1. Introduction
Rice (Oryza sativa L.) is one of the leading food crops in the world, which feeds almost half of the world’s population as a staple food (Chen et al., 2019). Rice is widely cultivated in all parts of the world and it is considered the most important grain crop in the world after wheat (Soto-Gómez and Pérez-Rodríguez, 2022). It belongs to the Poaceae family and is native to the ancient civilization of Southeast Asia (Pokhrel et al., 2020). Almost 90% of the world’s rice is grown and consumed in Asia, and 11 Asian countries produce 87% of the world’s rice (Bandumula, 2018).
Rice quality is evaluated based on characteristics that can be classified in different ways. Product attributes can include inherent taste, texture, or color, or external to the product, such as packaging, branding, or labeling. Another attribute category distinguishes between search, experience, and credibility attributes (Liu et al., 2022). Search characteristics for product evaluation before purchase include price, appearance, brand and packaging. Experience attributes can only be considered based on product experience, so after purchasing or using the product - attributes include taste, texture, cooking convenience, and swelling capacity. Credit attributes are those where consumers cannot evaluate or verify the product themselves, but rather they rely on individuals or institutions including government controls or industry claims (Enagi et al., 2021).
The visual characteristics of rice grains are important search characteristics that influence consumers’ purchase decisions and thus are considered as part of the first selection criteria in variety improvement models (Kholová et al., 2021). Seed size is more related to its length and on the other hand, the grain shape is related to the ratio of length to width. The normal classification system used by the breeding programs of the International Rice Research Institute (IRRI) is reported below: (<5.50 mm) as short, (5.51-6.60 mm) as medium/intermediate, (6.61-7.50 mm) as long, and (>7.50 mm) as very long. The shape of rice grains is also defined based on the usual value ranges used in IRRI: bold (<2.0), medium (2.1-3.0), and slender (>3.0) (Cuevas et al., 2016).
Rice grain quality is determined by a set of different characteristics. Each of these traits may be controlled by multiple sets of genes that likely interact with each other, resulting in additive, dominant, epistatic, or pleiotropic effects (You et al., 2022). These factors affect biochemical pathways and thereby cause functional properties. The expression of these genes may also be affected by environmental conditions. For this reason, to predict rice grain quality based on genetics, genetic information - and its possible complications - with grain quality phenotypes as well as possible interactions with the environment should be considered. The quality of rice grains can be examined from various aspects including eating, cooking, storage, chemical, nutritional and physical (depending on the concept that best represents its final consumption value) (Sharma and Khanna, 2019).
Due to the existence of different views, the concept of grain quality for rice is not easily clear. The aim of this article emphasizes the quality of rice grain to its physical and cooking characteristics. The normal physical characteristics used in the grading of milled rice are: the moisture content of the grains, the number of paddy (unmilled rice grains) in 1 kg of milled rice and the percentage of foreign matter, chalky immature grains and broken grains. In this regard, rice grain quality can be considered as a measure that a certain sample of grains meets a set of physical criteria specified by a known and valid grading system (Koklu et al., 2020). There are different systems for grading rice and tools for measuring the value of rice grains at the level of production and exchange (Custodio et al., 2019). In Asia, different rice grading standards have a lot in common despite different historical backgrounds and legal systems, so that all grading standards are sensitive to moisture content above 14% (wet basis), impurities, damaged grains or grains with waste materials and ultimately lead to improved yield and uniformity of rice grains (Koklu et al., 2020).
The characteristics of cooking and processing rice from a historical point of view have usually been important factors in high consumption areas of rice in the world. The quality of cooking and processing as well as the quality of milling are the main quality indicators that affect the economic value of rice grains (Bergman, 2019). Cooking index and rice processing quality can be checked in different ways. In the United States, one of the best ways to describe cooking and processing quality is based on defined chemical and physical conditions that can be considered as specific quality indicators for rice (Custodio et al., 2019). Rice is one of the main foods of people in the Kurdistan region, and there is little information available on its physicochemical properties. Collecting data and examining the physicochemical properties and cooking characteristics of the most commonly consumed rice in the market can be fruitful in formulating appropriate models to guide consumers, in preparing plans and reports of international institutions, as well as in the improvement programs of cooking characteristics by plant breeding specialists.
2. Material and method
The dried rice grains were prepared in January 2020 from the city market in Erbil, Kurdistan Region-Iraq. In total, fifteen different rice cultivars (1-15) were bought. Rice samples were collected from six different stores to check the physicochemical characteristics of each variety (each approximately 3 kg). The rice samples were kept in closed plastic bags at room temperature until the tests, and each measurement was performed in several replicates (enough to ensure a significant result). The physical and chemical tests were performed in the Laboratory of Food Technology of Salahaddin University-Erbil.
A vernier caliper with accuracy of 0.01 mm was used to determine the length L (mm), width W (mm), and thickness T (mm) of the rice kernels. Rice grains were randomly taken, and each dimensions were noted and recorded 20 times for each variety (Nádvorníková et al., 2018).
The following equation was used to measure the Equivalent diameter De (mm) (Nádvorníková et al., 2018):
The grain shape, which is considered here as the ratio of length to width, is obtained from the following equation:
Where L is the average length of milled rice (mm) and W is the average width of milled rice (mm) and L/W is the ratio of length to width of milled rice. According to the ratio of length to width, the seeds were grouped with classification provided by IRRI: narrow (>3.0), medium (2.1-3.0), bold (1.1-2.0) and round (<1.1) (Myint et al., 2023).
The previously recorded variables of length, width and thickness were used to calculate the surface area S (mm2). The following formula was described by (Hu et al., 2022) for surface area.
The sphericity of rice φ (−), was defined by the formula mentioned by (Steven et al., 2022):
Volume of grain V (mm3) was obtained from the formula mentioned by (Ewaid et al., 2020) as following:
This index was determined by randomly counting 1,000 seeds from each sample and weighing those selected grain (Pokhrel et al., 2020).
Moisture content of samples was determined according to the following equation (Azmi et al., 2021):
Where, m1 is the initial weight of grain and m2 is the weight of grain after drying.
Firstly the broken rice was separated before cooking and unbroken and uniform grains of rice was cooked by water with 1:2.5 ratio for all samples, because all rice varieties can absorb this proportion of water when optimally cooked. The cooked rice in each dish was covered with a piece of filter paper quickly (weighed down by the upturned aluminum cover and then stirred with a spatula to break the Lumps, once after 5 min and again after 15 min. Rice samples from two or more dishes were then pooled and transferred into a big Petri dish with a piece of filter paper placed below the lid. The Petri dish was allowed to stand for l h at room temperature about 30°C).
Viscosity of rice samples (Rice Hour pastes) were determined by Bostwick consistometer. A glass beaker containing the flour slurry (8%) was placed in a boiling water bath and stirred (approximately 20 rpm) for 15 min. It was tried to apply the minimum amount of shearing force to the mixture and then the hot dough was cooled to 70°C. A Bostwick constimeter was used to determine the relative viscosity measurements as distance (cm) the 70°C paste traveled over the metal slide in 30 seconds (Phongnarisorn et al., 2023).
In order to perform the alkali spreading test, seven whole kernels of each sample was taken in Petri dish at three replications and 20 mL of potassium hydroxide (0.3 M), 1.7 g in 100 mL of distilled water, was poured to the sample and maintained for 23 hours at room temperature of 21°C. The spreading value of each replication was evaluated visually on a 7-point numerical scale. Finally, average scores of seven kernels were counted as the spreading value (Nadaf et al., 2016).
The method of Saleh and Meullenet (2015) was used to determine the gel consistency.
The experiments were carried out under laboratory condition with at least three replications. The data obtained from the experiments were entered and calculated some physical index in Microsoft Office (Excel, 2013). Analysis of variance (one way-ANOVA), post hoc test using Tukey, PCA, and graphs were performed using Minitab (Version 17) statistical software.
3. Results and discussion
As shown in Table 1, the average length of the rice samples were between 5.05 and 8.75 mm while average width were between 1.37 and 1.97 mm and a significant different was also observed (p<0.05). Sample 5 and sample 14 were recognized with the longest length and width, respectively. Grains grouped as more than 6 mm long (long), 5 mm to 6 mm (medium) and less than 5 mm (short) by Adewusi et al. (2022). According to this classification, most of the tested rice samples were long grain, which have good potential for high price in the market. The ratio of length to width that determines the shape and size varies between 2.58 and 5.97. Brass samples with a ratio greater than 3 are classified as narrow, ratios between 2 and 3 grains are classified as bold, and ratios less than 2 are classified as round (Köten et al., 2020). Most of the tested samples had a narrow shape and only sample 12 has a bold shape. The shape of the grain affects its volume and weight. Narrow varieties of rice occupy more volume compared to round varieties and this in turn causes narrow varieties of rice to require more storage space for the same weight compared to other types of rice (Li et al., 2018).
Grain shape which related to its length, width and their ratio, is a vital index for estimating end-use quality in rice (Sattari et al., 2015). The first step to study on the rice shape is determining the grain dimensions, and also other values which further used for many dimensional measurements. Length, width, and thickness for Ozgon rice variety were evaulated by Smanalieva et al. (2015) at various storage period. Due to the classification of grain dimensions and the amount of off-size in the market ranking, measuring the dimensions and their ratio is of significant importance. The highest aspect ratio was found in Bekhtyari as an Iraqi rice (0.49) while lowest in Sevan Aspi resh as an Indian rice (0.18), the second one is belonging to the imported varieties rice (Table 2). Varnamkhasti et al. (2008) introduced the highest aspect ratio with 0.51 and the lowest with 0.40 for variety of Kyrgyz origin had Batken and Kyrgyz group had Ozgon Cerza, respectively. The values of 0.28-0.29 were recorded in their measurements while Nádvorníková et al. (2018) measured the same variable for different cultivars of rice aspect ratio (0.39-0.55). Our related results are also in the normal range compared to those measurements.
1) No.1-15 refer to Table 1.
Measuring grain volume is essential for modeling grain aeration and therefore for all drying devices, heating and cooling processes. The volume of the rice grain ranged from 4.48 mm3 to 17.74 mm3 (for example, Indian rice from 6.78 mm3 for Sevan Aspi resh to 9.23 mm3 for Royal Bakhtyari and Pakistan rice from 5.49 mm3 for Falak Exreme to 7.82 mm3 for Mahmood rice) (Table 2). The results of volume of Iranian rice were reported between 13.94 mm3 and 26.75 mm3 by Varnamkhasti et al. (2008). In onother research, the values of volume were recorded between 21.1 mm3 and 36.4 mm3 (Kawamur, 2015).
The surface area and thickness of the rice grain strongly affect the diffusion of water during the cooking process (Jogihalli et al., 2017). Also, the optimal rice cooking time is related to the thickness and surface of the specific grain (Nádvorníková et al., 2018). According to Mohsenin (1986), the sphericity values of raw rice have been reported between 0.32 and 1. In consistent with this word, the results of the present study are also included in the Mohsenin’s hypothesis range (0.32-0.56). Some authors reported the sphericity in the range of 0.32-0.53 in paddy Indian rice (Nádvorníková et al., 2018). Varnamkhasti et al. (2008) also recorded the sphericity values of Iranian rice between 0.37 and 0.46.
Based on the obtained results, the surface values of the tested samples were significantly different. The separation of the mixture of grains during the cleaning of cozen by the air flow is related to the ratio between the air speed and the final speed of the particles, as well as the amount of particles entered per unit volume of the air flow. The heat transfer surface significantly affects the rate of heat transfer to the material. Less material volume per unit area accelerates heat transfer. The effects of size and surface area on the drying speed of particles can be determined by the surface-to-volume ratio. When water diffusion in particles delays the drying rate, larger particles take longer to dry than smaller particles of the same shape. Finally, it can be said that the ratio of surface to volume affects drying time and energy supply (Varnamkhasti et al., 2008).
The true density of the grain mixture is measured by means of a solution or suspension. Apparent density values between different samples had a significant difference at the 5% probability level. In advanced agriculture, various approaches are used to calculate grain volume in the combine hopper. Apparent density is necessary to calculate the weight of the product in the hopper. Pneumatic sorting tables are used to separate the seeds of cereal crops with real density. Cereal grains differ greatly in true density from grains of various impurities such as centaure, rye grass, field mustard, and wild oats. The apparent density affects how the grain behaves in the dry mass. Whereas, the solid density is more concerned with the specific volume that the grains take up in space, when calculated by the water test.
Varnamkhasti et al. (2008) reported the results of bulk density between 0.47 and 0.55 g/cm3 and claimed that bulk density is critical in the design of warehouses, silos, and hoppers, as well as in the transportation and storage of rice because it can be said that the higher the bulk density, the less space rice can be packed. Researchers have introduced bulk density as a critical index during cooking. The thousand grain weight of rough rice is also recognized as a pleasant index to “milling outturn” for calculating the percentage of dockage or waste material in many rough rice, and the content of immature kernels or shriveled.
The correlations among the physical attributes of tested rice were described by PCA and were presented graphically. In fact, PCA was performed to analysis the information consisted of the multivariate data of tested rice with most important information. The eigenvalues and variance percentage are shown in scree plot (Fig. 1(A)) to recognize the number of principal components to be selected for further study. The first (PC1) and second (PC2) components retained 63.4% and 34.8 % of the total variance, respectively while in total, other principle component (PC3, PC4, PC5 and PC6) obtained below than 1% of total variance. As shown in Fig. 1(B), PC1 was mostly related with hectoliter, broken ratio and moisture content while PC2 was mostly concerned with hardness and true density (Table 3). In PC1 (63.4%), the variables like hectoliter, broken ratio and moisture content were positively correlated whereas the hardness and true density were negatively correlated. The second principal component PC2 (34.8%), showed negative correlation with all obtained variable.
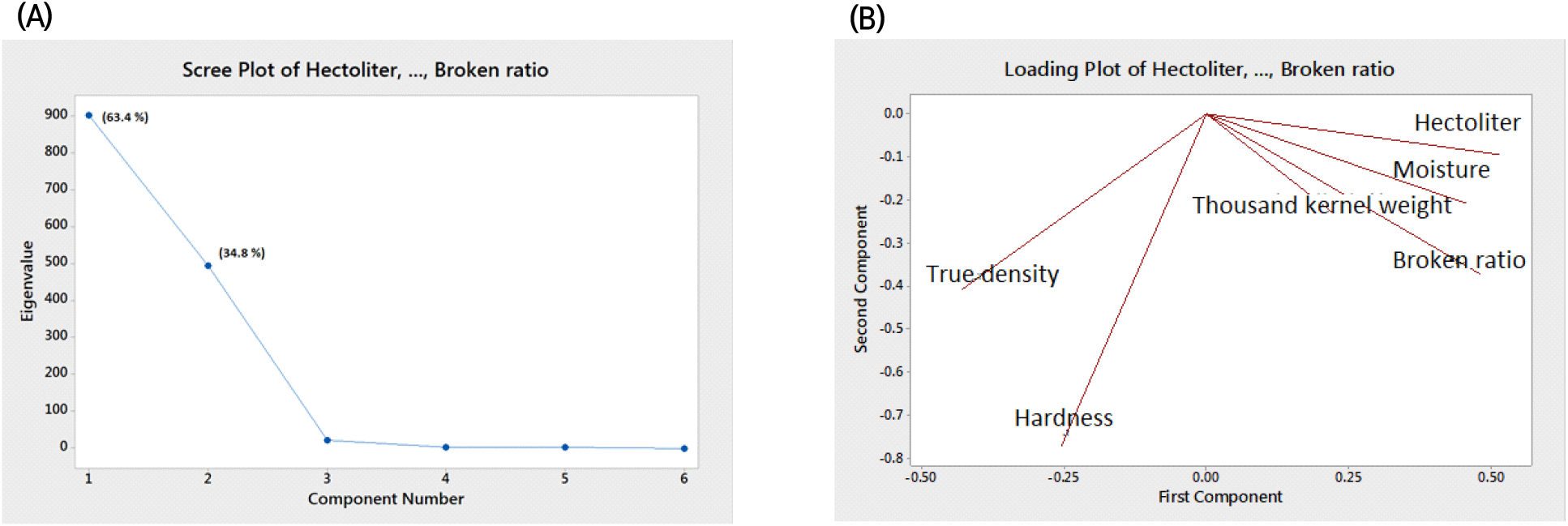
Gel consistency is used as an indirect method to screen cooked rice for its hardness during cooking. The consistency of hard gel is the result of the formation of hard rice gel. Therefore, the association of starch polymers in the aqueous phase and medium gel consistency is due to the effects caused by small genes. In order to strengthen rice improvement programs to select soft texture rices, gel consistency analyzes were introduced as an indirect method for normal selection of cooked rice hardness for rices containing more than 25% amylose. The gel consistency test calculates the distance traveled after 1 hour of gel produced from KOH and rice flour. In hard-textured rice, a short gel is formed and only travels a short distance, but for softer-textured rice, a viscous gel is produced and travels up to 10 cm within an hour (Hu et al., 2022). Gel consistency (GC) is a standard criterion used in rice quality improvement models to identify rice cultivars/breeding lines with high amylose content with soft or firm texture during cooking (Jeazet et al., 2022).
Rice ASV is a widely measured quality index as well as a valid gelatinization temperature (GT) index that was discovered in 1958. More precise methods to measure ASV and GT, for single kernel and bulk rice, are important tools to determine the effect of single kernels on final consumption quality and maximum cooking quality rice. ASV is a mostly reliable indicator of the GT class and is a method with reasonable efficiency to be used to improve rice breeding programs for selection from a large number of progeny isolates (Armstrong et al., 2022). To measure ASV, alkaline test is the most common method which shows the dispersibility of milled rice in a dilute alkaline solution with dispersion grade and mainly uses a 7-point numerical score system for evaluation. The ASV scores obtained from the single core alkali tests were used to group the samples into two groups: intermediate GT related to ASV scores of 2-5 or low GT related to ASV scores of 6-7 (Table 4).
1) No.1-15 refer to Table 1.
The hardness is one of the important parameters for evaluation of cooked rice texture were measured. As shown in Fig. 2, the results cleared that the hardness values of the samples after boiling were significantly lower than uncooked samples (p<0.05). The researchers explained the importance of stickiness and hardness in eating quality, how they are interrelated, and how they can be effectively measured with a tissue analyzer. However, they found organoleptic tests essential to study rice quality, with descriptive tests being the most objective tests and preference tests specifically explaining variations between evaluators, locations, and cooking conditions (Sharif et al., 2017). According to Fig. 1, it can be observed that samples 14 and 15 (Cihan Group and ADM rice from Greece and USA, respectively) have lower hardness than other samples before baking, and sample number 1 (Mania, Indian) has the highest hardness. But in general, after cooking, the hardness of most samples decreases, although with different intensity. In the meantime, it can be mentioned that the samples related to Indian rice recorded a greater decrease in hardness during cooking. At the similar research, Duangkhamchan et al. (2017) compared the quality properties of milled rice before and after IR heating under optimized conditions (53.6°C and 1.2 min) during 4-month storage and they reported a significant decrease in hardness of treated and untreated samples (Duangkhamchan et al., 2017).
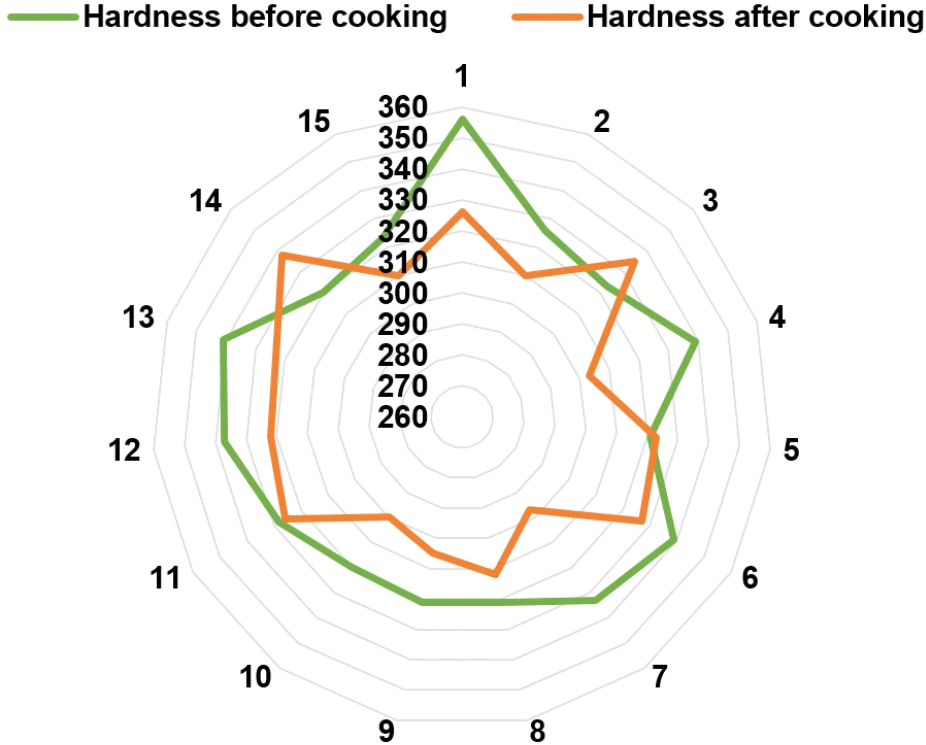
Measurement of water uptake ratio played a role in the set of analyses, as it reveals other textural characteristics based on rice rather than simply cooking. The water uptake ratio was recorded for three temperatures including 30, 45, and 60°C for 30 min. Fig. 3 summarize the results of samples after 30-min soaking. The significant difference between behaviors of tested rice was detected when rice sampled were soaked at different temperatures for 30 min. With rising temperature and fixing the soaking time, water uptake ratio steadily increased in Indian rice including (Mahmood rice, Bakhiyari rice and Sevan Aspi resh rice) (Fig. 3). In contrast, the Pakistan and Turkey cultivars rice showed considerable different behavior with a decreased in water uptake ratio at 60°C. As can be observed in the general scheme of Fig. 3, although in general, increasing the temperature improved the water uptake ratio of the rice samples. However, the intensity and direction of this trend is not the same for all samples at all tested temperatures. For example, by increasing the temperature from 30°C to 45°C for most of the samples, no significant increase in their water uptake ratio is detected, while by increasing the temperature to 60°C, a significant increase in the water uptake ratio is recorded for most of the samples. Many of researchers carried out the tests with different methods in comparison with our study. For example, Shittu et al. (2012) used the same temperature levels (30, 45, and 60°C) for water uptake ratio characterization. They also investigated the unusual behavior of rice at 45°C and related it to some microstructural details that have not yet been determined for different rice varieties. However, in their results, the gradual increase of values in all stages as a result of increasing temperature was contrary to this study (Shittu et al., 2012). In consistent with our results, Shittu et al. (2012) reported some irregular trends of rice as well. For instance, unexpected reduction was found in hydration rate with paddy rice of ITA 150, ITA 301, and WAB 450 with increasing soaking temperature from 45 to 60°C. This reduction in hydration rate is maybe due to partly swelling of the grains which could lead to a reduction in porosity of the material and this decreases water uptake ratio in turn.
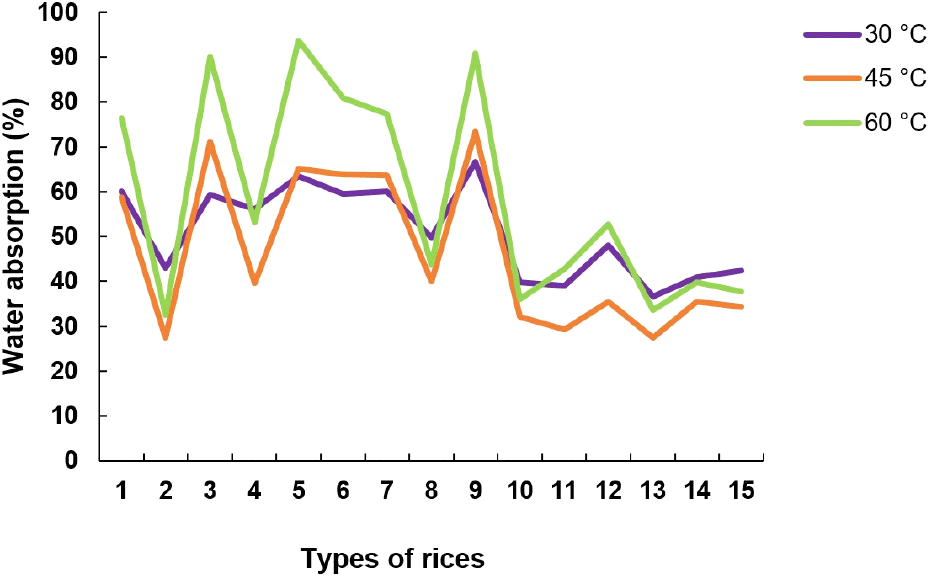
The correlations among the cooking properties of tested rice were also described by PCA. The eigenvalues and variance percentage are shown in the scree plot (Fig. 4(A)) to recognize the number of principal components to be more focused. The first (PC1) and second (PC2) components retained 88.5% and 9.3% of the total variance, respectively while in total, other principle component (PC3, PC4, PC5 and PC6) obtained about 2% of total variance. As shown in Fig. 4(B), PC1 was mostly related with viscosity, spring value and hardness after cooking properties while PC2 was mostly concerned with spring value, hardness before cooking and hardness after cooking (Table 5). In PC1 (88.5%), the variables spring value and hardness after cooking properties were positively correlated whereas the viscosity and hardness before cooking properties were negatively correlated. The second principal component PC2 (9.3%), showed a positive correlation with spring value, hardness before cooking and gel consistency properties while negatively correlated with hardness after cooking and viscosity.
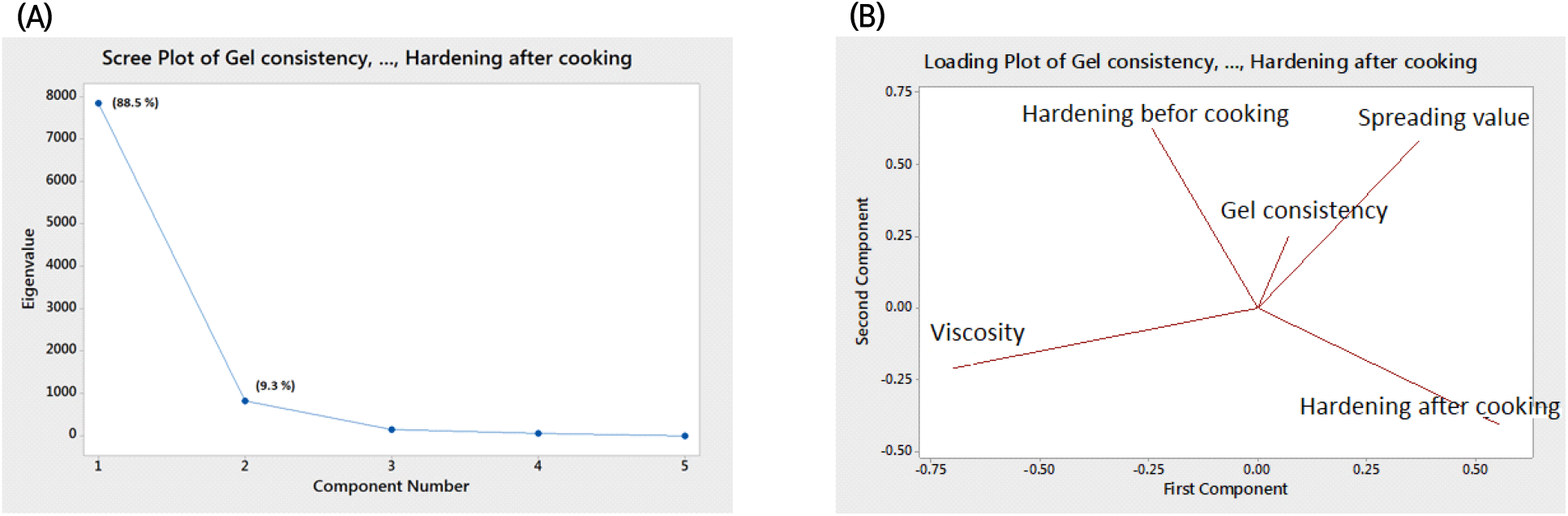
4. Conclusion
This study generally focused on the physicochemical and cooking characteristics of 15 types of common rice collected from Kurdistan market. The preliminary analysis of the results showed that more than half of the rice collected from the market originates from India and Pakistan and also except for the local rice from Iraq and Turkey, which were round and bold, the other types of rice were long and slender. The correlations among the physical and cooking attributes of tested rice were described by principal component analysis. For physical properties, PC1 was mostly related with hectoliter, broken ratio and moisture content while PC2 was mostly concerned with hardness and true density while for cooking properties. In PC1 (88.5%), the variables spring value and hardness after cooking properties were positively correlated whereas the viscosity and hardness before cooking properties were negatively correlated. The second principal component PC2 (9.3%), showed a positive correlation with spring value, hardness before cooking and gel consistency properties while negatively correlated with hardness after cooking and viscosity.