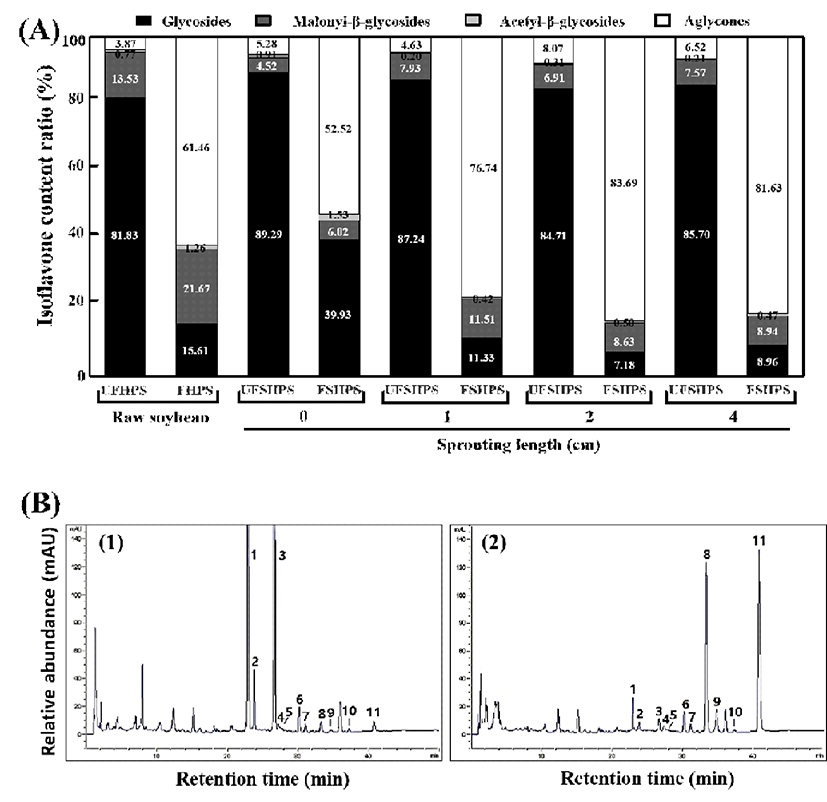
1) Abbreviation: UFHSP, unfermented high-protein soybean; FHSP, fermented high-protein soybean; UFSHPS, unfermented steam high-protein soybean; and FSHPS, fermented steam high-protein soybean.
It was reported that germination could affect the contents and compositions of isoflavones in the soybean (4). In this study, the content and percentage of aglycones were increased in the SPYs made from various lengths of sprouting. The enhancement of the total isoflavone content was proposed to be the biosynthesized through phenylpropanoid pathways (38) and malonate pathways (39). Therefore, in the SPY, the total aglycone enhancement is proportional to the total isoflavones that were synthesized during the sprouting of the soybean. It was reported that the ratio of aglycones to total isoflavones was improved by germination in black soybeans (37). Devi et al. (40) reported that the germinated soybean had the highest isoflavone content among the soybean products, including soy milk, soy sauce, soy meals and soy flour and soy seeds. In a related study, we found that the roasted soybean showed a maximum level of 598.6 μg/g of total isoflavones (3), while in this study, the SPY of the HPS showed a maximum level of 1,239.99 μg/g of total isoflavones, which is in agreement with Devi et al. (40).
The antioxidant activities of the unfermented and fermented HPS sprouts were analyzed according to the DPPH and ABTS radical‑scavenging activity (Fig. 5). The DPPH radicalscavenging activity in the UFHPS, 30.81%, markedly increased to 40.45% in the FHPS. It was observed to be 41.68% in UFSHPS-0, which slightly increased to 44.66%. The DPPH radical-scavenging activity in the UFSHPS-1 was 50.56%, which sharply increased to 63.28% in FSHPS-1. The levels sharply decreased to 41.24% and 49.98% in UFSHPS-2 and FSHPS-2, respectively. No significant changes were observed (48.81%) in the UFSHPS-4, but there was a great increase to 58.26% in FSHPS-4 (Fig. 5A). This result suggests that UFSHPS-1 and FSHPS-1 show the highest levels of DPPH radical‑scavenging activity compared to the other fermented and unfermented HPS sprouts. The ABTS radical‑scavenging activities were also observed to be the highest in UFSHPS-1 (60.56%) and FSHPS-1 (73.28%) compared to the other fermented and unfermented HPS sprouts, as seen in Fig. 5B.
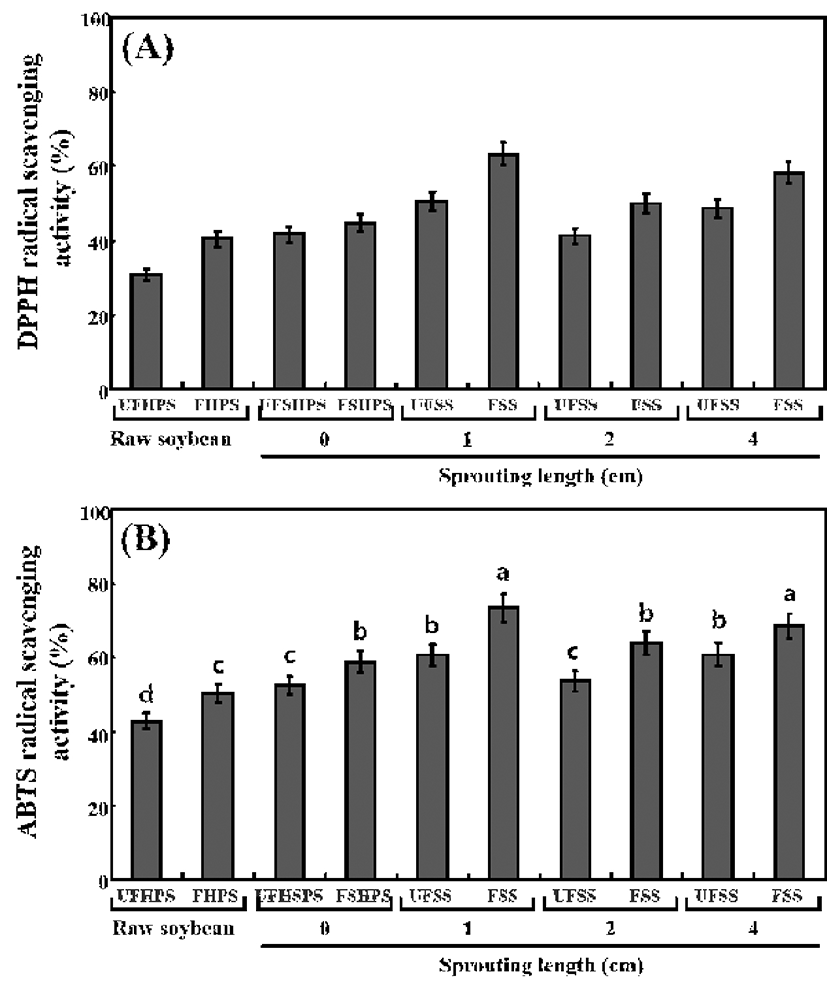
The antioxidant capacity is one of the biological activities of phenolic compounds that provide beneficial effects to humans (41). For the germinated soybean, the TPCs were enhanced during germination and reached its highest value after 4 days germination, but while the antioxidant capacities was also the highest then, the change in the TPCs were not proportional to the change in the antioxidant capacity (4). Because the highest TPCs and antioxidant activity were found in FSHPS-1 in this study, the change in TPCs are proportional to the changes in antioxidant activities. Previously, much of the research on isoflavones has reported that they had a low scavenging potential for DPPH radicals, with scavenging effects only half those of α-tocopherol and epicatechin (42). However, several studies revealed that phenolic compounds were responsible for the antioxidant activities of soybean seed, soy paste and soy curd (43,44). Juan and Chou (45) reported that the combined isoflavone and phenolic content accounted for nearly all of the in vitro antioxidant activity of black soybean extract. Recently, the TPC was higher in soybean sprouts than in soybean seeds, corresponding to antioxidant activity higher appeared (2). Therefore, it is expected that the high antioxidant activity of the fermented FSHPS-1 might be related to the markedly higher total phenolic and isoflavone aglycone contents achieved during fermentation.
In conclusion, the contents of total phenolics, isoflavone aglycones, GABA, and ornithine and antioxidant activities were significantly enhanced in the SPYs made of HPS sprouting of various lengths. Importantly, FSHPS-1 exhibits higher levels of these functional products than the others, such as FSHPS-2 and FSHPS-4, made of soybeans with various sprouting lengths. FSHPS-1 also showed the highest DPPH and ABTS radical scavenger activities. This result suggested that FSHPS-1 exhibits high nutritive value and functional properties, which make it a prospect for application in the soy-dairy industry.
요 약
본 연구는 발아된 고단백 콩(high protein soybeans, HPSs) 으로부터 가바(GABA)와 비배당체 이소플라본(isoflavone aglycones)이 증가된 콩-분말 요구르트(SPY)를 제조하였 다. 이를 위해 L. brevis로 발효 증자 고단백콩 발아체 (FSHPS, 0-4 cm)를 72시간 발효하였다. 발효 고단백콩 (FHPS), 0 cm 발효 증자 고단백콩 발아체(FSHPS-0), 1cm 발효 증자 고단백콩 발아체(FSHPS-1), 2cm 발효 증자 고단 백콩 발아체(FSHPS-2) 및 4 cm 발효 증자 고단백콩 발아체 (FSHPS-4)의 총 유리아미노산 함량은 각 79.53, 489.93, 877.55, 780.53 및 979.97 mg 이었다. 발효되지 않은 고단백 콩 발아체(UFSHPS-1, 1 cm) 및 FSHPS-1의 글루탐산(GA) 및 GABA 함량이 각각 최고 100.31 mg/100 mL 및 101.60 mg/100 mL로 관찰되었다. 또한 FSHPS-1에서 가장 높은 DPPH(63.28%) 및 ABTS(73.28%) 라디칼 소거능을 보였다. 그러나 FSHPS-4에서 isoflavone aglycone 비율이 81.63%로 가장 높았다. 특히, FSHPS-1은 높은 가바 함량과 기능적 특성을 나타내어 두유 산업에 응용할 수 있을 것이다.