Introduction
Ginger (Zingiber officinale Roscoe) is a perennial herbaceous plant of the Zingiberaceae native to subtropical and tropical regions, and it is grown in tropical and subtropical regions in China, India, Egypt, and Iraq (Lee et al., 2011). In Korea, ginger is mostly produced in Andong and Yeongju of the North Gyeongsang province and Seosan and Dangjin of the South Chungcheong province (Lee and Kim, 2016). The ginger root and stalk feature a unique spicy taste and aroma and are distributed as fresh ginger, dried ginger, and ginger oil, which are widely utilized as food, medicine, and cosmetics (Lee et al., 1979; Lee et al., 1996). Further, ginger is not only used as a spice worldwide but has been proven to have pharmacological efficacy, based on which it is utilized as digestive aid, medicine for nausea, abdominal pain, low back pain, and diarrhea, and antibacterial agents (Kim et al., 2001). Past studies have compared the constituents (Chung et al., 1996) and amino acid composition of ginger according to the growing regions (Takahashi et al., 1982), and studies have also investigated the spicy component (Chen et al., 1986) and aromatic component that is closely related to the flavor of ginger (Moon et al., 1997). Ginger has a moisture content of 80-90%, and starch accounts for 40-60% of the entire solid mass (Kim et al., 1991; Shin et al., 1994). The major ingredients of ginger include carbohydrates, ketones, alcohols, and volatile aromatic components, such as zingiberene and γ-cadinene. Ginger consists of 0.1-0.3% of oil, and the main ingredients of this oil, the 6-gingerol and 6-shogaol homologues, give ginger its characteristic potent spice, with their content markedly higher than that of other trace ingredients. For this reason, 6-gingerol and 6-shogaol are frequently used as quality indices during storage and distribution of ginger, and they have attracted much attention as potential ingredients of functional foods owing to their antioxidant and anti-inflammatory properties (Connell et al., 1969; Connell et al., 1970). Particularly, 6-gingerol has an antioxidant activity of equivalent to 95% of that of ascorbic acid (Lee et al., 2006), and as it stabilizes β-carotene (Lee et al., 1985), many studies have investigated its antioxidant (Chang et al., 1994), anti-cancer (Surh et al., 2002), and anti-bacterial (Ippoushi et al., 2003) effects. With its pharmaceutical effects documented, ginger, along with various herbs, is utilized in developing health supplements and crude drugs.
Ginger is generally harvested in October and November and stored and distributed throughout the year. However, ginger has poor storability, as it is susceptible to chilling injury at a temperature of 10°C or lower and is susceptible to germination (Enmaya et al., 1981), mold growth, surface drying, and softening at a temperature of 18°C or higher (Jo et al., 1996). In Korea, 58,947 tons of ginger was produced in 2016, with the production growing at an annual rate of about 15.4%, and the ginger market size is about 535.9 billion KRW, also growing at an annual rate of 14.2% (Chungcheongnam-do Agricultural Research and Extension Services, 2017), indicating growing demands. To address the challenges of ginger storage and increase the diversity of ginger consumer products, past studies have investigated the optimal quality characteristics of sweet-rice muffins containing ginger powder (Lee et al., 2011), rheological characteristics of pound cake containing ginger powder (Chung et al., 2012), quality characteristics of ginger juice-added muffins (Han et al., 2012), quality characteristics of apple jams made with ginger (Lee et al., 2014), quality characteristics of all-purpose flour containing ginger powder (Lee et al., 2021), and cookies containing ginger powder (Kwon et al., 2021). In terms of processed ginger, candied ginger accounts for nearly half of all processed ginger products (KAMIS). Jeonggwa (candied snacks) is a traditionally Korean snack prepared by lightly boiling plant roots, stalks, or fruits to soften the flesh and slowly braising it in sugar or honey (Kim, 1999). Ginger has a potent spicy flavor, so eliminating the spicy flavor during the candying process is more important for ginger than other types of jeonggwa. Ginger is generally treated with hot water to remove its pungent flavor, and during this process, its active ingredients are lost, and it may be browned or discolored.
While several studies have explored measures to enhance the storability of ginger and ginger-containing processed food products, studies on hydrothermal treatment, an essential step in producing candied ginger, are lacking. Thus, this study applied hydrothermal treatment to ginger slices at varying temperatures and durations and analyzed the changes in physicochemical, functional, and sensory characteristics to identify the optimal conditions for hydrothermal treatment that do not impair the quality of processed ginger products. The results of this study would be useful as basic data for producing processed products using ginger.
Materials and method
For this experiment, ginger produced in Seosan, South Chungcheong Province that are similar in color and size and has no blemishes. Ginger washed in running water was cut into 0.4-cm slices using a vegetable cutter (RG-100, Hallde, Kista, Sweden). The ginger slices were placed in a water bath (WMB-311, Daihan Scientific, Seoul, Korea) with water at a 1:10 (w/v) ratio and heated at 60°C, 70°C, 80°C, 90°C, and 100°C for 1 h. For the evaluation of suitability for food processing by temperature of hydrothermal treatment, the temperature was fixed to 80°C, which was the temperature at which the best results were obtained in the functional ingredient experiment, and to establish the optimal duration of hydrothermal treatment, ginger and water were heated in a water bath (WMB-311, Daihan Scientific, Seoul, Korea) at a 1:10 (w/v) ratio for 1 h, 2 h, 3 h, 4 h, and 5 h. The ginger slices were taken out immediately after the hydrothermal treatment and drained for an analysis of the characteristics.
For the pH and soluble solid content analysis, the hydrothermal-treated ginger slices were immersed in distilled water of a volume fourfold greater than the sample and ground using a homogenizer (AM-9, Nihonseiki Kashima Co., Ltd., Tokyo, Japan). The pH of the prepared sample was measured using a pH meter (pH 510 Benchtop Meter, Oakton Instruments, IL, USA), and the dilution factor was not reflected in the pH value. Soluble solid content was measured using a refractometer (Master-α, ATAGO Co., Tokyo, Japan) and presented as °Brix, and the dilution factor was not reflected in the measurement. Color was analyzed by arbitrarily choosing 10 samples from each treatment group and making three repeated chrominance measurements at the center of a cross-section of the ginger slices. The average value was used, and a colorimeter (CR-400, Konica Minolta Co., Osaka, Japan) calibrated with a standard white plate (L*=97.79, a*=-0.38, b*=2.05) was used. The color difference between pre- and post-treatment samples was calculated using the following equation:
A modified version of the Blois method (Blois et al., 1958) was used for the 1,1-diphenyl-2-picrylhydral (DPPH) radical assay. The sample was prepared through ultrasound-assisted extraction of the ginger slices in 80% ethanol (40 kHz, Daihan Scientific Co., Ltd., Seoul, Korea) for 30 min at room temperature (20±5°C) and four rounds of filtration with Whatman filters. 0.4 mM DPPH (Sigma-Aldrich, MO, USA) solution was adjusted with ethanol to achieve an absorbance of 0.95-0.99 and mixed with the sample solution at 4:1 ratio. After incubating the mixture for 10 min, absorbance was read at 517 nm using a UV-Visible spectrophotometer (Evolution 201, Thermo Fisher Scientific, MA, USA), and the DPPH radical scavenging activity was computed using the following equation:
ABTS radical scavenging activity was measured with reference to the method proposed by Re et al. (1999). 7.4 mM ABTS was mixed with 2.45 mM potassium persulphate (Sigma-Aldrich, MO, USA) at 1:1 ratio and incubated for 14 h in dark to form cation radicals (ABTS·+). The solution was then diluted such that the absorbance value is below 1.00 at 734 nm. 20 μL of sample solution was added to 980 μL of the diluted ABTS·+ solution and incubated for 10 min before reading absorbance. The result was presented as Trolox equivalent (μM) using Trolox (6-hydroxy-2,5,7,8-tetramethylchroman-2-carboxylic acid; Sigma-Aldrich, MO, USA) as the standard substance.
Total phenol content (TPC) was quantified based on the Folin-Ciocalteu method (Benvenuti et al., 2004). The sample was prepared through ultrasound-assisted extraction of the ginger slices in 80% ethanol (40 kHz, Daihan Scientific Co., Ltd., Seoul, Korea) for 30 min at room temperature (20±5°C) and four rounds of filtration with Whatman filters. 1 mL of the sample solution was mixed with 1 mL of Folin-Ciocalteu reagent (Junsei Chemical Co. Ltd., Tokyo, Japan), incubated for 15 min, mixed with 1 mL of 10% Na2CO3 1 mL (Duksan Pure Chemicals Co., Ansan, South Korea) and incubated for another 60 min in dark. Absorbance was measured at 700 nm using a UV-Visible spectrophotometer (Evolution 201, Thermo Fisher Scientific, MA, USA), and the TPC was presented as tannic acid equivalent (TAE) mg/L using tannic acid (Chameleon Chemicals, Osaka, Japan) as the standard substance.
To analyze the 6-gingerol, 6-shogaol contents of hydrothermal-treated ginger slices, the sample was prepared through ultrasound-assisted extraction in 80% methanol (40 kHz, Daihan Scientific Co., Ltd., Seoul, Korea) for 30 min and filtration through a 0.45-μm membrane filter. The methods proposed by Gorecki et al. (1997) and He et al. (1998) that enable simultaneous analysis of 6-gingerol and 6-shogaol were applied to perform high performance liquid chromatography (HPLC) (Model Prominence, Shimadzu, Kyoto, Japan). The YMC-Peak Pro C18 (4.6×250 nm, 5 μm, 120Å) was used as the column, with the temperature set at 30°C. A gradient system with water and acetronitrile was used for the mobile phase. The flow rate was set at 1.0 mL/min, and the volume of sample injection was set at 20 μL.
Sensory evaluation was performed by 15 adequately trained graduate students of the Gyeongbuk National University. The samples for the sensory evaluation were prepared by mixing the ginger slices treated at each experimental condition with sugar at a 2:1 ratio and braising it for about 40 min at 80°C. The evaluation consisted of color, appearance, odor, taste, ginger taste, hardness, chewiness, and overall preference using a five-point scale. The students were instructed to mark 1 for low preference due to off-taste and off-flavor and to mark 5 for high preference. The sensory evaluation was exempted for review by the Institutional Review Board at Gyeongbuk National University (Application number: 2021-0096) and proceeded safely.
Results and discussion
Table 1 shows the pH and soluble solid contents of ginger slices by heating conditions. The pH of ginger slices tended to decrease with increasing temperature of hydrothermal treatment and with increasing duration of hydrothermal treatment. This is consistent with previous findings that pH decreased with increasing temperature of thermal treatment of bellflower (Platycodon grandifloras) (Song et al., 2018). Kim et al. (2014) reported that hydrothermal-treated carrots had the lowest organic acid content compared to carrots with other types of pretreatment (steaming, stir frying). This was speculated to be due to the possibility that organic acids were volatilized by heat or were extracted into the hot cooking water (Lee and Jung, 2012). Further, this result was similar to the report by Lee et al. (2005), where pH increased over time after hydrothermal immersion treatment as organic acids were extracted. In general, pH change is closely linked to organic acid content and is used as a marker of biochemical change, and it has been reported that prolonged thermal treatment at high temperatures facilitates the biochemical changes of ginger (Chung et al., 2009). Soluble solid content of ginger slices significantly decreased with increasing temperature and duration of hydrothermal treatment. This is in line with the results of Lee et al. (2015) that total sugar content of burdock declines with steaming due to the extraction of water-soluble sugar ingredients and that of Yoon et al. (2005) that the soluble solid content of heat-treated ginseng decreases with increasing temperature and duration of heating.
Table 1 shows the color (CIE L*a*b*) results of heated ginger. The L* value significantly decreased with increasing temperature of hydrothermal treatment but did not differ according to the duration of hydrothermal treatment. The a* value decreased below that of non-treated samples with weaker heat treatment, resulting in a greenish color. However, the value increased to a level comparable to the non-treated samples with increasing temperature and duration of hydrothermal treatment. In other words, weak hydrothermal treatment can actually cause ginger to develop a greenish color. The b* value significantly decreased with increasing temperature and duration of hydrothermal treatment. Curcumine, the yellow pigment found in ginger, is insoluble in cold water but dissolves well in warm water and is vulnerable to heat (Kim, 2007). Thus, the extraction of curcumine into the hot water seems to have resulted in a lower b* value of ginger slices (Jung et al., 2004; Jung et al., 2012). Fig. 1 shows the ∆E and browning index (BI) of the non-treated sample and treated samples. The ∆E value significantly increased from 6.59 to 15.15 with increasing treatment temperature but did not markedly change according to the duration of treatment. Considering that there were no significant changes when the temperature was fixed at 80°C and durations of treatment were extended, the surface color of ginger seems to be more heavily influenced by the temperature of treatment rather than the duration of treatment. Regarding the BI, the BI was lower than the non-treated group when treated with 60-80°C for 1 h, but the index increased to a similar level to that of the non-treated group when the temperature was increased to 90°C or higher. When the temperature was fixed to 80°C and the duration was varied, the range of BI was significantly lower (45.90-48.27) than the non-treated group, with no significant differences among the treated groups. These results were similar to that of Kang and Hyun (2007), where curcumine content did not significantly differ according to the duration of water extraction of turmeric (30-180 min) but did significantly differ according to the temperature of extraction. As shown in Table 1, the L* value is more sensitive to the temperature of treatment, rather than the duration of treatment, where it markedly decreases at higher temperatures, thereby also affecting the BI. Jo et al. (1996) reported that browning of ginger generally is due to the Maillard reaction primarily involving fructose and asparagine and nonenzymatic browning involving the oxidation of ascorbic acid, and that the brightness value decreased. Chun et al. (1986) showed that when glucose+glysine solution and fructose+glysine solution were heated at varying temperatures, the Maillard reaction was dramatically facilitated from a temperature of about 90°C.
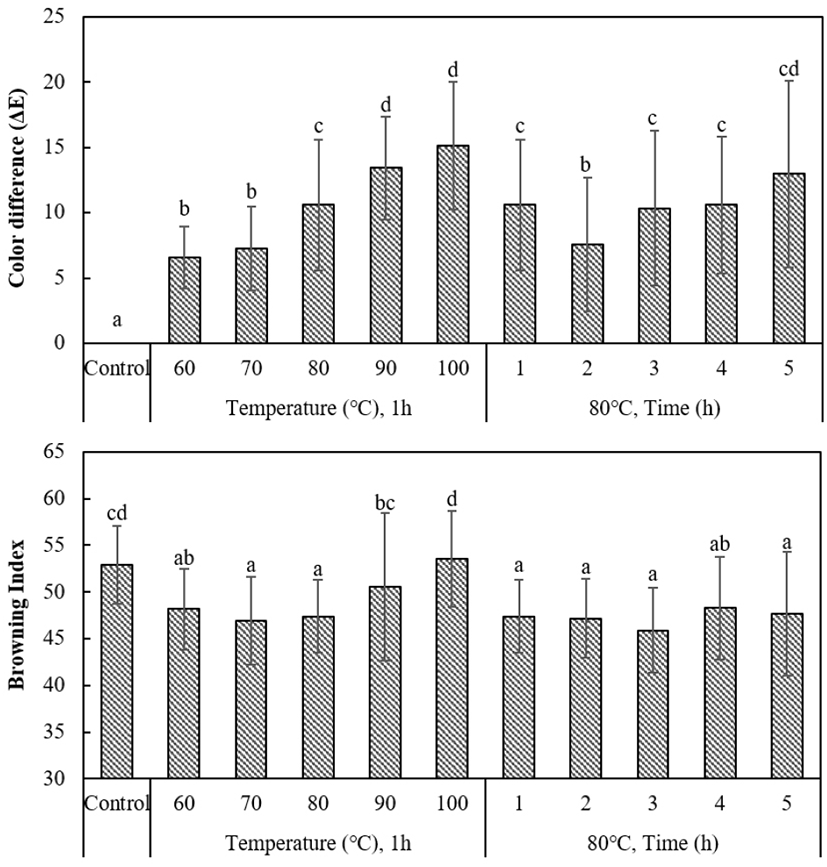
In conclusion, treatment with hot water at 60-80°C facilitated the extraction of curcumine into the hot water, resulting in a brighter color or lower BI compared to the non-treated samples. However, hydrothermal treatment at a temperature of 90°C or higher triggered the Maillard reaction and caused browning.
Table 2 shows the DPPH and ABTS radical scavenging activities and TPC by heating condition. DPPH radical scavenging activity of ginger slices increased with increasing temperature of hydrothermal treatment and peaked at 80°C, after which it declined. This is similar to the results of Ross et al. (2011), where radical scavenging activity increased proportionally to heating temperature and duration and then decreased from a certain point. The decreasing DPPH radical scavenging activity with increasing duration of heat treatment is speculated to be due to the extraction or alteration of the active ingredients of ginger slices as a result of extended heat treatment. Further, based on the degree of change, DPPH radical scavenging activity seems to be more heavily influenced by the temperature of treatment than duration of treatment. The ABTS radical scavenging activity of ginger slices increased with increasing temperature of heat treatment and peaked at 80°C (596.91 μM), after which it declined. The scavenging activity decreased with increasing duration of heating, similar to the results of DPPH radical scavenging activity. The higher ABTS radical scavenging activity compared to the DPPH radical scavenging activity is reported to be due to the difference in the radical-eliminating mechanisms and substrate binding (Re et al., 1999). The TPC of ginger slices was the highest at 80°C and tended to decrease with increasing duration of hydrothermal treatment. This is similar to the results of Kim et al. (2008), where TPC of fruits and vegetables increased with increasing temperature of heat treatment and decreased from a certain point. Yoon et al. (2005) reported that phenolic compounds were more easily extracted from ginseng with higher heating temperature and duration and that insoluble phenolic compounds were isolated from high-molecular compounds and degraded to free phenolic compounds, consistent with the findings of this study. Furthermore, DPPH and ABTS radical scavenging activity and TPC were higher at a temperature of 80°C or higher early in the heating (1st h) compared to the control (raw ginger), presumably due to an elevation of melanoidins, a brown product of the Maillard reaction (Do et al., 1989). However, as prolonged heat treatment may actually break down phenol components (Kim et al., 2014), and antioxidant substances are released into the hot water, the TPC may be lower after long heat treatment compared to the earlier values. In conclusion, the antioxidant capacity and TPC of ginger slices were more substantially influenced by temperature changes than the duration of treatment, with the values peaking after 1 h of treatment at 80°C.
Table 3 shows the 6-gingerol and 6-shogaol contents, the major functional ingredients, of ginger following hydrothermal treatment. 6-gingerol content was the highest in the 70°C group (215.55 mg/L) and began to decrease from 80°C with the lowest content in the 100°C group (95.77 mg/L). This is similar to the results of Bhattarai et al. (2001) that 6-gingerol content decreases at high temperatures because it is converted to 6-shogaol at high temperatures. 6-Gingerol prevents phospholipid oxidation by the iron chloride ascorbate (FeCl3-ascorbate) system and inhibits xanthine oxidase, an enzyme that contributes to the formation of reactive oxygen species such as superoxide anions (Lee et al., 2006). 6-Shogaol is produced by dehydration of gingerol and has been reported to have biochemical effects such as antibacterial and antioxidant effects. The 6-shogaol has been reported to have superior anti-inflammatory, antioxidant, and anti-cancer effects compared to 6-gingerol (Chang et al., 1994). 6-Shogaol content remained unchanged at low temperatures but increased at higher temperatures, peaking at 100°C (15.87 mg/L). It also significantly increased with increasing duration of heating, with the highest content (16.71 mg/L) in the 5 h group. These results are similar to those reported by Ok et al. (2012), where 6-shogaol content of ginger increased with increasing drying and extraction temperatures. Further, Cheng et al. (2011) also reported that 6-shogaol content increases with decreasing 6-gingerol content and is inversely proportional to the degree of exposure to heat during processing. Therefore, the temperature or duration of hydrothermal treatment should be raised to increase the content of 6-shogaol, a functional ingredient, in ginger.
Table 4 shows the results of sensory evaluation of candied ginger prepared by braising hydrothermal-treated ginger slices with sugar at a 2:1 ratio. The appearance preference score for the control group was 3.5, and the sample with the highest preference score for appearance was the ginger hydrothermal treated at 60°C for 1 h. Similarly, the preference for color was also the highest for the 60°C treatment group, followed by control group, 70°C group, 80°C group, 100°C group, and 90°C group. Ginger slices treated at higher temperatures and for longer durations would have had the Maillard reaction involving sugars and amino acids that causes browning (Kim et al., 2018), which would have contributed to less preferred appearance and color. The preferences for odor, taste, ginger taste, hardness, chewiness, and overall preference did not significantly differ according to the conditions of hydrothermal treatment. In terms of the duration of heating, the 2 h group was rated to have the most preferred appearance and color, and the preference score tended to decrease with increasing duration of treatment beyond this point. On the other hand, there were no significant trends observed for odor, taste, and chewiness. The sample treated at 80°C for 5 h was given a markedly lower score for ginger taste and overall preference. The browning caused by longer duration and higher temperature of heating led to significantly different color, and particularly, browning caused by long pre-treatment influenced the overall preference for candied ginger. However, the heating conditions did not have noticeable effects on the properties, taste, and odor overall. Thus, heating ginger at lower than 80ºC for less than 3 h would not alter the sensory qualities of candied ginger.