1. Introduction
The rise in single-person households, dual-income families, and an aging population has fueled a growing demand for convenience foods, leading to the development of processed options such as home meal replacement (HMR) products (Choi et al., 2020). The COVID-19 pandemic further accelerated this trend by reducing dining out expenses and increasing the consumption of processed foods, thereby cementing HMR’s role in contemporary food culture (Seo and Jeong, 2022). A significant challenge in processing vegetables for HMR is the presence of enzymes like peroxidase and polyphenol oxidase, which hinder the storage and processing of fresh vegetables, necessitating specific pretreatments (Hwang et al., 2019).
To augment the commercial appeal and maintain the quality of foods, the food industry commonly employs pretreatment methods such as heating and freezing. In the absence of such pretreatments, browning, decomposition due to microbial growth, and acidification can significantly degrade food quality (Kim et al., 2014). Blanching, a widely used pretreatment process for vegetables, plays a crucial role in maintaining vegetable texture stability, reducing food quality variation by deactivating specific enzymes, preventing browning, and offering a sterilization effect, thus establishing itself as a key food pretreatment technique (Jin and Choi 2001; Sim et al., 2016). While heating during pretreatment may lead to the destruction of unstable nutrients, it can also enhance the bioavailability of certain bioactive substances through facilitated hydrolysis, thereby offering nutritional benefits (Hwang and Nhuan, 2015). Furthermore, heating has been recognized for its inhibitory effects on microbial proliferation, contributing positively to food safety (Den et al., 2018).
Pak choi, scientifically known as Brassica rapa L. ssp. chinensis from the Cruciferae family, has gained prominence as a daily vegetable, particularly in light of the increasing consumption of leafy vegetables. Cultivated annually in greenhouses, pak choi is noted for its rich content of minerals, vitamins A and C, β-carotene, calcium, and high levels of chlorophyll and carotenoids. These components contribute to a range of bioactivities, from inhibiting microbial growth to enhancing liver function and providing protective effects against skin aging and cardiovascular symptoms (Kwon et al., 2019).
However, vegetables such as pak choi necessitate precise processing methods to minimize nutrient loss and physical deterioration during heat treatment (Arroqui et al., 2001). Previous research has encompassed various aspects of leaf vegetable processing, including the microbial decontamination of cabbage leaves using citric acid and ethanol washing (Han and Yang, 2019), morphological analysis of pak choi under salt stress (Memon et al., 2010), the impact of cooking methods on the mineral content of leaf vegetables (Cha and Oh, 1996), the effects of different pretreatment methods on the hot air drying of vegetables (Yoon et al., 1997), and the vitamin content in vegetables pre- and post-blanched state (Kim et al., 2016).
However, a gap in the literature is evident as most of these studies do not differentiate between the various parts of the vegetables when examining their quality characteristics. In particular, there is a scarcity of research focused on the effects of pretreatment on different parts of pak choi, underscoring a need for more detailed investigation in this area.
In this study, the concept of true retention (TR), as defined by the United States Department of Agriculture (USDA), was employed to quantify changes in the content of functional ingredients in pak choi following various pretreatments. TR is calculated based on the alterations in the content and weight of functional ingredients pre- and post-treatment in cooked foods (Lee et al., 2018). Although previous studies have explored the impact of different blanching conditions on the quality characteristics of various vegetables, such as Korean thistle (Cirsium setidens) with varying salt contents (Park et al., 2015), the effects of blanching time on the physico-chemical and sensory properties of Chamchwi (Doellingeria scabra) (Beom et al., 2015), and the changes in physicochemical properties of burdock, lotus root, and garlic stem (Hwang et al., 2019), the application of TR as a measure has been relatively limited. This study aimed to fill this gap by isolating the stems and leaves of pak choi and comparatively analyzing their quality characteristics and antioxidant activities under various pretreatment conditions, with a focus on TR levels. The intent was to provide foundational data on pretreatment conditions and techniques for the advancement of processed foods.
2. Materials and methods
For the study, pak choi samples were purchased in August 2023 from a joint market of the National Agricultural Cooperative Federation in Yesan-gun, Chungcheongnam-do, Korea. Anhydrous citric acid was obtained from Jungbunzlauer, Pernhofen, Austria, and purchased online from Cheiljedang Co., Ltd. (www.cj.co.kr).
In this study, pretreatment of pak choi was conducted in a 70% dark room, following the experimental conditions outlined by Choi et al. (2021) as detailed in Table 1. Initially, pak choi samples were washed twice, and then the stems and leaves were separated and cut into 2×2 cm2 squares. The control group (CON) consisted of untreated pak choi, while the three pretreatment groups were subjected to different blanching methods: water blanched (WB), 2% citric acid blanched (CB), and 2% NaCl blanched (NB). The pak choi samples (both leaves and stems) were added to water at five times their weight and blanched at 100°C for 30 sec and 2 min, respectively. Subsequently, the pretreated samples were cooled at room temperature for 30 min, after which their quality characteristics and antioxidant activities were assessed.
Post-cooling, the pak choi samples from different pretreatment groups were weighed, and the heating loss was calculated as a percentage, representing the difference between pre- and post-heating weights. A water analyzer (MJ-33, Mettler Toledo, Zurich, Switzerland) set at 105°C was utilized to measure the water content of thinly sliced pretreated pak choi samples, with the average and standard deviation derived from five repeated measurements.
For pH analysis, 5 g of pak choi was mixed with 45 mL of distilled water, vortexed, and filtered. The pH of the filtrate was measured five times using a pH meter (Corning 340, Mettler Toledo), and the mean and standard deviation were recorded.
The color of the pak choi’s inner and outer surfaces was analyzed using a colorimeter (CR-300, Minolta Co., Osaka, Japan). The color values were expressed in terms of L (lightness), a (+redness/−greenness), and b (+yellowness/−blueness), with the standard plate values being L=94.65, a=−0.43, and b=4.12.
For antioxidant activity analysis, 10 g of the sample was combined with 90 mL of 70% ethanol. This mixture was then subjected to a 24 h extraction period in a shaking incubator (SI-900R, Jeio Tech, Kimpo, Korea) at room temperature, followed by filtration. The filtrate obtained was used as the test solution.
To accurately measure the total polyphenol contents (TPC) in pak choi, the study implemented the methods established by Swain and Hillis (1959). This process involved creating a mixture consisting of 100 μL of the test solution, 200 μL of 2N Folin Ciocalteu reagent, and 2,000 μL of distilled water. The mixture was vortexed and left to stand in a dark room for 3 min. Subsequently, 300 μL of 1N sodium carbonate (Na2CO3) was added to the mixture, which was then allowed to react for 60 min in the dark. The absorbance of the resultant solution was measured at 725 nm using a spectrometer (DU-800, Beckman Coulter Inc., Seoul, Korea). TPC was quantified in terms of gallic acid equivalents (mg GAE/100 g), based on a calibration curve derived from gallic acid. This measurement was repeated five times to ensure accuracy, and both the mean and standard deviation were obtained.
For the total flavonoid contents (TFC) measurement, the study followed the modified Davis method as adapted by Um and Kim (2007). This method involved mixing 1 mL of the test solution with 10 mL of 90% diethylene glycol and 1 mL of 1 N NaOH. The mixture was then incubated for 60 min in a 37°C water bath (SB-1200, Eyela, Siheung, Korea). The absorbance of the mixture was measured at 420 nm using a spectrometer. TFC was calculated in quercetin equivalents (mg QE/100 g), using a calibration curve based on quercetin. This procedure was also conducted five times, with the mean and standard deviation calculated for precision.
To assess the DPPH (1,1-diphenyl-2-picrylhydrazyl) radical scavenging activity, the methodology described by Lee et al. (2007) was utilized. This involved adding 1 mL of DPPH solution (1.5×10−4 M) to 4 mL of the test solution, vortexing the mixture, and then allowing it to stand in a dark room for 30 min. The absorbance was subsequently measured at 517 nm. Ethanol was used as a control in place of the test solution. The scavenging activity was calculated using a specific formula, and, as with the other tests, this measurement was repeated five times to obtain both the mean and standard deviation.
To evaluate the ABTS+ (2,2’-azino-bis(3-ethylbenzothiazoline-6-sulfonic acid) radical scavenging activity in pak choi, the study implemented the methods described by Siddhuraju and Becker (2007). This involved preparing a mixture of 7.0 mM ABTS+ solution and 2.45 mM potassium persulfate solution, which was left in a dark room for a 24 h reaction. The resulting solution containing ABTS+ radicals was then diluted with ethanol to achieve an absorbance of 0.70±0.02 at 734 nm. The prepared ABTS+ solution was subsequently used for the ABTS+ scavenging activity analysis. The absorbance of a mixture consisting of 900 μL of ABTS+ solution and 100 μL of the test solution was measured at 734 nm at 1-min intervals for 6 min. Ethanol was used as the control in place of the test solution. This process was repeated five times, with the mean and standard deviation recorded for each measurement.
After the pretreated pak choi samples were cooled at room temperature for 30 min, the TPC and TFC both before and after pretreatment were calculated and expressed as percentages.
where A denotes the functional ingredient content per 100 g of pak choi after blanching and B denotes the functional ingredient content per 100 g of pak choi before blanching.
The TR of TPC and TFC per 100 g of pak choi, pre- and post-treatment, was also calculated and expressed as a percentage.
where A denotes the functional content per 100 g of pak choi after blanching, B denotes the weight (g) of pak choi after blanching, C denotes the functional content per 100 g of pak choi before blanching, and D denotes the weight (g) of pak choi before blanching.
For microbial analysis, the study followed the methods outlined by Kang et al. (2013). This involved homogenizing 10 g of pak choi in 90 mL of sterile physiological saline (0.85%) using a stomacher (HG400V, Mayo International SR., MI, Italy). The homogenate was then serially diluted with sterile physiological saline. 1 mL of the diluted solution was added to 3M Petrifilm medium (3M Co., St. Paul, MN, USA) and incubated at 36±1°C for 48 h. The number of colonies formed was counted, and the total microbial count was expressed in log CFU/g.
All collected data in this study were statistically analyzed using SPSS 25.0 (Statistical Package for Social, SPSS Inc., Chicago, IL, USA). The results were expressed as mean and standard deviation. To determine the significance of observed differences, one-way analysis of variance (ANOVA) was conducted, followed by Duncan’s multiple range test, with a significance level set at p<0.05.
3. Results and discussion
Table 2 details the heating loss and water content measurements of pak choi. For the stems, the highest heating loss was observed in the S-NB (2% NaCl blanched) group at 15.10% (p<0.001), followed by the S-WB (water blanched) group at 8.99%, and the S-CB (2% citric acid blanched) group at 8.74%. Similarly, the leaves exhibited the highest heating loss in the L-NB group at 11.44%, with lower values in L-WB (−3.10%) and L-CB (−4.19%). Both the stem and leaf groups demonstrated significant differences in heating loss (p<0.001). Roult-Wack et al. (1992) reported that the addition of salt in a colloidal solution could draw out water, leading to sample dehydration, which aligns with the highest heating loss observed in the NB groups.
1) CON, non-treatment; WB, blanching with water; NB, blanching with 2% NaCl; CB, blanching with 2% citric acid. Stems and leaves were blanched during 2 min and 30 sec with each treatment method, respectively.
Regarding water content, the stems had percentages of 94.50% in S-CB, 93.86% in S-WB, 93.79% in S-CON (control), and 93.53% in S-NB. The leaves showed water content percentages of 88.86% in L-CB, 88.62% in L-CON, 88.40% in L-WB, and 87.90% in L-NB. No significant difference in water content was found between the stem and leaf groups (p>0.05). This contrasts with Hwang et al. (2019), who reported lower water content in root vegetables like burdock and lotus roots after blanching compared to controls. According to Molina et al. (2011), high-temperature pretreatment can cause water loss due to cellular tissue damage. Conversely, Choi et al. (2021) found that increasing blanching time led to higher water content in leafy vegetables as tissues reabsorbed water. Similarly, Lee et al. (2015) reported no significant difference in water content between control and pretreated butterbur stems, in agreement with the current study’s findings.
Table 3 presents the pH measurements of pak choi. The pH ranged from 3.69 to 6.01 for the stems and 3.50 to 6.22 for the leaves, with the lowest pH observed in the CB groups due to the acidic nature of citric acid. This is consistent with findings by Choi et al. (2021) and Han and Yang (2019), where the lowest pH values were also recorded in groups with added citric acid.
Table 3 also includes color measurements of pak choi following different pretreatment conditions. For pak choi stems, the lightness of color (L value) varied significantly across the groups: it was highest in the control group (S-CON) at 66.83 and lowest in the NaCl blanched group (S-NB) at 55.08, citric acid blanched (S-CB) and water blanched (S-WB) groups showing intermediate values of 57.10 and 56.01, respectively. This indicates a significant reduction in lightness in all pretreatment groups compared to the control (p<0.05).
The a value, indicating redness/greenness, was most negative in S-NB at −11.33 and least negative in S-CB at −3.81, with S-CON and S-WB having intermediate values. This demonstrates a trend towards increased greenness in the pretreatment groups compared to the control (p<0.05). In terms of yellowness/blueness (b value), the range spanned from 14.19 in S-CON to 20.14 in S-NB, suggesting an increase in yellowness following pretreatment (p<0.05). For pak choi leaves, similar trends were observed. The L value was significantly lower in all pretreatment groups (L-WB, L-CB, L-NB) compared to the control (L-CON), indicating darker colors post-treatment. The a value was most negative in L-NB at −11.76 and least negative in L-CB at −2.34, suggesting a trend towards increased greenness in the pretreated leaves. The b value varied from 11.06 in L-WB to 14.91 in L-CB, indicating variable changes in yellowness/blueness across the different pretreatments (p<0.05).
These results highlight the significant impact of different pretreatment methods on the color attributes of pak choi, both in stems and leaves, which is crucial for consumer appeal in processed foods.
In this study, the color difference (ΔE) in the Hunter color system was analyzed to assess the impact of various pretreatments on pak choi. According to Kim et al. (2009), ΔE values in the range of 6.0 to 12.0 and 3.0 to 6.0 indicate ‘very large’ and ‘large’ variations in color, respectively.
For pak choi stems, compared to the control group, the a value, which represents redness/greenness of the stem pretreated with water (S-WB) and salt (S-NB) decreased by about 2.52-4.37, and the a value pretreated with citric acid (S-CB) increased by about 3.15. The b value, indicating yellowness/blueness, increased by approximately 3.52 to 5.93 in the pretreated stems relative to the control. These changes suggest a ‘very large’ variation in color, with an increase in both greenness and yellowness for the stems pretreated with water and salt, and an increase in redness and yellowness for those treated with citric acid.
In the case of the leaves, the a value increased marginally in the groups treated with water (L-WB) and salt (L-NB). In the citric acid-treated group (L-CB), both a and b values increased by approximately 7 and 3, respectively. This indicates a ‘very large’ variation in color, with increases in redness and yellowness in the leaves following citric acid pretreatment.
The high chlorophyll content in pak choi, as reported by Kwon et al. (2019), and the changes in vegetable color due to alterations in chlorophyll pigments with varying cooking temperatures and times, as noted by Kim et al. (2022), are relevant to these findings. Ngo and Zhao (2007) reported that chlorophyll pigments form olive green pheophytin upon treatment with weak acid or heating at ≥60°C, while brown pheophorbide is generated with continuous acid treatment. The current study also indicates that pretreatment temperature and the presence of acid affect the L, a, and b values. Therefore, it can be inferred that the yellowness in pak choi is likely altered by the addition of citric acid and the greenness by the addition of salt, reflecting changes in the chlorophyll pigments.
Table 4 details the TPC and TFC for pak choi stems and leaves, along with their respective percentage variations and TR following different pretreatments. In pak choi stems, the TPC was highest in the NaCl blanched group (S-NB), ranging between 35.69 and 43.61 mg GAE/100 g. However, this was not significantly different from other pretreatment groups (p<0.05). Notably, both the percentage variation and TR in TPC were highest in the S-NB group (p<0.01). Similarly, TFC was highest in the S-NB group, with values ranging from 34.71 to 70.43 mg QE/100g (p<0.001). The S-NB group also exhibited the highest percentage variation and TR in TFC (p<0.001). Apart from the S-NB group, all other pretreatment groups showed reduced TFC compared to the control.
3) CON, non-treatment; WB, blanching with water; NB, blanching with 2% NaCl; CB, blanching with 2% citric acid. Stems and leaves were blanched during 2 min and 30 sec with each treatment method, respectively.
Previous research, such as that by Hong and Ahn (2005) and Cha et al. (2023), noted a decrease in TFC and TPC in leafy vegetables like spinach and water spinach after blanching. However, studies like Yoon et al. (1998) and Park et al. (2015) have shown that NaCl pretreatment can enhance phenolic compounds in vegetables, indicating a protective effect of NaCl on bioactive substances.
In pak choi leaves, the highest TPC was observed in the water blanched group (L-WB), ranging from 146.25 to 209.58 mg GAE/100 g (p<0.001). This group also had the highest TPC percentage variation and TR (p<0.01). Likewise, the TFC was highest in L-WB, with values between 403.76 and 884.71 mg GAE/100 g (p<0.001). Both the percentage variation and TR in TFC were also highest in the L-WB group (p<0.01). Unlike the stems, the leaves showed higher TFC values in the L-WB group compared to the L-NB group.
In the context of the study, various research findings on the effect of salt stress on plants are pertinent. Choi and Chiang (2015) observed that plants produce antioxidants and enzymes to mitigate the effects of Reactive Oxygen Species (ROS) under salt stress. However, Kim and Hyun (2011) noted that this response varies among different plant species. In an examination of the physiological response to NaCl stress in three potato species, Daneshmand et al. (2010) found that two species showed a decrease in phenolic compounds with increasing NaCl levels. They also reported that TFC decreased with added NaCl, indicating that antioxidant levels might increase under optimal salinity but decrease under higher salinity conditions.
Moreover, Navarro et al. (2006) found that phenolic compounds in chili decreased as salinity increased. In line with this, Kim et al. (2021) observed the lowest TFC in salt-treated samples of Apocynum lancifolium. These findings suggest that an increase in salinity can lead to a reduction in bioactive substances in plants. Applying these insights to the current study, it appears that the pak choi leaves might have experienced a more significant reduction in bioactive substances compared to the stems due to a relatively higher salt concentration in the pretreatment.
Consequently, the study concludes that more pronounced levels of TPC and TFC were found in salt-treated stem groups and water-treated leaf groups, underscoring the differentiated response of pak choi stems and leaves to various pretreatment conditions.
Fig. 1 displays the results of the DPPH and ABTS+ radical scavenging activity assays for pak choi stems and leaves subjected to different pretreatments. In the stems, the DPPH radical scavenging activity was observed to be highest in the control group (S-CON) at 90.12%, followed by the NaCl blanched (S-NB) at 87.83%, the water blanched (S-WB) at 87.11%, and the citric acid blanched (S-CB) at 85.54%. Notably, S-NB demonstrated the highest scavenging activity among the pretreated groups, whereas S-CB exhibited the lowest (p<0.001). Similarly, ABTS+ radical scavenging activity was highest in S-CON at 42.47%, with a subsequent decrease in S-NB (37.80%), S-WB (32.96%), and S-CB (29.67%) (p<0.001). These results indicate a general decrease in radical scavenging activity across all pretreated groups compared to the control.
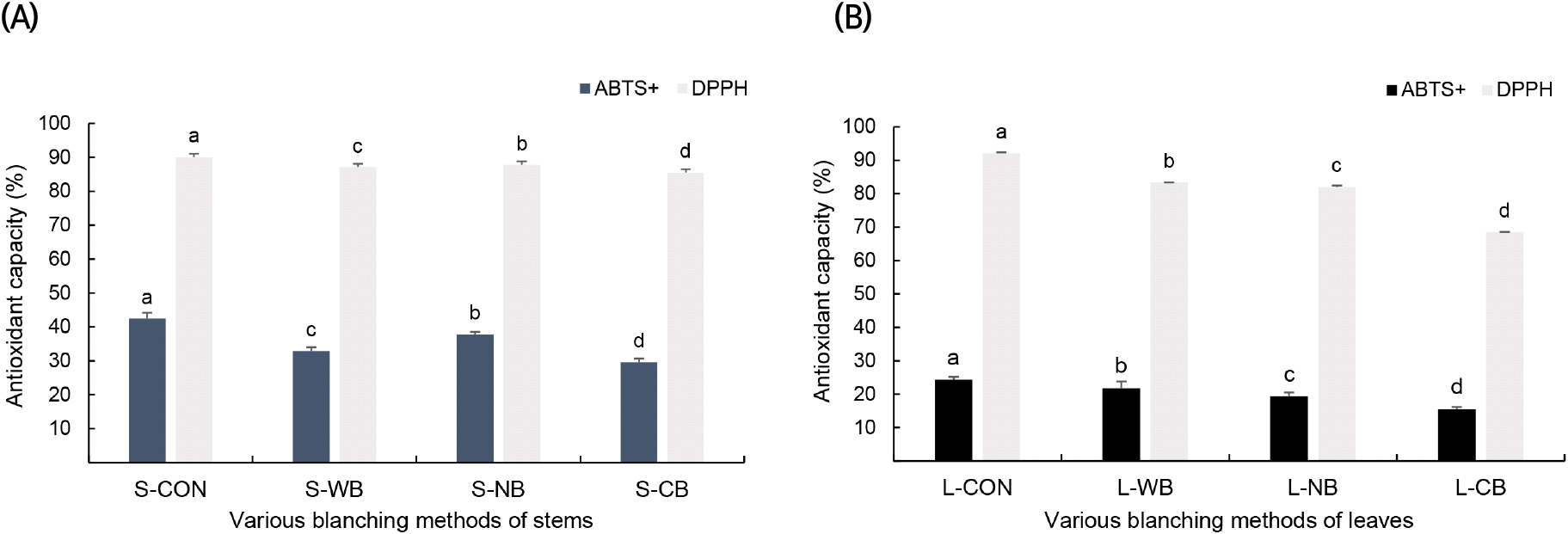
The elevated radical scavenging activity in S-NB is likely linked to the distribution of TPC and TFC, as delineated in Table 4. Previous studies have established a correlation between the increase in phenolic compound contents and the enhancement of DPPH and ABTS+ radical scavenging activities (Kim et al., 2019). Factors influencing antioxidant activity in vegetables, such as the type and structure of bioactive substances, cooking temperature, cutting style, and the presence of synergistic substances, have been identified (Jiménez-Monrea et al., 2009). The relationship between polyphenols and antioxidant activity is well-documented, with increased polyphenol content potentially contributing to enhanced DPPH and ABTS+ activities. This observation aligns with the findings of Park et al. (2015), who reported an increase in phenolic content in vegetables blanched with NaCl.
In pak choi leaves, the highest DPPH radical scavenging activity was recorded in the control group (L-CON) at 92.17%, followed by the water blanched (L-WB) at 83.37%, the citric acid blanched (L-CB) at 82.05%, and another citric acid blanched group (L-CB) at 68.55% (p<0.001). For ABTS+ activity, L-CON showed the highest activity at 24.31%, followed by L-WB (21.83%), L-NB (19.35%), and L-CB (15.47%). Among the pretreatment groups, L-WB exhibited the highest radical scavenging activity, while L-CB showed the lowest (p<0.001). The superior radical scavenging performance in L-WB could be attributed to its TPC and TFC levels, along with the potential influence of excessive salt stress on antioxidant activity.
The pretreatment methods applied in this study demonstrated effectiveness in minimizing the loss of antioxidant activity in pak choi. Specifically, a 2% NaCl solution was used for the stems and a lower concentration of NaCl for the leaves.
Table 5 details the total microbial counts in pak choi stems and leaves. The microbial counts in the control groups were 2.57 log CFU/g for the stems (S-CON) and 2.87 log CFU/g for the leaves (L-CON). In contrast, the pretreated groups showed no detectable microbial presence. This observation aligns with the findings of Bae et al. (2003), who noted the suppression of microbial growth and biochemical activity in food through pretreatment processes. Similarly, Choi et al. (2022) reported a complete absence of microbial growth in pretreated carrot samples, while the untreated control exhibited a count of 3.37 log CFU/g. These results suggest the sterilizing effect of a brief pretreatment, whether with NaCl or citric acid, on both pak choi stems and leaves.
Samples | Total microbial counts (log CFU/g) | |||
---|---|---|---|---|
CON1) | WB | CB | NB | |
Stems | 2.57 | ND2) | ND | ND |
Leaves | 2.87 | ND | ND | ND |
4. Conclusions
In this study, the stems and leaves of pak choi were separately treated and analyzed to determine the impact of various pretreatments on their quality characteristics, with a particular focus on TR. The pretreatments employed included distilled water, 2% NaCl, and 2% citric acid. The highest heating loss was observed in the NaCl-treated stems (S-NB) and leaves (L-NB). Water content in the stems remained within a narrow range of 93.53% to 94.50%, and in the leaves, it ranged from 87.90% to 88.86%, with no significant variation noted across the pretreatment groups for both stems and leaves. The pH levels were found to be the lowest in both stems and leaves following citric acid pretreatment. Color analyses indicated an increase in yellowness with citric acid pretreatment and an enhancement in greenness with NaCl pretreatment. The TPC, TFC, and TR were highest in the NaCl-treated stems (S-NB) and in the water-treated leaves (L-WB). Correspondingly, the highest DPPH and ABTS+ scavenging activities were recorded in S-NB for the stems and in L-WB for the leaves. The total microbial count was measured at 2.57 log CFU/g in the stem control group (S-CON) and 2.87 log CFU/g in the leaf control group (L-CON), while no microorganisms were detected in any of the pretreatment groups. These findings are expected to provide essential data for developing pretreatment conditions and processing techniques for HMR containing pak choi. The study concludes that to preserve bioactive substances and prevent microbial proliferation in pak choi, the leaves should be pretreated with water, while the stems should be pretreated with water containing 2% NaCl.