1. Introduction
Korea has become an aging society, with the population aged 65 and over surpassing 14% of the total population as of 2017. This demographic shift is accompanied by an accelerated incidence of various adult diseases. In particular, calcium levels tend to decline with age and menopause in women, compared to men, leading to an increased incidence of osteoporosis. This condition is becoming more prevalent in younger generations due to excessive dieting among women (Hodges et al., 1993; Iwamoto, 2000; Jeong, 2017; Kasper et al., 2013). Vitamin K plays a crucial role in promoting the gamma-carboxylation of osteocalcin in bones, which inhibits bone resorption by osteoclasts and fosters bone formation by osteoblasts. In particular, vitamin K has a beneficial impact on maintaining calcium balance, a vital aspect of bone metabolism, thus underscoring the importance of consuming vitamin K in conjunction with calcium (Kanai et al., 1997; Price, 1985). As a fat-soluble vitamin, vitamin K is essential for reducing osteoporosis through its roles in blood coagulation and bone metabolism, as well as aiding in protein synthesis and decreasing arteriosclerosis (Institute of Medicine, 2001). Naturally occurring vitamin K mainly exists as K1 (phylloquinone), predominantly found in plants, and K2 (menaquinone), synthesized by bacteria. Vitamin K2 is further classified as menaquinone-n, based on the number of prenyl units in its side chain, and is especially abundant in animal-based foods.
Evaluating the nutrient intake from major foods consumed by the public is crucial for enhancing dietary habits and formulating national health and nutrition policies. Korea, surrounded by the sea on three sides, has a wealth of fishery resources available year-round. With a per capita seafood consumption of 57.05 kg per year, the country ranks fourth globally in seafood consumption (FAO, 2019). However, the existing Korea Standard Seafood Composition Table 2018, which details the nutritional content of domestic seafood, currently only includes vitamin A (retinol) among the fat-soluble vitamins. Although the Ministry of the Interior and Safety has recently started providing nutritional information on foods commonly consumed in Korea through a public portal, there is a noticeable gap in information on micronutrients in fishery resources. This lack of data limits the utility of the national food nutrient database. In particular, there is an immediate need to establish detailed information on vitamin K in commonly consumed domestic fishery resources and processed products. Presently, such information is predominantly available for agricultural and livestock resources, with a significant deficiency in data on fishery resources.
Korea’s distinct four seasons and the resulting changes in water temperature and environment significantly impact the growth environment of fishery resources. Jeong et al. (1998) reported that in marine ecosystems, the nutrient composition of fish is likely to vary considerably across seasons due to a complex interplay of factors such as fish diet, water temperature, and spawning patterns. While there have been studies on the seasonal variations in the characteristics of fishery resources, the majority have focused on changes in species composition and community distribution (Hwang and Park, 2017; Lee et al., 2000; Lee et al., 2010; Oh et al., 2012; Shin et al., 2016). However, research addressing seasonal changes in the levels of micronutrients in fishery resources is notably scarce.
Among the various fishery resources found in the waters around Korea, the conger eel (Conger myriaster) stands out as a highly nutritious food. It is rich in protein, lipids including ω-3 PUFAs, fat-soluble vitamins, and phospholipids. Recognized as an important edible fish species not only in Korea but also in Japan, it holds significant economic value (Kim et al., 2011; Park, 2022). Despite its nutritional importance, research on C. myriaster has predominantly focused on aspects such as age, growth, diet, feeding ecology, and gonadosomatic index (Correia et al., 2009; Huh and Kwak, 1998; Kim et al., 2011; O’Sullivan et al., 2003; Xavier et al., 2010). Studies on its composition have been limited mainly to its general makeup (Ryu et al., 2009), highlighting a need for more research into its micro-vitamin content.
This study aims to sample C. myriaster, a settled fish species available year-round in the waters near Tongyeong, Korea, on a monthly basis throughout 2021. Our objective is to analyze the changes in vitamin K content relative to seasonal variations and size, as well as to examine the correlations between fat-soluble vitamin K content and seasonal changes in fat content. Furthermore, to guarantee the reliability of the analytical data, the enzymatic extraction and HPLC-FLD quantitative methods used for vitamin K analysis were validated using established method validation indicators. Analytical quality control was also rigorously maintained throughout the entire study period.
2. Materials and methods
The standards for phylloquinone and menaquinone used in the vitamin K assay were obtained from Sigma-Aldrich (St. Louis, MO, USA). Lipase (from Candida myriaster), employed in the extraction process, was also sourced from Sigma-Aldrich. Sodium sulfate anhydrous was purchased from Junsei (Tokyo, Japan), and potassium carbonate anhydrous from Daejung Chemical (Shiheung, Korea). All other reagents and solvents utilized in the analysis were of high-performance liquid chromatography (HPLC) grade.
To validate the accuracy of the vitamin K assay, samples SRM 1869 (infant/adult nutritional formula II) and SRM 1849a (infant/adult nutritional formula I) were acquired from the National Institute of Standards and Technology (NIST, Gaithersburg, MD, USA). Additionally, commercial infant formula (Imperial Dream XO World Class 3, Namyang, Seoul, Korea), used as analytical quality control samples, was purchased from a local supermarket. These samples were stored at −70°C and utilized as quality control samples throughout the entire study period.
C. myriaster, an anadromous fish found in the waters around Tongyeong, Gyeongsangnam-do, Korea, was collected monthly, categorized by size (large and small) from January to December 2021. Each month, C. myriaster was collected a total of 12 times per month and sorted into large (80±5 cm) and small (50±5 cm) sizes. The fishes were then homogenized, with non-edible parts such as head, intestines, skin, and fins removed, following the seafood pre-treatment method presented in FAO/INFOODS. Sampling and pre-treatment processes were performed at the National Fisheries Research and Development Institute (Busan, Korea). The homogenized samples were aliquoted and shipped frozen in ice packs to the analytical laboratory. They were stored at −70°C until the time of analysis.
For the vitamin K assay, we employed a modified method from the Food Code (MFDS, 2023). Initially, 1.00 g of solid sample was weighed into a stoppered extraction tube and sonicated (using a Cole-Parmer 8893 sonicator, Chicago, IL, USA) with tertiary distilled water at approximately 40°C for 5 minutes. Subsequently, 1.0 g of lipase and 5.0 mL of 0.8 M phosphate buffer (pH 7.95) were added, thoroughly mixed, and incubated in a shaking water bath (HB-205SW, Hanbaek Scientific Co., Bucheon, Korea) for 2 hours at 37°C and 150 rpm. Following the reaction, 1.0 g of potassium carbonate anhydrous was added to the tube. Next, 10 mL of an ethanol-methanol mixture (95:5, v/v) and 15 mL of n-hexane were added, vigorously mixed for 10 minutes, and then stored in a dark room to facilitate the separation of the hexane layer. The separated supernatant was filtered through a Pasteur pipette filled with sodium sulfate anhydrous and collected into a 25 mL purification flask. The extraction tube, after complete supernatant collection, was treated with an additional 10 mL of n-hexane, and the purification process was repeated to achieve a total volume of 25 mL in the extract, which was then used for the vitamin K assay.
After extracting 10 mL of the sample and concentrating it under nitrogen, it was redissolved in 1 mL of methanol. This solution was then filtered through a 0.45 μm membrane filter (Advantec Co., Tokyo, Japan) and prepared for HPLC analysis. The HPLC was performed using an Agilent 1200 series system (Agilent, Santa Clara, CA, USA), equipped with a ZORBAX Eclipse XDB-C18 column (5 μm, 4.6×150 mm, Agilent) and connected to a post-column (2.0 mm×50 mm, YMC Co., Ltd., Kyoto, Japan). The analysis utilized a fluorescence detector (Agilent) set to an excitation wavelength of 243 nm and an emission wavelength of 430 nm. The flow rate was maintained at 1.0 mL/min, with a column oven temperature of 35°C and an injection volume of 20 μL. The mobile phase consisted of 3 mM sodium acetate trihydrate, 10 mM zinc chloride, and 5 mM acetic acid in a methanol:dichloromethane mixture at a 9:1 (v:v) ratio.
The crude fat content of C. myriaster was determined using the Soxhlet method with a crude fat extractor (ST 243 SOXTECT™, FOSS Tecator, Hillerod, Denmark), as per the guidelines in the Food Code (MFDS, 2018).
The validation of the vitamin K assay adhered to the single laboratory guidelines set by the Association of Official Agricultural Chemists (AOAC) (2002). Specificity was assessed by comparing the retention times of the target peaks in HPLC chromatograms of both standards (phylloquinone and menaquinone) and samples. Linearity was determined by serially diluting the standard solution (0.001, 0.002, 0.004, 0.008, 0.015, 0.030, 0.060, 0.125, and 0.250 μg/mL) to construct a calibration curve. The limit of detection (LOD) and limit of quantification (LOQ) were calculated by analyzing a blank test solution and applying the standard deviation to the mean value of the signal-to-noise (S/N) ratio, multiplying by 3.3 for LOD and 10 for LOQ, respectively. The method’s accuracy was evaluated using standard reference material SRM 1849a (infant/adult nutritional formula I). Recovery (%) was determined by comparing the certified values from the NIST with the analytical values. Precision was evaluated through repeatability (RSDr), based on five replicates per day, and reproducibility (RSDR), based on five replicates over five days, using the commercial infant formula (Imperial Dream XO, Namyang).
Throughout the 12-month duration of this study, a quality control (QC) chart was maintained to manage the analytical system. Before analyzing the fish samples, 10 analytical values with a standard deviation within 5% were initially selected from the values obtained by replicating with the commercial infant formula (Imperial Dream XO World Class 3, Namyang). Their mean and standard deviation were calculated to establish a baseline. The average value was then set as the reference value on the QC chart. The upper and lower control lines (UCL and LCL), as well as the upper and lower action lines (UAL and LAL), were established using specific calculations to monitor the analytical quality of the fish samples. A QC sample was analyzed alongside each sample to ensure consistent quality control of the vitamin K analysis. If the QC sample value fell outside the control lines, the analyzed sample was excluded from the data.
Statistical analysis was performed using SPSS 22.0 (Statistics Package for the Social Sciences, SPSS Inc., Chicago, IL, USA). This involved calculating means and standard deviations. To identify significant differences among samples from three or more groups, Duncan’s multiple range test was applied at the p<0.05 significance level. For samples from two groups, a t-test was used to test for significant differences. The correlation between vitamin K and crude fat content was analyzed using the Pearson correlation coefficient.
3. Results and discussion
Table 1 presents the results of the linearity, LOD, and LOQ validations for the HPLC-FLD method applied to vitamin K in fish. Linearity verification was achieved by diluting a standard solution containing a mixture of both phylloquinone and menaquinone standards to nine concentrations (0.001-0.250 μg/mL). The linearity correlation coefficient (R2) between vitamin K concentration and HPLC quantification across these nine concentrations was 0.9999, demonstrating the method’s strong linearity. For comparison, Kim et al. (2014) used vitamin K1 standards in the concentration range of 0.0313-2.5075 μg/mL for analyzing eight vegetables. Kim et al. (2022) employed vitamin K1 and K2 standards in the range of 0.0125-0.2 μg/mL for analyzing 30 processed foods. In our study, the selected quantification range of 0.001-0.250 μg/mL was lower, deemed appropriate for quantifying vitamins K1 and K2 in seafood where vitamin K exists at trace levels. The LOD for phylloquinone and menaquinone was 0.017 μg/100 g and 0.021 μg/100 g, respectively, and the LOQ was 0.040 μg/100 g and 0.052 μg/100 g, respectively. These values are over 100-fold lower than those reported by Kim et al. (2022), who analyzed vitamin K in meat using the same HPLC-FLD method, highlighting the high sensitivity of the method employed in this study.
Analytes | Regression equation1) | Correlation coefficient (R2) | LOD2) (μg/100 g) | LOQ3) (μg/100 g) |
---|---|---|---|---|
Phylloquinone | y=508.25x-0.0837 | 0.9999 | 0.017 | 0.040 |
Menaquinone | y=511.58x-0.0927 | 0.9999 | 0.021 | 0.052 |
Fig. 1 illustrates the specificity of the vitamin K assay. In the HPLC chromatogram, the retention times for the peaks analyzed from the vitamin K standard solution and the sample extract were 5.4 minutes for phylloquinone and 8.6 minutes for menaquinone. These peaks demonstrated high resolution without interference from neighboring peaks, ensuring the specific detection of the target components. The precision of the vitamin K assay was assessed in terms of repeatability (RSDr) and reproducibility (RSDR), as shown in Table 2. According to the AOAC guidelines (2022), for an analyte concentration of 10 μg/kg in a sample, the acceptable range for repeatability and reproducibility is a relative standard deviation (RSD) of 15% and 32% or less, respectively. In this study, the repeatability and reproducibility of the method were within the acceptable ranges set by the AOAC, with RSDs ranging from 1.7-2.6% and 4.0-6.4%, respectively.
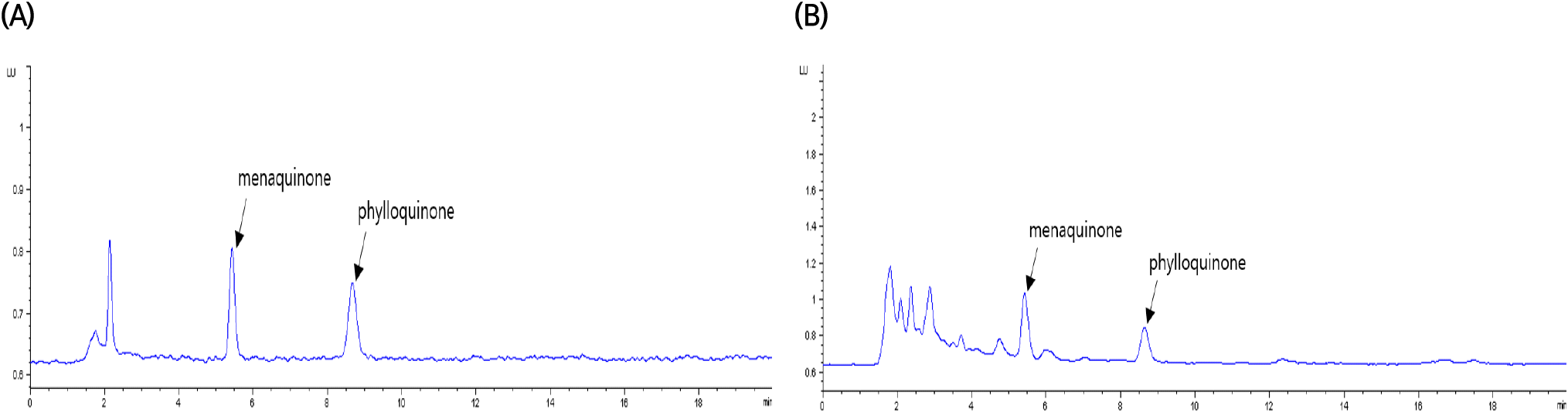
Analytes | Repeatability1) (μg/100 g) | Reproducibility2) (μg/100 g) | ||
---|---|---|---|---|
Mean±SD3) | RSDr4) (%) | Mean±SD | RSDR5) (%) | |
Phylloquinone | 42.43±1.09 | 2.6 | 41.68±1.85 | 4.4 |
Menaquinone | 2.42±0.04 | 1.7 | 2.28±0.16 | 6.9 |
1) Repeatability refers ro the results of independent 5 determinations in triplicates obtained by analyzing a sample five times on the same day.
The accuracy of the vitamin K assay was validated for phylloquinone using the standard reference material SRM 1849a, as shown in Table 3. In this study, using the HPLC-FLD method, the recovery rate for phylloquinone was 88.8%. When compared to the recovery rate of 114.05% achieved by Kim et al. (2022), who also used the same HPLC-FLD method, the discrepancy between the assay value and the true value appeared to be comparable. Both studies demonstrated high accuracy, with recovery rates of 70-125% and 75-120%, respectively. These rates meet the AOAC guideline’s acceptable ranges for accuracy when the analyte concentration in the sample is 10 μg/kg and 1 μg/g, respectively.
Analytes | Sample | Certified value1) (μg/100 g) | Analytical value2) (μg/100 g) | Recovery3) (%) | Recovery limit4) (%) |
---|---|---|---|---|---|
Phylloquinone | SRM 1849a5) | 106±17 | 94.15±3.85 | 88.8 | 75-120 |
The QC for the vitamin K analysis of C. myriaster samples collected throughout 2021 was monitored using a QC chart, as illustrated in Fig. 2. QC charts are valuable for ensuring analytical quality control in studies requiring independent analysis and data production over extended periods. They facilitate monitoring of the validated method’s performance during the sample analysis period and help track changes in various analytical environments that may occur. Fig. 2 displays the results from analyzing the vitamin K content in the infant formula, used as a homogeneous control for each C. myriaster sample. The control lines, based on the mean values of vitamin K in the QC samples (phylloquinone 42.06 μg/100 g and menaquinone 2.35 μg/100 g), were set at 39.08-45.03 μg/100 g for phylloquinone and 2.08-2.62 μg/100 g for menaquinone, incorporating twice the standard deviation. The action lines were established at 37.60-46.52 μg/100 g and 1.95-2.75 μg/100 g, respectively. In the analysis for vitamin K content, the analytical values of the co-analyzed QC samples consistently fell within the control lines. This indicated that the vitamin K analysis of all C. myriaster samples analyzed during the year conformed to the established level of method validation.
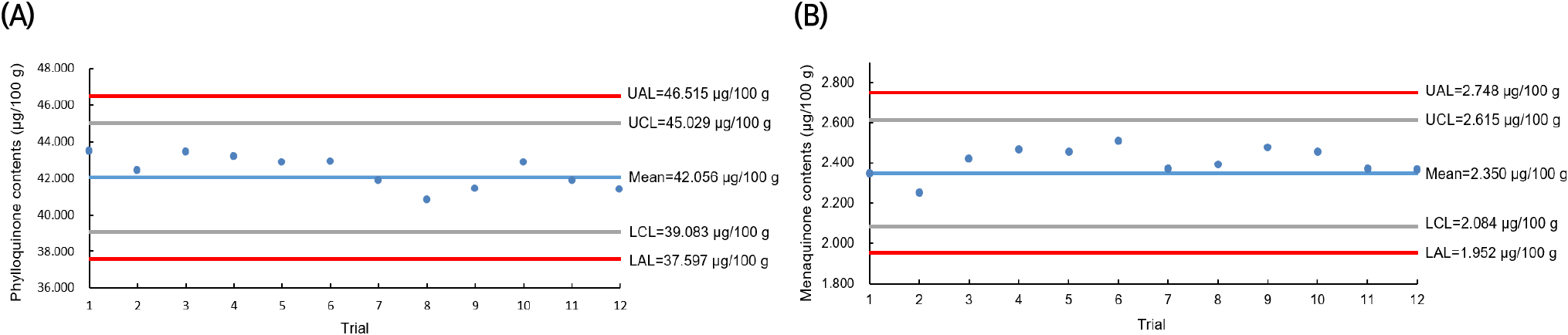
Fig. 3 presents the results of analyzing the vitamin K content in large and small C. myriaster samples collected from the waters of Tongyeong, Gyeongsangnam-do, each month from January to December 2021. The large C. myriaster samples, harvested over the year, showed a range of 0.31-0.80 μg/100 g for phylloquinone, 0.41-0.79 μg/100 g for menaquinone, and 0.71-1.44 μg/100 g for total vitamin K. In contrast, the small C. myriaster samples displayed a range of 0.10-1.94 μg/100 g for phylloquinone, 0.18-0.66 μg/100 g for menaquinone, and a total vitamin K content of 0.33-2.39 μg/100 g. This indicates a substantial monthly variation in vitamin K content, ranging from a 2.5-fold to 18.7-fold difference. Specifically, for phylloquinone, large C. myriaster samples harvested in July (0.80±0.09 μg/100 g) and small samples harvested in December (1.94±0.15 μg/100 g) showed significantly higher vitamin K content compared to other seasons (p<0.05). Menaquinone content peaked in May (0.79±0.11 μg/100 g) for large samples and in January (0.66±0.02 μg/100 g) for small samples. The highest total vitamin K content was observed in July (1.44±0.12 μg/100 g) for large C. myriaster samples and in December (2.39±0.14 μg/100 g) for small samples, highlighting that the differences in monthly content also varied with the size of C. myriaster.
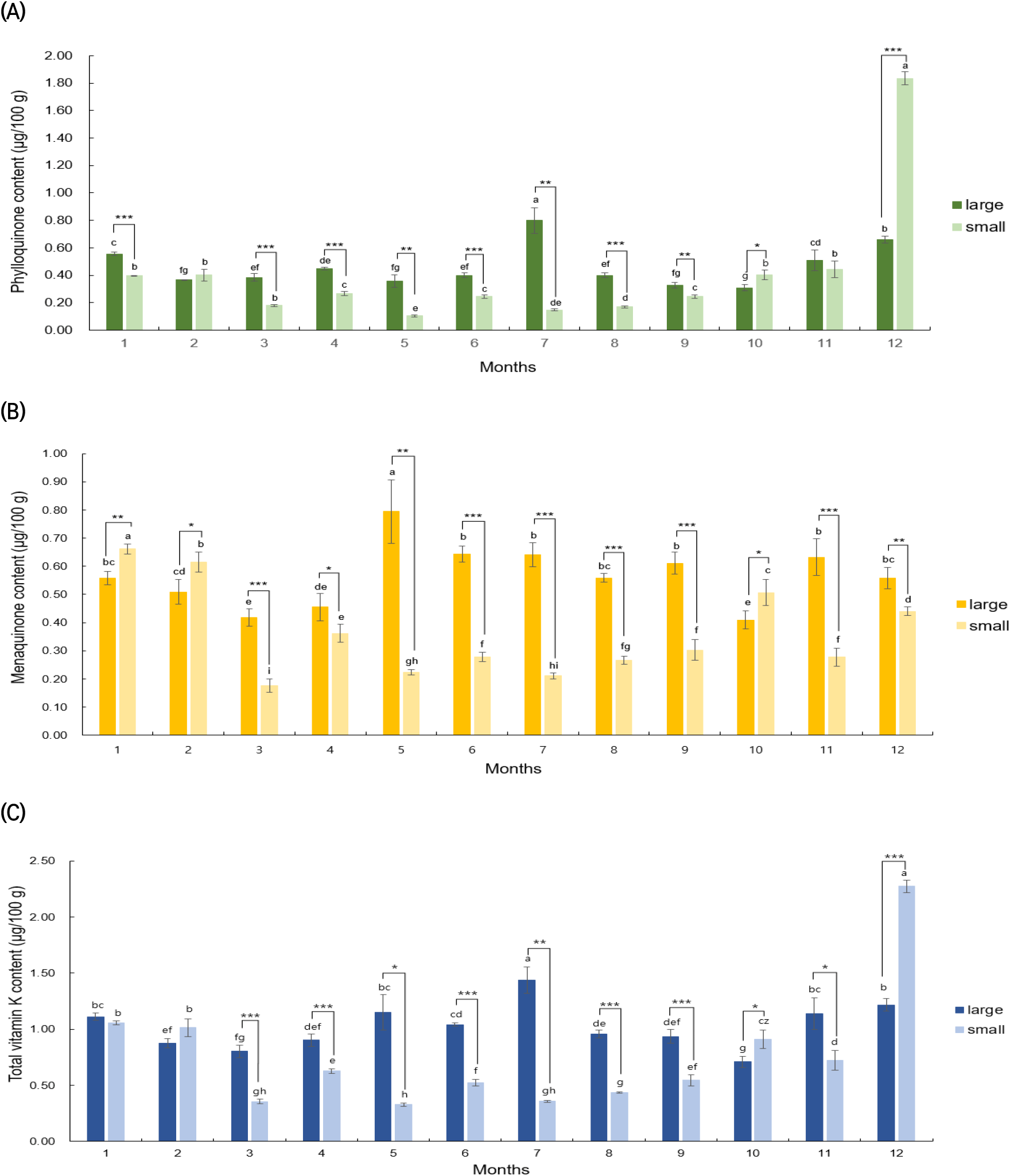
Upon analyzing the significance of differences in vitamin K content in C. myriaster by size for the same harvest time (as shown in Fig. 3), it was found that phylloquinone and total vitamin K exhibited significant differences by size in 10 of the 12 monthly comparisons from January to December. For menaquinone, significant differences were observed in all 12 months. Additionally, when comparing the mean vitamin K content of large and small C. myriaster samples over the entire 12-month period, significant differences were noted in menaquinone and total vitamin K according to sample size, with the exception of phylloquinone. Udagawa et al. (1996) reported that the vitamin K composition in fish tended to mirror that of their prey, indicating that vitamin K content is influenced by the type of prey consumed. In a related study, Kim et al. (2020) investigated changes in stomach content composition with the growth of C. myriaster, identifying anchovies as the most important prey for smaller fish, while fish from the family Gobiidae were more dominant in larger C. myriaster. This suggests a variation in the dominant prey based on the size of C. myriaster. Given these previous findings, the observed differences in vitamin K content among the different size groups of C. myriaster in this study could be attributed to the varying dominant prey species and their subsequent influence on vitamin K content.
Upon analyzing outliers in the phylloquinone content of small C. myriaster samples using the interquartile range (IQR), the December value was identified as an outlier, as it exceeded the maximum range. Consequently, statistical analysis was divided into two parts: one considering 11-month vitamin K content values excluding December (Jan-Nov) and another including the full year with December (Jan-Dec). The results of this significance analysis are detailed in Table 4. Contrary to the December values, which showed no significant size-based difference for phylloquinone, the analysis excluding December revealed highly significant differences (p<0.001) by size for phylloquinone, menaquinone, and total vitamin K. This suggests that the overall vitamin K content in C. myriaster varies by size. When examining the December samples in isolation, there are significant differences in phylloquinone (p <0.001), menaquinone (p<0.01), and total vitamin K (p<0.001) contents between large and small C. myriaster samples, based on the size of the fish. These distinctions are clearly demonstrated in Fig. 3(a), (b), and (c).
Figs. 4 and 5 display the results of a correlation analysis between the monthly changes in crude fat and vitamin K contents in C. myriaster. In large C. myriaster samples, both crude fat and total vitamin K contents peaked in July. Conversely, in small C. myriaster samples, the highest crude fat content was observed in January, while the peak phylloquinone content occurred in December. This suggests that the monthly variation in crude fat and vitamin K is similar across all sizes of C. myriaster. Upon analyzing the correlation between crude fat and vitamin K contents, phylloquinone did not exhibit a significant correlation with crude fat in either large or small C. myriaster samples (p>0.05). However, excluding the December phylloquinone content in small C. myriaster samples, which was deemed an outlier deviating significantly from the overall data range, there was a significant correlation between crude fat and phylloquinone in small C. myriaster samples (p<0.05). Menaquinone displayed a strong positive linear relationship with crude fat (r=0.730, 0.923) across all sizes of C. myriaster, and total vitamin K also showed a clear positive correlation with crude fat in all sizes. This suggests that vitamin K and crude fat are significantly correlated. This finding aligns with the research of Ostermeyer and Schmidt (2001), who observed a positive correlation between vitamin K and fat content, noting that fish with higher fat content had higher levels of vitamin K than those with lower fat content. Factors such as gonadal maturity, water temperature, and feeding influence the fat content of fish, while vitamin K content is known to be affected by fat content, season, feeding, and habitat (Oh et al., 1989; Ostermeyer and Schmidt, 2001; Udagawa and Yamashita, 2008). Thus, these two nutrients in fish appear to share similar influencing factors and are consequently thought to be correlated.
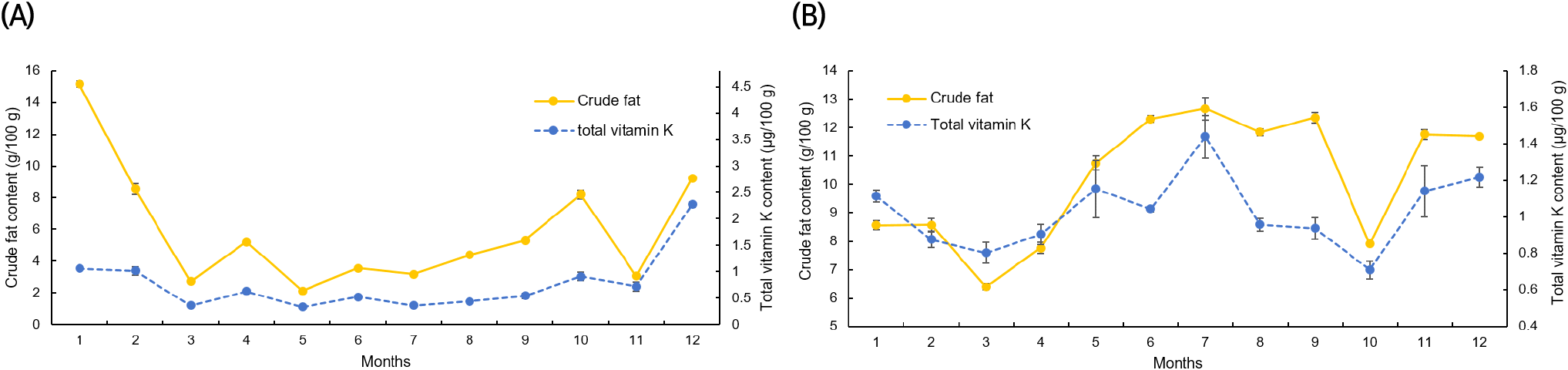
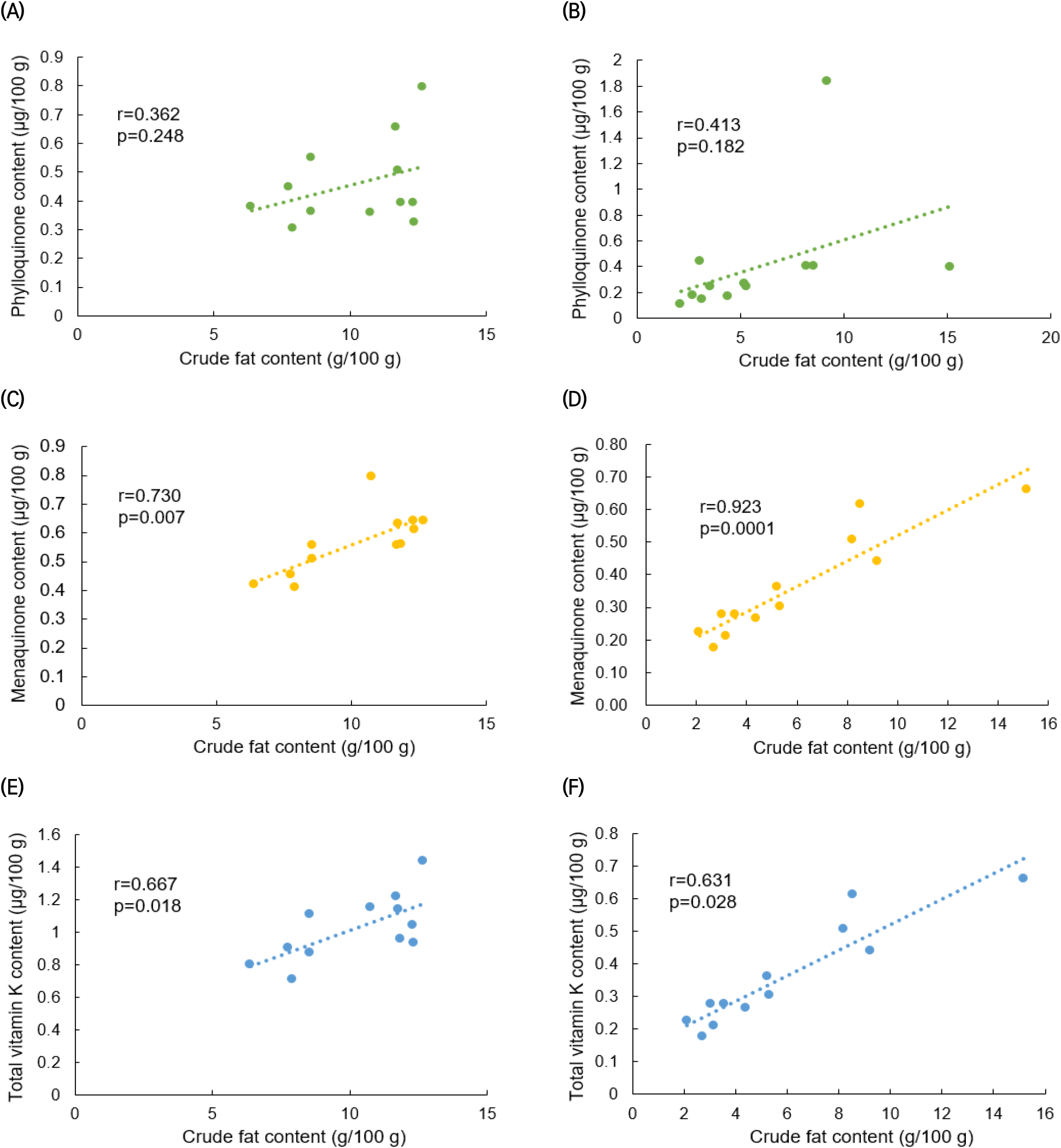
The notably higher phylloquinone content in small C. myriaster samples harvested in December appears to correlate with the peak crude fat content observed in January. Phylloquinone, a prenylated naphthoquinone, is synthesized only by plants, green algae, and certain cyanobacteria species. Unlike these organisms, animals obtain their phylloquinone through the consumption of plants (Basset et al., 2017; Reumann, 2013). Therefore, the phylloquinone content in these fish is likely influenced by their diet, which may include phytoplankton, algae, or plankton-feeding herbivores. The December increase in phylloquinone content in small C. myriaster samples is likely due to an increased dietary intake, while the January rise in crude fat content may be attributed to fat accumulation.
Previous studies have indicated that the nutritional composition of fish is affected by the spawning season (Kim et al., 2009; Lee et al., 1995; Park et al., 1999). According to the National Fisheries Research and Development Institute (2021), the spawning season for C. myriaster is in April-May. However, no significant changes were observed in either vitamin K or crude fat contents during this spawning season. As small C. myriaster individuals are immature and do not engage in spawning, the spawning season seems to have no impact on them. In contrast, large individuals are sedentary fish that do not expend much energy to recover from spawning, thus not necessitating fat accumulation (Jeong et al., 1998). Therefore, the crude fat content in C. myriaster, being a sedentary species, appears to be minimally influenced by the spawning season. Similarly, vitamin K content, which shows a high correlation with fat content, also seems to be largely unaffected by the spawning season.
Vitamin K and crude fat contents in C. myriaster were categorized into spring (March-May), summer (June-August), fall (September-November), and winter (December-February) to determine seasonal variation, as shown in Table 5. Vitamin K content did not exhibit significant seasonal variation across all sizes of C. myriaster. While crude fat was significantly higher in winter, there were no notable changes in spring, summer, or fall, making it difficult to determine a clear pattern of seasonal variation. Comparing these findings with water temperature changes in the Geojedo waters near Tongyeong, as provided by the Korea Hydrographic and Oceanographic Agency (KHOA), it was observed that water temperatures were higher in summer (26.7±3.1°C) and fall (23.7±3.0°C), and lower in spring (16.4±1.8°C) and winter (14.4±1.0°C), indicating significant seasonal differences. However, vitamin K and crude fat contents in C. myriaster did not show significant seasonal variations, suggesting no substantial association with water temperature.
The escalating issue ofglobal warming, marked by rising temperatures, is inducing various alterations in marine ecosystems. These changes include shifts in habitat, food chain structure, and the dominant species of marine organisms. Consequently, there are transformations in the quantity, size, and location of fishing grounds (Doney et al., 2012). In the context of this accelerating global warming, studies like the current one, focusing on the size and seasonal variations in micronutrient content of C. myriaster, are expected to become increasingly significant. They will serve as a foundational basis for future research on the impacts of climate change on marine life.
In addition, this study is notable for being the first to provide reliable information on the vitamin K content of fishery resources that are consumed year-round in Korea. This has been achieved through rigorous method validation and analytical QC. The findings from this analysis can be instrumental in the development and enhancement of a national food nutrient database.
4. Conclusions
In this study, C. myriaster, a sedentary fish species available year-round in Korean waters, was sampled monthly over a year and sorted by size to analyze seasonal changes in vitamin K content and its correlation with fat content. Method validation and analytical QC were rigorously conducted to ensure data reliability over the extended analysis period. The HPLC-FLD method’s analytical performance indicators, including specificity, linearity, LOD, limit of quantitation, precision, and accuracy, were evaluated. All parameters met the acceptance ranges of the AOAC guidelines, confirming the method’s efficacy for analyzing vitamin K content in C. myriaster. QC chart management throughout the study affirmed the reliability of all sample analyses to the level of method validation. The analysis of vitamin K content in C. myriaster by size and month revealed that phylloquinone content peaked in July (0.80±0.09 μg/100 g) for large samples and in December (1.94±0.15 μg/100 g) for small samples. Similarly, menaquinone content was highest in May (0.79±0.11 μg/100 g) for large samples and in January (0.66±0.02 μg/100 g) for small samples. Total vitamin K was also higher in July (1.44±0.12 μg/100 g) and December (2.39±0.14 μg/100 g) for large and small samples, respectively, indicating that monthly content variations depended on the size of C. myriaster. Moreover, a significant difference in vitamin K content between sizes at the same harvest time was observed (p<0.05). The correlation analysis between seasonal and size-specific crude fat and vitamin K content in C. myriaster showed that crude fat content was significantly correlated with menaquinone and total vitamin K contents (p<0.05). This study provides reliable information on the size and seasonal variation of vitamin K in C. myriaster, which is consumed year-round in Korea. These findings can contribute to the national food nutrition database and aid in shaping future national health and nutrition policies.