1. Introduction
Persimmons (Diospyros kaki Thunb.) are a globally cultivated fruit, with significant production in its native region of East Asia (Nam et al., 1998). Various horticultural varieties exist and they are generally classified as astringent or non-astringent, which determines their post-harvest consumption method (Kim et al., 2004). Among the astringent varieties, Cheongdobansi is a variety produced in Cheongdo, North Gyeongsang Province, at a rate of approximately 40,000 tons per year. Cheongdobansi becomes edible only after astringency removal or softening owing to its high water-soluble tannin content. Furthermore, it has generally been processed and distributed as a soft persimmon owing to its tender flesh and lack of seeds (Cheongdo Agricultural Technology Center, 2023). However, it is increasingly processed and distributed in a slice-dried form for extended shelf life and added value (Kim et al., 2009). Cheongdobansi are harvested in late September and kept refrigerated until the peeled fruits are processed into dried and slice-dried persimmons for mass distribution. Therefore, one major drawback is that a large quantity of persimmons must be processed within a limited time frame (Kim et al., 2018; Praeger et al., 2018). For this reason, it is important to develop post-harvest storage technologies that ensure that the fruit remains safe for human consumption, minimize external and internal physical damage to the fruit, and preserve its quality characteristics to maintain consistency in the quality of dried persimmon products.
Various processing methods are utilized to preserve the quality of fruits that are harvested en masse at a single time point, such as low-temperature storage (Kim et al., 2017), high CO2 treatment (Kim et al., 2012), UV irradiation (Jang et al., 2012), hot water treatment (Park and Kim, 2002), controlled atmosphere (CA) storage (Chung et al., 2005), modified atmosphere storage (Kim et al., 2017), and 1-methylcyclopropene treatment (Win et al., 2017). Among these, ClO2 treatment is utilized to enhance the storage quality of agricultural produce (Kim et al., 2016). Some key benefits of ClO2 are that it is readily degraded in the air, leaving no residue on the food after treatment, and it can be applied over a broad pH range (pH 3-8) (Malka and Park, 2022). Furthermore, owing to its high water solubility and stability as dissolved gas in aqueous solutions, it is primarily used for fumigation in gaseous and aqueous forms (Moon et al., 2017). In contrast to chlorine, which is generally used to extend the storage of various fresh agricultural produce, ClO2 does not generate carcinogens such as trihalomethane, or alter the nutritional and sensory qualities of the produce because it does not react with organic substances (Praeger et al., 2018). Moreover, ClO2 has been approved as a disinfectant for fresh agricultural produce and food processing industries owing to its efficacy and safety (FDA, 1998). Maintaining the storage quality of agricultural products requires technologies for regulating their respiration rate and ethylene biosynthesis. ClO2 controls water transpiration by regulating the opening and closing of plant stomata (Chen and Zhu, 2011), and prevents weight loss by reducing the respiration rate (Guo et al., 2014). Furthermore, it delays ripening by regulating ethylene biosynthesis-related genes, namely ACS2, ACO1, and ACO3 (Guo et al., 2013). It can also prevent spoilage by inhibiting microbial growth, thereby preserving the sensory characteristics of fruits and vegetables, such as color and flavor (Chen et al., 2010).
ClO2 gas has been used to enhance the storage quality of fruits such as tomatoes (Yang et al., 2020), strawberries (Kim and Hwang, 2019), paprika (Kang et al., 2015), and broccoli (Park and Lee, 2018). Studies that have used ClO2 have primarily directly treated fruits and vegetables with aqueous ClO2 (Park et al., 2008) or investigated the optimal concentration of ClO2 gas (Kim et al., 2005). Various studies have been conducted to improve the storage quality of persimmons, including CO2 gas adjustment (Cia et al., 2006), combined treatment with potassium lactate and heat (Naser et al., 2018), and edible coating based on gum arabic (Saleem et al., 2020). However, there is still a paucity of research on extending the storage quality of Cheongdobansi using ClO2 gas. Therefore, this study analyzed the quality characteristics of Cheongdobansi over the storage period based on the duration of ClO2 gas fumigation, and evaluated the possibility of extending the shelf life using this treatment. Therefore, this study analyzed the quality characteristics of Cheongdobansi over the storage period based on the duration of ClO2 gas fumigation. The chemical, physical, and sensory properties of the Cheongdobansi were evaluated and compared after various storage periods, with untreated samples were used as a control. From these results, we evaluated the possibility of extending the shelf life using this treatment.
2. Materials and methods
The persimmons used in this study were a variety of astringent persimmon known as Cheongdobansi (Diospyros kaki T.). The persimmons were harvested in October 2022 and were purchased from a farm in Cheongdo, North Gyeongsang Province. Following harvest, the control group (CONT) was analyzed immediately (week 0). Subsequently, all other samples were stored at 0±1°C and 30% relative humidity. Persimmons stored under a low temperature conditions only (PNT), persimmons stored under low temperature conditions after two weeks of ClO2 fumigation (P2T), and persimmons stored under continuous chlorine dioxide fumigation (PAT) were analyzed over a 10 week period at two-week intervals. ClO2 gas was generated using a ClO2 gas generator (CA-300, Purgofarm Inc., Yongin, Korea) fabricated according to the electrochemical method described by Gates (1998). The high-purity ClO2 gas generated from a NaClO2 aqueous solution passed through a porous membrane electrode assembly, underwent a series of electrochemical reactions, and flowed into the collection chamber via a vent. The gas stream was controlled with a regulator valve to maintain a ClO2 gas concentration of 60 mg/L in the storage facility (Analytical Technology, Inc., Collegeville, PA, USA).
A smartphone (iPhone 11 Pro, Apple Inc., CA, USA) was placed 60 cm above a non-glossy white background to capture images of the appearance of the persimmons. To minimize shadows, a studio photo box (Puluz Photo Studio Light Box, Shenzhen Puluz Technology Co., Ltd., Guangdong, China) was used with 1,690 LM LED lights installed on two sides. Then, the Cheongdobansi was halved and placed on the white background to photograph its appearance. All pictures were taken in a dark room at 20°C.
Changes in the moisture content were determined using the air-oven method of the Codex Alimentarius. A 3 g sample was weighed and spread on a aluminum dish. The sample was then dried in an oven at 105°C for 5 h and cooled in a desiccator for 30 min before measurement. The results were expressed as a percentage (%) using the following equation:
W0: the initial weight of sample.
W1: the weight of sample after drying.
A quarter of the Cheongdobansi flesh was minced using laboratory scissors and ground at 8,000 rpm for 30 s. The ground sample (10 g) was mixed with distilled water (400 g) in a media bottle (50 times dilution). An ultrasonicator (DH WUC, D22H, Daihan Scientific Co., Ltd., Wonju, Korea) was used to extract the mixture for 30 min at 200 W (50% of the maximum ultrasound power) at 40 kHz. Then, the extract was filtered through a Whatman® No. 2 filter paper to prepare the final Cheongdobansi extract. The soluble solid content and pH were measured three times using a refractometer (0-32 °Brix) (Maste-α, Atsgo Co., Tokyo, Japan) and pH meter (Orion 3-Star, Thermo Scientific Co., Waltham, MA, USA), respectively.
A random sample was selected and cut at a point 1.5 cm away from the stem, and the chromaticity of the cut surface was measured. A colorimeter (CR0400, Minolta, Tokyo, Japan), calibrated with a white plate (L*=61.04, a*=35.70, b*=58.02), was used to take 10 measurements of the L* (lightness), a* (redness), and b* (yellowness) values, and the average values were calculated. The color difference was calculated using the following equation based on the measured L*, a*, and b* values:
ΔL*: Difference lightness value between the sample stored for 0 d
Δa*: Difference redness value between the sample stored for 0 d
Δb*: Difference yellowness value between the sample stored for 0 d
Hardness was measured using a texture profile analyzer (Compac-100II, SunScientific Co., Tokyo, Japan). A No.5 (Φ 5 mm) probe was used and the test conditions were set as follows: test mode, 20; probe penetration distance (%), 5 mm; table speed, 180 mm/min; and maximum load cell stress, 2 kg. The sample was cut at a point 1.5 cm from the stem, and 10 cut surfaces were measured to calculate the average.
The total sugar content was quantified using the phenol-sulfuric acid method (Dubois et al., 1956). The Cheongdobansi extract prepared in Section 2.5 was diluted 800 times. A mixture of 1 mL of the diluted solution and 0.5 mL of 5% Folin-Ciocalteu’s reagent (Sigma Chemicals, Co., St. Louis, MO, USA) was prepared. This mixture was incubated with 2.5 mL of sulfuric acid (Duksan Pure Chemicals Co., Ltd., Ansan, Korea) in a water bath at 85°C for 15 min and then left to stand at room temperature for 30 min. The absorbance was measured at 480 nm using a UV-Visible spectrophotometer (Evolution 201, Thermo Fisher Scientific Inc., Madison, WI, USA). Glucose (Duksan Pure Chemicals Co., Ltd., Ansan, Korea) was used as the standard material for calculating the sugar content, and a standard curve was generated to express the total sugar content as glucose equivalents.
The soluble tannin content was measured using the Folin-Denis method (Folin and Denis, 1912). The Cheongdobansi extract prepared in Section 2.5 was diluted eight times. One milliliter of the diluted solution was mixed with 1 mL of Folin-Ciocalteu’s reagent (Sigma Chemicals, Co., St. Louis, MO, USA) at a 1:1 ratio and allowed to stand in a dark room for 15 min. After adding 10% Na2CO3 (Duksan Pure Chemicals Co., Ltd., Ansan, Korea), the mixture was vigorously shaken and left to stand in the dark for 1 h. The absorbance was measured at 760 nm using a UV-Visible spectrophotometer (Evolution 201, Thermo Fisher Scientific Inc., Madison, WI, USA). The soluble tannin content was calculated from a standard curve prepared with tannic acid (Sigma Chemicals, Co.).
A sensory evaluation was conducted with 15 students from Kyungpook National University. The evaluation parameters were: degree of clearness, weakness, sweet flavor, and off-flavor, using a seven-point scale (1: very opaque or weak to 7: very clear or strong). Based on this, the overall acceptability was evaluated on a seven-point scale (1: very poor to 7: very good). The highest score for marketability was set to 2. Five Cheongdobansi samples were selected and each Cheongdobansi was cut into quarters before providing them to the participants. This sensory evaluation was conducted safely and with final exemption from the Kyungpook National University Institutional Review Board (IRB) (Approval number: KNU-2022-0488).
The results were analyzed with one-way analysis of variance (ANOVA) using the SPSS (Statistical Package for Social Sciences, SPSS Inc., Chicago, IL, USA) software package. Duncan’s multiple range test was performed to analyze the significance of the differences across storage periods and samples. The significance of the differences among the samples at week 2 were analyzed with the t-test (p<0.05).
3. Results and discussion
Table 1 shows the changes in the weight loss rate of Cheongdobansi under different ClO2 storage conditions. There were no significant differences in the moisture content based on the storage period. However, after week 8 of storage, the PAT and P2T samples had slightly higher moisture contents. Lee et al. (2011) reported that the moisture content of Cheongdobansi immediately after harvest ranges from 80.40-84.70% depending on the harvesting time. Hence, all samples showed a high moisture content overall until week 10 of storage. In general, the moisture content of fresh produce decreases with increasing storage time due to increased transpiration (Park et al., 2004). The resulting skin shrinkage causes the appearance of the fruit to change, which directly affects the sensory quality characteristics of the fruit (Choi et al., 2012). Kim and Hwang (2019) reported that ClO2 treatment suppresses moisture loss in fruits by reducing respiration rates. They confirmed that continuous ClO2 treatment throughout storage is more effective than a one-time treatment with ClO2 gas early in the storage period to maintain the quality of strawberries. Moreover, Ku et al. (2006) demonstrated that a combination of low-temperature and ClO2 treatment lowered the weight loss of Agaricus bisporus during storage by regulating their transpiration and respiration. In addition, Wang et al. (2014) found that extended ClO2 treatment delays transpiration in plums. Therefore, ClO2 treatment has a positive effect on maintaining the initial moisture content throughout storage.
1) CONT, no treatment; PNT, only stored at 0±1℃ and RH 30%; P2T, chlorine dioxide was treated at concentration 60 mg/L for 2 weeks and after stored at 0±1℃ and RH 30%; PAT, all was stored by chlorine dioxide treatment at concentration 60 mg/L, and stored at 0±1℃ and RH 30%.
Table 1 shows the changes in the soluble solids content and pH of the Cheongdobansi under various ClO2 storage conditions. The soluble solids content of fruits generally indicates sweetness and is used as an objective indicator of palatability. The pH not only affects microbial growth but also directly affects the quality and safety of food (Kim et al., 2005). The soluble solids content of the Cheongdobansi exhibited no significant differences among the samples or storage periods. Besada et al. (2009) reported that the soluble solids content of persimmons did not differ significantly between ripening stages. Similarly, Chang et al. (2007) found that the soluble solids content of grapes treated with ClO2 packaging remained similar from the beginning of storage until week 10 of storage. The pH of the Cheongdobansi did not exhibit significant differences overall over the days of storage, although a slight increase was observed with increasing storage period. No trends were observed between the results and the storage treatment method. Ultimately, ClO2 gas treatment did not affect the pH of Cheongdobansi. Similarly, Du et al. (2007) reported that the pH of bell peppers was not affected by the ClO2 gas concentration during storage.
Table 2 shows the changes in the internal chromaticity (CIE L*, a*, b*) and color difference (ΔE) of the Cheongdobansi during storage under different ClO2 treatment conditions. In terms of lightness (L* value), all samples exhibited a decreasing trend during storage. In particular, PNT exhibited a greater variation in L* value compared to those of the P2T and PAT samples over the storage period. In terms of redness (a* value), there was an overall increasing trend followed by a decreasing trend during storage; however, no significant differences were observed among the treatment groups. In terms of yellowness (b* value), all samples exhibited a steady decreasing trend during storage. The decrease was most pronounced in PNT, which were stored at a low temperature. At week 10, the color difference (ΔE) was 9.97±1.39 for PNT, 7.42±3.05 for P2T, and 5.74±2.12 for PAT, indicating that the greatest color change occurred in the low-temperature-stored sample. This was visually confirmed by the overall darkening of color, as shown in Fig. 1. Moreover, a significant difference was observed between P2T and PAT from week 8 of storage. The internal color of a fruit is an important factor for determining fruit quality throughout ripening (Mendoza and Aguilera, 2004). Indicators of deteriorating appearance during the storage of persimmons include color change and injury. A decrease in lightness and yellowness and an increase in redness are the main causes of color change (Jeong, 2001). Overall, PAT exhibited small color differences throughout storage, and the internal color changes in terms of lightness, redness, and yellowness were smaller compared to those of the other samples. Therefore, it can be inferred that continuous ClO2 treatment during storage helps maintain the internal color of Cheongdobansi. Similar trends were observed in studies by Mahmoud et al. (2007) and Choi et al. (2013).
1) CONT, no treatment; PNT, only stored at 0±1℃ and RH 30%; P2T, chlorine dioxide was treated at concentration 60 mg/L for 2 weeks and after stored at 0±1℃ and RH 30%; PAT, all was stored by chlorine dioxide treatment at concentration 60 mg/L, and stored at 0±1℃ and RH 30%.
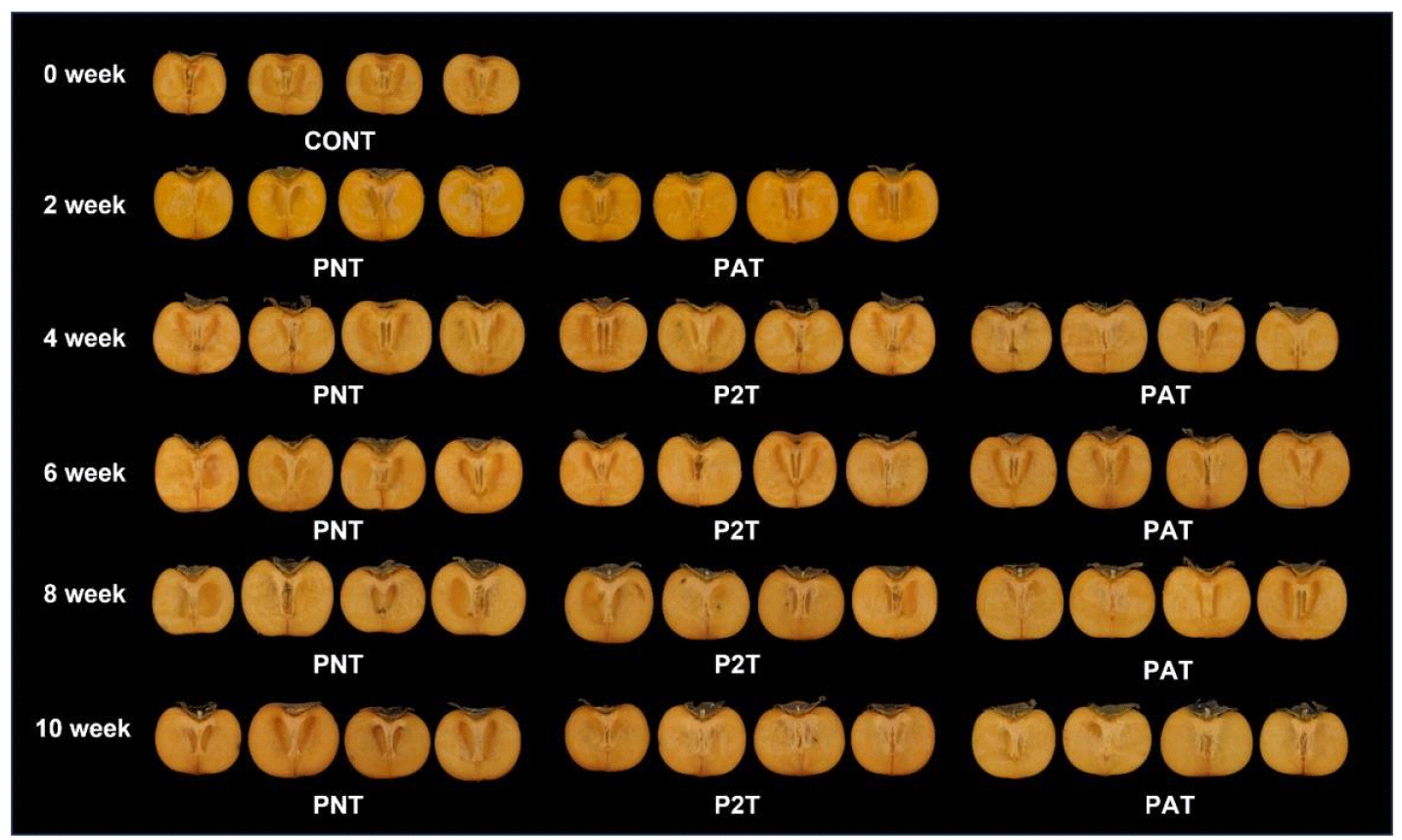
Fig. 2 illustrates the changes in the hardness of the Cheongdobansi under different ClO2 storage conditions. Hardness, measured using a texture profile analyzer, refers to the force required for the material to yield or deform during the first compression or shear state (Peleg, 2019). Therefore, hardness is considered an index of the mechanical properties of fruits (King and O’Donoghue, 1995). The hardness of the PNT samples decreased significantly with increasing storage period. P2T had a greater hardness than that of PAT until week 6. However, the hardness declined overall after week 8. In other words, hardness decreased in all samples with increasing storage period, although P2T and PAT showed significantly higher hardness values than PNT. Moreover, all samples treated with ClO2 gas maintained similar hardness values to those of week 4 during storage. During the ripening process, fruits soften owing to the enzymatic breakdown of the cell walls and pectin. Therefore, the ripening process affects the mechanical characteristics of fresh fruits (Chauhan et al., 2006). ClO2 fumigation delays ripening by inhibiting the activity of factors involved in ethylene biosynthesis, which is the basis for regulating the quality of fresh produce (Guo et al., 2014). Consequently, respiration decreases and softening is delayed, which suppresses alterations in the mechanical properties of Cheongdobansi during storage. Therefore, ClO2 fumigation is expected to have a positive effect on the storage quality of Cheongdobansi by preserving its hardness, a major factor in evaluating the storage quality of fresh persimmons (Kim, 1999). Similar results were found in the studies of Choi et al. (2013) and Kang et al. (2015), in which ClO2 gas treatment maintained the hardness of cherry tomatoes and paprika.
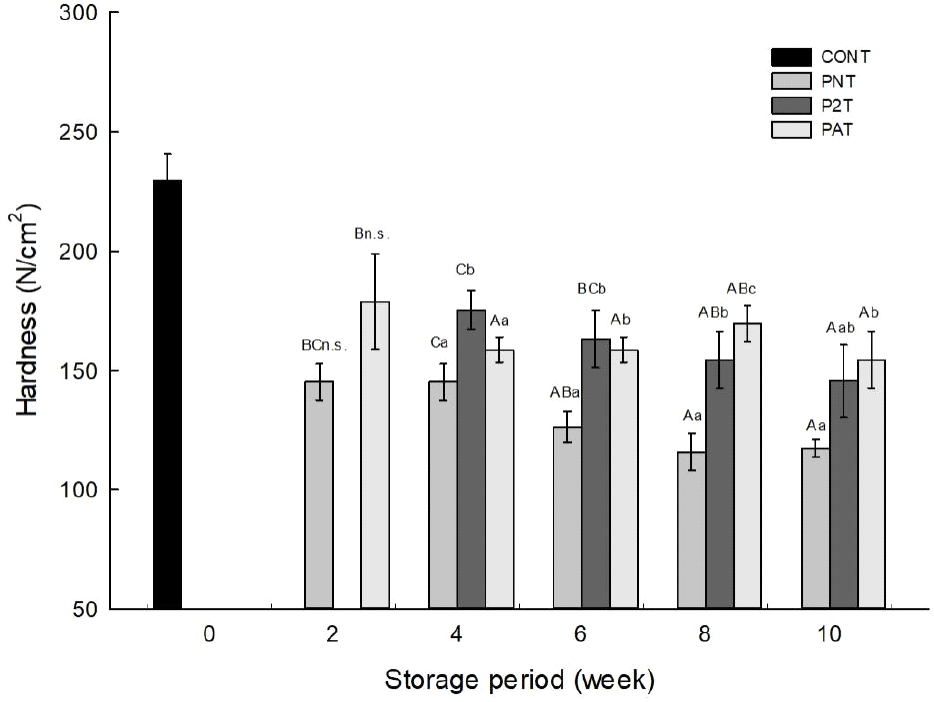
The primary sugar components in astringent persimmons are sucrose and fructose. The glucose content increases throughout ripening as the sucrose is broken down into glucose and fructose by invertase (Jeong et al., 2010). Table 3 shows the changes in the total sugar content during the storage of Cheongdobansi under different ClO2 treatment conditions. Overall, there were no significant differences in the total sugar content based on the storage treatment. However, PNT showed a slight increase in the total sugar content with increasing storage time from week 6 of storage. The trends in the soluble solids and total sugar contents were generally similar. ClO2 treatment has been reported to affect the enzymatic breakdown of cell wall components and suppress fruit softening (Kim et al., 2018). This may be the reason for the increasing trend in total sugar content in the PNT samples, which were stored under low-temperature conditions only. Jiang et al. (2022) reported that ClO2 treatment suppressed the increase in the total sugar content of grapes during storage.
1) CONT, no treatment; PNT, only stored at 0±1℃ and RH 30%; P2T, chlorine dioxide was treated at concentration 60 mg/L for 2 weeks and after stored at 0±1℃ and RH 30%; PAT, all was stored by chlorine dioxide treatment at concentration 60 mg/L, and stored at 0±1℃ and RH 30%.
Plant-derived tannins are water soluble and coagulate the mucosal proteins on the tongue, resulting in a strong astringent taste that affects palatability (Seo et al., 2000). However, the tannin content gradually decreases as they react with the acetaldehyde produced and accumulated in fruits during the late ripening process. In other words, the astringency of the fruit decreases during storage (Shahkoomahally and Ramezanian, 2013). Table 3 shows the changes in the soluble tannin content of Cheongdobansi during storage under different ClO2 treatment conditions. Generally, the samples treated with ClO2 showed a higher soluble tannin content than those stored at low temperatures only over the days of storage. The PNT sample showed a sharp decrease in tannin content starting from week 6 of storage. The PAT and P2T samples showed a slow decreasing trend during storage; however, no trends between the tannin content and the treatment conditions were observed. ClO2 treatment can delay the polymerization of tannin molecules by suppressing the ripening and softening of persimmons. However, the PNT samples ripened rapidly, resulting in the transformation of the tannins from soluble to insoluble, which led to a relatively low soluble tannin content. Sim et al. (2016) reported that the tannin content of a water extract of unripened Cheongdobansi was 2.88 mg/g. Therefore, the reason for the high tannin content may be due to differences in the harvesting time (Bian et al., 2015).
In general, persimmons are harvested in a single harvest for distribution. During this process, they can ripen and become soft owing to respiration and transpiration (Jeong et al., 2010). As persimmons ripen, their internal transparency and sweetness increase, while their mechanical properties deteriorate. Consequently, decay can occur, which can critically impact the sensory quality characteristics. Therefore, we evaluated the sensory qualities of Cheongdobansi stored under different ClO2 conditions in terms of clearness, weakness, sweet flavor, off-flavor, and overall preference (Table 4). Overall, the clearness increased with storage time, and P2T and PAT treated with ClO2 exhibited low levels from week 6. This can be observed visually in Fig. 1, in which the transparent area is wider. Kim et al. (2009) confirmed the correlation between tissue texture and clearness in persimmons. A similar trend was observed between the transparency and weakness. Compared to the CONT samples, the weakness mostly increased with increasing storage time, which was inversely proportional to the hardness trend. The samples treated with ClO2 exhibited relatively low weakness compared to PNT. In particular, the value was significantly lower overall in PAT than in P2T. This indicates that ClO2 treatment can delay changes in the mechanical properties of Cheongdobansi over the storage period. According to Cha et al. (2019), the sweetness of a fruit increases as the fruit ripens, and the sweet aroma also increases overall throughout the storage period. In particular, a significant difference was observed between the samples stored under different conditions at week 10. The common causes of off-flavor are environmental contaminants, microbial growth, lipid oxidation, or enzymatic degradation. Among these causes, spoilage from microbial growth has the greatest impact on the flavor of the sample (Wikes et al., 2000). However, the off-flavor values in all samples were similar to that of CONT, and no significant differences were observed based on the storage period or condition. This suggests that no microbial decay or accumulation of foreign substances occurred due to ClO2 treatment, and the flavor characteristics were not affected. The overall preference score for all the stored samples was lower than that for CONT, and it tended to decline as the storage period increased. However, the preference was higher for the ClO2-treated samples than for PNT over the storage period. In particular, the participants showed a high preference for PAT until week 10 of storage. In conclusion, ClO2 treatment improved the sensory qualities of Cheongdobansi during storage, with PAT treatment in particular maintaining good storage quality.
1) CONT, no treatment; PNT, only stored at 0±1℃ and RH 30%; P2T, chlorine dioxide was treated at concentration 60 mg/L for 2 weeks and after stored at 0±1℃ and RH 30%; PAT, all was stored by chlorine dioxide treatment at concentration 60 mg/L, and stored at 0±1℃ and RH 30%.
4. Summary
In this study, we comparatively analyzed changes in the quality of Cheongdobansi after varying durations of ClO2 gas fumigation treatment under low-temperature storage conditions. Treating Cheongdobansi with ClO2 gas helped maintain the moisture content, color, hardness, soluble tannin content, and sensory characteristics. However, ClO2 gas treatment did not affect the soluble solids content, pH, and total sugar content. Regarding the duration of ClO2 gas treatment, the quality of the PAT samples, which were continuously treated with ClO2 gas, was better preserved after 6-8 weeks of storage. In particular, their mechanical properties, namely hardness and weakness in sensory evaluation, were the most influenced by ClO2 gas treatment. These findings suggest that a packaging system that continuously releases ClO2 gas during distribution could contribute to preserving the freshness of Cheongdobansi. Additional research is needed to shed light on the correlation between stoma opening and closing and ripening based on the duration of ClO2 gas treatment. Based on these findings, identifying the optimal ClO2 gas treatment duration for each type of fruit could enhance the distribution and storability of fruits and vegetables.