1. Introduction
Camellia oleifera Abel. is known as a plant that provides oil with high medicinal and nutritional value and is distributed in China, India, and Southeast Asia. To cultivate C. oleifera trees, it is essential to select well-drained soil, such as lava soil (schisteux), sand, or sloping soil. These trees are susceptible and unsuitable for growing in waterlogged areas near lakes, ponds, or wetlands. Additionally, they thrive in environments with adequate humidity but should be protected from strong winds (Thang, 2015).
Camellia oleifera is a small evergreen tree, typically reaching a 5-7 m height. Multiple trunks emerge from the base, with branches densely clustered at the top. The leaves are simple, spaced apart, and characterized by serrated edges and thick leaf blades. In terms of size, the leaves measure approximately 3.5-9 cm in length and 1.8-4.2 cm in width, with 6-7 pairs of veins. The flowers of C. oleifera have inverted egg-shaped or spear-shaped petals, measuring around 3-4 cm in length and 2-2.5 cm in width. The pistil is about 12-13 mm long, with a style that is typically divided into 3-4 branches, spreading horizontally about 2 mm from the tip. The base of the style may have a smooth surface or a few hairs. The fruit of this tree is characterized by a hard shell, ranging from 2.1-6.7 cm in diameter and 2.1-6.1 cm in height (Fig. 1). The top of the fruit is either round or slightly pointed, with a thick wall divided into three compartments. Each compartment usually contains 1-3 seeds that are rich in oil (Thang, 2015).
In Vietnam, C. oleifera is distributed in northeastern mountainous provinces, including Lang Son and Quang Ninh. It is considered a wild plant growing in the forest. Indigenous people mainly harvest seeds to export to China for oil pressing. Although oil has been exploited for a long time, knowledge about the chemical component of the oil and other parts of the plant is still quite limited, especially the uses of this plant in medicine and pharmacy, which still have much to discover for scientists. Therefore, this article will overview the above issues to create a premise for future research.
2. Some major food and medical applications of C. oleifera
Camellia oleifera has many uses, but the main product today is cooking oil from seeds. In addition, C. oleifera oil is a traditional drug to treat stomach pain and burning in various Asian regions. Moreover, C. oleifera oil is widely used in functional foods, pharmaceuticals, and cosmetics (Zhang et al., 2022). C. oleifera flowers in October and they last until February of the following year. The fruit develops from February until October and is usually harvested in October (Deng et al., 2020). After being picked, C. oleifera fruit is dried in a well-ventilated place for 4-5 days, during which the fruit naturally splits, allowing the seeds to fall out. These are the main ingredients for oil pressing. The shape of the ripe fruit is spherical; the seeds have a hard black shell and yellow kernel (Zeng and Endo, 2019). The different fruit parts are displayed in Fig. 2.
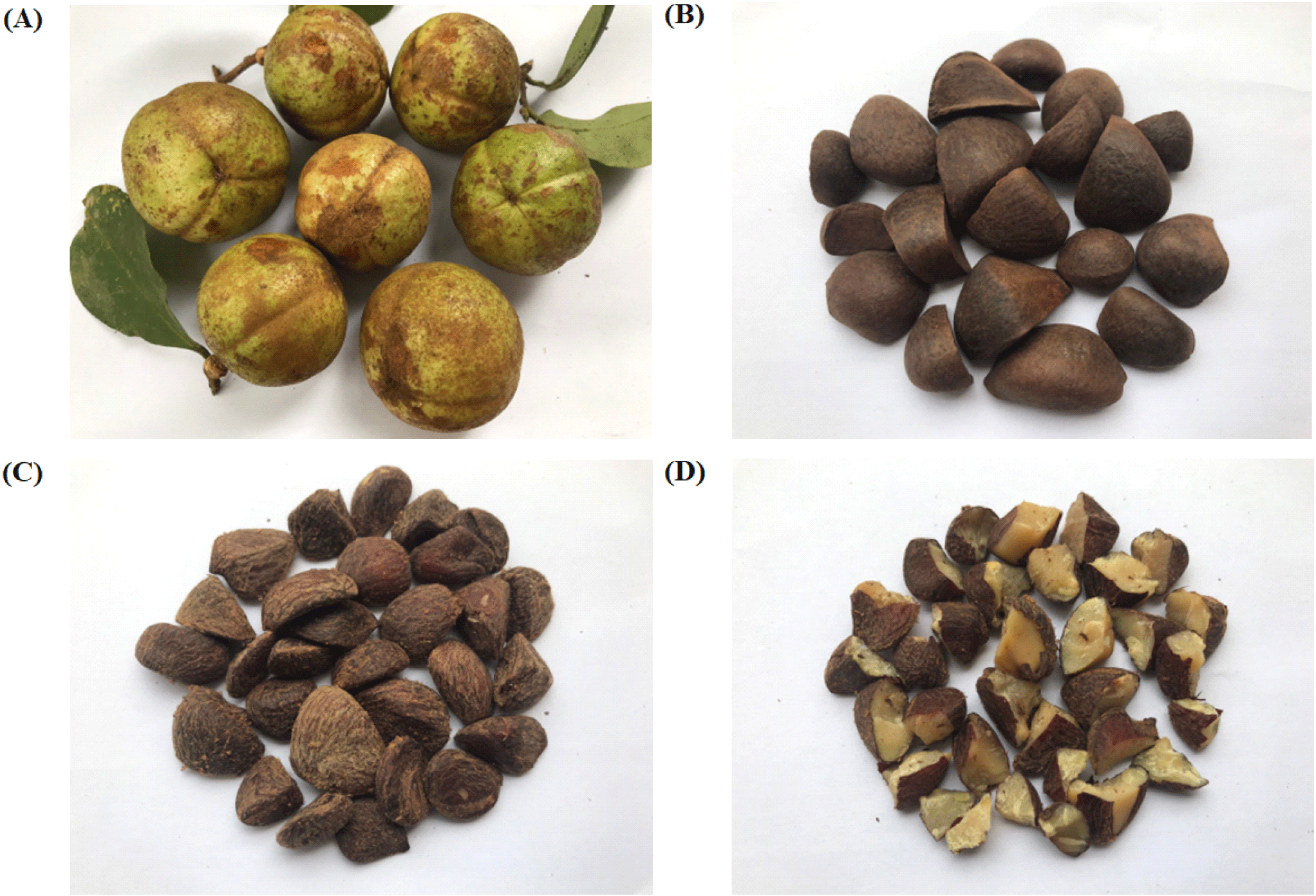
Camellia oleifera oil is a high-quality vegetable cooking oil, rich in unsaturated fatty acids (UFAs, > 85%), especially oleic acid (75.78-81.39%) and omega-6-linoleic acid (4.85-10.79%) (Cheng et al., 2018; Yang et al., 2016). Different parts of the C. oleifera plant serve as ingredients in traditional medicines in China. Nowadays, a total of 249 compounds, including triterpenoid saponins, flavonoids, polyphenols, lignans, polysaccharides, and others, have been extracted from the seeds, peel, seed cake, leaves, flowers, and roots of C. oleifera (Chou et al., 2018; Guo et al., 2020; Zhang et al., 2021). Most of these compounds are notable components with many potential biological properties. Recent findings have shown that some crude extracts, such as monomeric compounds and polysaccharides isolated from the seed cake, peel, and leaves of C. oleifera, have outstanding pharmacological properties, such as anti-cancer (Yan et al., 2016), antioxidant (Lee et al., 2007), anti-inflammatory (Ye et al., 2012), antibacterial (Feás et al., 2013), liver protection, stomach protection (Lee et al., 2007), blood sugar lowering (Zhang and Li, 2018), immunomodulatory, and antihypertensive activities (Chiang et al., 2021), both in vivo and in vitro. Most of these studies’ effects followed those observed for C. oleifera in traditional medicine (Yang et al., 2016).
The oil extraction process from different seed varieties is highly versatile, using various techniques from traditional to contemporary methods such as pressing, thermal, solvent, Soxhlet extraction, supercritical fluid extraction, etc. Each method has different advantages and disadvantages. However, the pressing method still stands out because of its convenience, even though the quality and recovery efficiency may not be as good as other methods (Fig. 3) (Thao et al., 2021). Nowadays, the utilization of C. oleifera oil remains relatively unfamiliar in Asian nations, particularly Vietnam. Consequently, oil consumption and production predominantly occur on a small scale within households with convenient access to raw materials. Therefore, employing the traditional method (pressing method) can enhance production capacities, increasing local citizens’ income. Additionally, on an industrial scale, the preferred extraction method is still hot pressing, which was applied to produce oil from various materials. The advantages and disadvantages of this method are illustrated in Table 1 (Geow et al., 2021). For C. oleifera oil, the seeds are dried for about 7 days to carry out this process until the moisture content drops below 8% and they are then pressed at 140°C. After pressing, the oil is stored in bottles at room temperature (Fang et al., 2015). In certain households equipped for the task, individuals opt to roast the seeds before pressing. Roasting serves the fundamental purpose of achieving uniformity, ensuring the oil maintains consistent quality and color. Following the roasting process, the beans undergo pressing, during which an oil filter separates any remaining residue from the oil. Additionally, high-powered presses are used in certain areas to extract oil from whole seeds. Currently, there are seed shell machines, creating favorable conditions for extraction. For enhanced oil quality, the cold pressing method is often preferred. This approach entails pressing and grinding the seeds at temperatures not surpassing 49°C. By employing cold pressing, the resulting oil preserves the distinct flavor, aroma, and nutritional advantages inherent in the seeds. However, it should be noted that this method has some limitations. For example, cold-pressed oils have a shorter shelf life and are more difficult to use. Due to the short storage time, it is more difficult to use because the oil is not yet cooked. Investment costs are also relatively high (Geow et al., 2021). Therefore, the hot-pressing method is still frequently applied in small-scale oil production in households.
Camellia oleifera is considered a valuable oilseed crop because of its high content of unsaturated fatty acids (UFA, 90%), surpassing that of olive oil (86%) (Guo et al., 2018), while saturated fatty acids (SFA) account for only a small portion of the oil (Quan et al., 2022). Some previous studies have proved the long-term health benefits of C. oleifera oil in preventing and treating cardiovascular and cerebrovascular diseases, reducing the risk of heart disease, lowering cholesterol levels, and protecting the liver (Bumrungpert et al., 2016; Ko et al., 2019; Qiu et al., 2016; Yeh et al., 2020). Additionally, C. oleifera contains various bioactive compounds, such as sterols, squalene, tocopherol, and fatty acids, which have been shown to promote overall health, possess anti-atherosclerotic properties, and exhibit antioxidant effects (Xiao et al., 2017; Zhang et al., 2019). The seed oil of this material is commonly used as a health food and dietary supplement to reduce the risk of cancer, improve digestion, regulate blood cholesterol, and enhance the immune system (Du et al., 2020; Wang et al., 2019).
Table 2 shows that the UFA content of C. oleifera oil accounts for the highest proportion (90%), followed by walnut (87.5%), olive (86%), and coconut oil (8.4%). This demonstrates that this is an excellent food for human health.
Oil1) | C. oleifera (Quan et al., 2022) | Olive (Guo et al., 2018) | Walnut (Moigradean et al., 2013) | Coconut (Moigradean et al., 2013) |
---|---|---|---|---|
UFAs (%) | 90 | 86 | 87.5 | 8.4 |
SFAs (%) | 10 | 14 | 9.5 | 87.2 |
Table 3 shows that C. oleifera and olive oil contain similar fatty acids; perhaps that is why scientists named it the “Oriental olive”. In addition, monounsaturated fats - oleic acid accounts for the highest proportion, with 87.93% for C. oleifera and 72.77% for olive oil. Oleic acid is known for its many health benefits and for regulating many physiological functions. Some studies have also shown its beneficial effects on cancer (Carrillo et al., 2012a), autoimmune and inflammatory disease infections, and its ability to support wound healing (Carrillo et al., 2012b). In addition, this oil also contains omega-6 in a small proportion (Quan et al., 2022). In contrast, Table 3 shows that walnut and coconut oils have deficient oleic acid and not as many fatty acids as C. oleifera and olive oils. Camellia oleifera, olive, and walnut oils have a high percentage of unsaturated fatty acids (>85%), while coconut oil is shallow. Coconut oil has only 8.4% unsaturated fatty acids, mainly oleic acid (5.5%). Lauric acid accounts for the highest portion of the oil content (44.6%) in coconut oil; thus, coconut oil is said to be a primary source of lauric acid, with significant food and non-food utilization (Gervajio, 2013). Compared to other oils (coconut oil, walnut oil, and olive), the UFAs found in C. oleifera oil are also diverse. This shows that C. oleifera oil has a nutritional value equivalent to or even higher than olive oil, where the UFA and oleic acid content account for the highest proportion of the four oils mentioned above. Chaikul et al. (2017) showed that C. oleifera oil contained oleic acid, a major UFA, that inhibits melanogenesis through the inhibition of tyrosinase activity and TRP-2, as well as antioxidant activity. Overall, the nutritional components of C. oleifera oil show that it is a raw oil with much potential, promising to bring high value to the food technology, medical, and cosmetic industries.
Fatty acid composition | C. oleifera oil (%) (Chaikul et al., 2017) |
Olive oil (%) (Guo et al., 2018) |
Coconut oil (%) (Moigradean et al., 2013) |
Walnut oil (%) (Moigradean et al., 2013) |
---|---|---|---|---|
Capric acid (C10:0) | - | - | 7.00 | - |
Lauric acid (C12:0) | - | - | 44.60 | - |
Myristic acid (C14:0) | 0.04 | 0.01 | 20.40 | 0.20 |
Palmitic acid (C16:0) | 5.08 | 12.09 | 11.20 | 6.30 |
Palmitoleic acid (C16:1) | 0.19 | 1.15 | - | - |
Margaric acid (C17:0) | 0.10 | 0.05 | - | - |
Margaroleic (C17:1) | - | 0.10 | - | - |
Stearic acid (C18:0) | 5.14 | 3.01 | 2.60 | 3.00 |
Oleic acid (C18:1) | 87.93 | 72.77 | 5.50 | 24.20 |
Linoleic acid (C18:2) | 0.10 | 9.47 | 1.80 | 54.80 |
Linolenic acid (C18:3) | - | 0.60 | 1.10 | 8.50 |
Arachidic acid (C20:0) | 0.34 | 0.36 | 1.40 | - |
Eicosaenoic acid (C20:1) | - | 0.23 | - | - |
Behenic acid (C22:0) | 0.92 | 0.11 | - | - |
Lignoceric acid (C24:0) | 0.25 | 0.05 | - | - |
Additionally, the byproduct obtained after C. oleifera oil treatment (seed cake) also has many valuable compounds. The total energy content is approximately 19,600 kJ/kg, and the main constituents include polysaccharides (26.09%), crude protein (15.94%), saponin (12.8%), crude fat (6.64%), crude fiber (6%), soluble sugar (4.3%), minerals (3.37%), tannins (2%), vitamins, and polyphenols (Li et al., 2020; Zhu et al., 2018). This is also a potential material for application in many agricultural fields.
3. Phytochemical composition of C. oleifera
Studies have shown that C. oleifera is a rich source of natural compounds with different types. The structures of about 249 compounds have been obtained and determined. After oil extraction, these compounds are found in different plant parts, such as flowers, leaves, roots, stems, seeds, and even seed baits (Luan et al., 2020).
They are classified into the five main groups, including triterpenoid, saponins, flavonoids, polyphenols, lignan, and other compounds (Luan et al., 2020). These main compounds are shown in Table 4.
Parts | Component name | References |
---|---|---|
Triterpenoid saponins | ||
Seed pulp | Oleiferasaponin C1 | Zong et al. (2015) |
Oleiferasaponin C2 | ||
Oleiferasaponin C3 | ||
Defatted seeds | Oleiferasaponin D1 | Fu et al. (2018) |
Oleiferasaponin D2 | ||
Oleiferasaponin D3 | ||
Roots | Oleiferoside N | Yang et al. (2015) |
Oleiferoside O | ||
Oleiferoside U | ||
Flower buds | Yuchasaponin A | Sugimoto et al. (2009) |
Yuchasaponin B | ||
Yuchasaponin C | ||
Trunk | Skullcapflavone II | Yan et al. (2015) |
Ursolic acid | ||
Betulinic acid | ||
Leaves | Lupeol | Chen et al. (2010) |
Kaempferol | ||
Quercetin-3-O-β-D-rhamnoside | ||
Flavonoids | ||
Seed pulp | Kaempferol-3-O-β-D-xylopyranosyl-(1 → 2)-β-D-glucopyranoside | Gao et al. (2011) |
Kaempferol-3-O-β-D-glucopyranosyl-(1 → 2)-β-D-glucopyranoside | ||
Kaempferol-3-O-α-L-rhamnopyranosyl-(1 → 6)-β-D-glucopyranoside | ||
Seed | Naringenin | Wang et al. (2017) |
Luteolin | ||
Myricetin | ||
Roots | Quercetin-3′-O-β-D-glucoside | Wang et al. (2019) |
Apigenin-7-O-β-D-glucoside | ||
3,4,5-Trimethoxyphenyl-6-O-syringoyl-β-D-glucopyranoside | ||
Flower buds | Quercetin-3-O-α-L-rhamnopyranoside | Sugimoto et al. (2009) |
Kaempferol-3-O-α-L-rhamnopyranoside | ||
Leaves | Quercetin-3-O-β-D-glucopyranoside | Chen et al. (2010) |
Quercetin-3-O-β-D-galactopyranoside | ||
Quercetin-3-O-β-D-rhamnoside | ||
Trunk | Skullcapflavone II | Yan et al. (2015) |
Polyphenols | ||
Seed pulp | p-Hydroxybenzoic acid | Jiao et al. (2019) |
Gallic acid | ||
Protocatechuic acid | ||
Seed | Epigallocatechin | Wang et al. (2017) |
p-Hydroxyphenylacetic acid | ||
Phthalic acid | ||
Lignan | ||
Dump | α-Conidendrin | Yan (2016) |
3’,4-O-Dimethylcedrusin | ||
(−)-Medioresinol | ||
Seed | (−)-Pinoresinol-4-O-β-D-glucopyranoside | Zhu et al. (2018) |
Methylpinoresinol | ||
(−)-Pinoresinol diglucoside | ||
Roots | (+)-Lyoniresinol-3α-O-β-D-glucopyranoside | Wang et al. (2019) |
Trunk | (−)-Pinoresinol | Yan et al. (2015) |
Other compounds | ||
Leaves | 1-(3’,5’-Dihydroxy)-phenyl-2-(4’’-O-β-D-glucopyranosyl)-phenylethane | Chen et al. (2011) |
1-(3’,5’-Dimethoxy)-phenyl-2-(4’’-O-β-D-glucopyranosyl)-phenylethan | ||
1-(3’,5’-Dimethoxy)-phenyl-2-[4’’-O-β-D-glucopyranosyl-(6 → 1)-O-α-L-rhamnopyranosyl]-phenylethane | ||
Roots | Rubusoside | Wang et al. (2019) |
Dulcoside B | ||
Seed | Carbamate 4,4′-diphenylmethane-bis (methyl) | Zhu et al. (2018) |
Dump | Acteoside | Yan et al. (2016) |
Seed coat | Ergosta-4,6,8(14),22-tetraen-3-one | Wang et al. (2017) |
(R)-de-O-Metillasiodiplodine | ||
6-Ethyl-5-hydroxy-2,7-dimethoxy-1,4-naphthoquinone |
Camellia oleifera is also a rich source of bioactive compounds. All compounds are extracted and purified from many parts of the plant. Saponin triterpenoids and their derivatives are mainly secondary metabolites found in plants and have been extensively utilized in medicine, agriculture, and cosmetics. Research on phytochemicals has revealed that triterpenoid saponins are the primary bioactive compounds in C. oleifera. These compounds have demonstrated significant anti-cancer properties by inhibiting tumor growth in different types of human cancer cells (Zong et al., 2015).
In addition, flavonoids and their derivatives are principal bioactive components obtained from C. oleifera. Some of these bioactive compounds have been shown to have pharmacological effects and are therefore receiving increasing attention. Fifty-three flavonoid compounds with antioxidant, antibacterial, cytotoxic, and anti-inflammatory capacities have been extracted and structurally determined from all plant parts, such as seeds, leaves, and roots (Luan et al., 2020). In addition, polyphenol groups are often said to exist abundantly in plants and have rich biological properties such as anti-cancer, anti-inflammatory, and antioxidant capacities. However, reports of phenolic compounds on the health-promoting properties of C. oleifera are still limited. Thus, more research on the properties of C. oleifera is needed to gain more information and develop products with high market value, such as health foods, cosmetics, and medicines (Wang et al., 2017). Polysaccharides also exist in C. oleifera, which are important biological macromolecules with positive biological activities. They are exerted on cell membrane structures and participate in various pharmacological activities, such as anticancer, antioxidant, immunomodulatory, and hypoglycemic activities (Zhang and Li, 2018).
Currently, more new compounds are being discovered in C. oleifera, Lee et al. (2006) isolated 12 lignans from the bark, seeds, and leaves. They may act as protective agents to prevent and treat free radical-related diseases. In addition to the groups mentioned above, other compounds have also been discovered. A total of 24 other components have been extracted from the roots, leaves, and fruit peels. Among these, three bibenzyl glycosides extracted from leaves showed strong cytotoxic activity against Hela and hep2 cells. Furthermore, rubusoside and dulcoside B extracted from roots exhibit antiangiogenic and antiallergic activities. In addition, dulcoside B is a natural sweetener that can be used for the controlled diet of people with diabetes (Wang et al., 2017).
4. Biological activity of C. oleifera
Both C. oleifera oil and seed cake have excellent antioxidant effects; seed cakes contain many biologically active compounds, such as sasanquasaponin, flavonoids, and tannins. According to Lee et al. (2007), lignan compounds in C. oleifera oil inhibited oxidative stress damage to red blood cells caused by 2,2’-azobis-(2-amidinopropane)-dihydropropane (AAPH). The induction and inhibition amounts were 49% and 48%, respectively. In addition, when using different types of solvents, they produce different antioxidant effects. Typically, the glycerol-ethanol mixture provides extracts with better antioxidant effects than glycerol or ethanol solvents. The extract from seed cake also has excellent antioxidant properties and significant antibacterial effects (Zhang et al., 2020).
According to Cheng et al. (2014), intestinal epithelial cells treated with C. oleifera oil (50-75 μg/mL) acted as a barrier against oxidative damage to the gastrointestinal mucosa. Furthermore, administering C. oleifera oil at 2 mL/kg/day significantly inhibited ketoprofen-induced gastrointestinal mucosal damage in mice (Table 5). These findings showed an anti-ulcer effect against oxidative damage in the stomach and intestines caused by ketoprofen. Lee et al. (2018) fed mice 2 mL/kg body weight/day of the following oils: C. oleifera, olive, and soybean oil. After 20 days, the absorption of C. oleifera oil increased the ratio of Firmicutes/Bacteroidetes increased Bifidobacteria density and reduced intestinal Prevotella. These results show that C. oleifera oil can improve the decline of the anti-inflammatory system caused by acetic acid compared to olive and soybean oil, possibly because C. oleifera oil changes intestinal microflora or is a rich source of phytochemicals. In addition to oil, asanquasaponin hydrolyzed from defatted seeds helps reduce edema caused by carrageenan and the writhing reaction caused by acetic acid in mice (Ye et al., 2013). In addition, the anti-inflammatory effect of biflavonoids obtained from shells of C. oleifera was studied in mice with otitis caused by croton oil, showing a reduction in the inflamed ear at a dose of 200 mg/kg, biflavonoids inhibited inflammation by up to nearly 72% (Ye et al., 2012).
Materials | Dosage and effects | References |
---|---|---|
Oil | With a dosage of 2 mL/kg/day, it can inhibit the gastrointestinal mucosal damage caused by ketoprofen in mice. | Cheng et al. (2014) |
With a dose of 2 mL/kg body weight/day, it significantly reduces damage caused by inflammation and lipid peroxidation, improving colitis caused by acetic acid. | Lee et al. (2018) | |
Extract | Decreased paw edema in rats; the maximal inhibition of inflammation was up to 60.3% at 200 mg/kg. | Ye et al. (2012) |
Decreased ear inflammation caused by croton-oil in mice; the maximal inhibition of inflammation was up to 71.9% at 200 mg/kg. |
Overall, the above studies show that using C. oleifera oil and some compounds from their extracts has anti-inflammatory properties in mice. This has also been proven since ancient times when people applied C. oleifera seed oil to burns and used it to treat stomach aches, and relieve pain (Ye et al., 2013).
Until now, there have been many studies on the anticancer ability of compounds in C. oleifera, such as research by Di et al. (2017) on two compounds Camelliasaponin B1 and Camelliasaponin B2, at high concentrations. A concentration of 10 μM significantly inhibited human lung cancer cell proliferation with 94.44% and 79.12% inhibition rates, respectively. Similarly, the biologically active compounds isolated from C. oleifera stems demonstrated significant cytotoxic activity against five tumor cell lines (BGC-823, HCT-8, A5049, Bel-7402, and A2780), with IC50 values ranging from 3.15 to 7.32 μM (Yan et al., 2016). In addition, according to a study by Fu et al. (2018), two compounds oleiferasaponin D1 and oleiferasaponin D2, purified from defatted seeds of this material, also showed strong cytotoxic capacity. The IC50 values range from 3.31 to 10.23 μM. For breast cancer, a common disease-causing high mortality in women around the world, Chen et al. (2013) showed that the compound sasanquasaponin inhibited 80% of breast cancer cells at a concentration of 30 μM (Table 6).
Materials | Dosage and effects | Author |
---|---|---|
Extract | A concentration of 10 μM significantly inhibited human lung cancer cell proliferation. | Di et al. (2017) |
At a concentration of 30 μM, it inhibits 80% of breast cancer cells. | Chen et al. (2013) | |
The compound exhibited cytotoxic activity against five human cancer cell lines (BGC-823, HCT-8, A5049, Bel-7402, and A2780), with IC50 values ranging from 3.15 to 7.32 μM. | Yan et al. (2016) | |
With IC50 values ranging from 3.31 to 10.23 μM, cytotoxicity against 5 human cancer cell lines (HCT-116, HepG2, BGC-823, NCI-H1650, and A2780). | Fu et al. (2018) |
Generally, the bioactive components of C. oleifera possess outstanding anticancer potential based on in vitro experiments. However, further studies in clinical settings on the anticancer properties of C. oleifera are essential for studying its anticancer effects in vivo. This will help clarify the anticancer properties of the compounds contained in C. oleifera.
Currently, there is a growing focus on addressing food hygiene and safety issues using antibacterial compounds. Previous observations have proved that extracts from C. oleifera exhibit robust resistance against bacteria, such as Escherichia coli, Salmonella, and Bacillus subtilis, as well as the fungus Mucor racemosus. Oil from C. oleifera seeds has been shown to possess quite strong antibacterial activity when inhibiting E. coli with a minimum inhibitory concentration (MIC) of 3.9 mg/mL compared with standard doses of gentamicin; the MIC was 4.2 mg/mL (Feás et al., 2013). Besides, saponins extracted from defatted C. oleifera seeds have shown strong antibacterial activity against E. coli and Staphylococcus aureus with MICs of 71.4 and 94.5 μg/mL, respectively (Ye et al., 2015).
In addition to saponins, flavonoids (at concentrations ranging from 0 to 200 mg/mL) extracted from seeds can drastically reduce inflammatory responses (Liu et al., 2014). In addition to inhibiting bacteria, it can also inactivate yeast and mold. Research on the total amount of triterpenoid saponins isolated from C. oleifera shows high inhibitory activities against B. maydis; the MIC was 250 μg/mL for 24 hours, and the fungal hyphae were shrunken, collapsed, and hollow. The hyphae, plasmalemma, and cell walls are broken, coagulate, or leak cytoplasm, hindering the growth of the mycelium (Zhang et al., 2014). For these reasons, C. oleifera has the potential to be used as a natural antibacterial compound in food.
Lee et al. (2007) demonstrated that saponin compounds from C. oleifera seed oil can reduce the cholesterol, triglyceride, and low-density lipoprotein (LDL) content in mouse blood. C. oleifera seed oil is rich in oleic acid, similar to olive oil, and is considered the primary source of fat in the Mediterranean diet.
According to research by Lee et al. (2007), the methanol extract obtained from C. oleifera seed oil can reduce the formation of intracellular reactive oxygen species (ROS), inhibit the oxidation of LDL, and protect lymphocytes against genetic damage caused by H2O2. The findings showed that C. oleifera seed oil diet significantly reduced serum levels of liver enzyme markers. Treating pets with C. oleifera seed oil (150 g/kg diet) may enhance the activity of hepatic glutathione peroxidase, glutathione reductase, and glutathione S transferase compared with the CCl4-treated group. Thus, according to the findings of this study, when using C. oleifera seed oil in the diet, the liver will be protected against oxidative damage caused by CCL4 in mice, and the hepatoprotective effect may be correlated with its antioxidant and free radical scavenging effects.
The above studies show that C. oleifera seed oil is a potential raw material with excellent health protection effects and can be used to replace soybean oil and olive oil. Although it has been used in some localities, many places still do not know about this type of oil, and it still needs to be widely available. Therefore, it is necessary to scale up production, introduce it to everyone, and reduce the load of other oil seeds, ensuring food security.
Hypertension is one of the most common diseases worldwide today. Being able to control blood pressure accurately can help reduce the incidence of complications. Data measuring tissue activity have shown that the kidneys, lungs, and heart are the main sites of action for lowering blood pressure by inhibiting their angiotensin-converting enzyme (ACE) activity (Chiang et al., 2021). Research results have shown that C. oleifera can inhibit ACE activity and lower blood pressure similarly to olive oil in both acute and long-term use experiments. Furthermore, determining biochemical factors in serum has also demonstrated that C. oleifera can regulate the balance between its concentrations. Based on this, it can be seen that C. oleifera has a positive antihypertensive effect (Chiang et al., 2021).
Some scientists recently assessed the antihypertensive capacity of C. oleifera mixed with Eucommia extract complex administered to rats for 120 days. These mixtures reduced body weight, systolic blood pressure, diastolic blood pressure, mean blood pressure, and heart rate (Du et al., 2019). This shows that the mixture has a strong antihypertensive effect on the cardiovascular system of rats.
Until now, C. oleifera has been used as a food and medicine on a small scale in households and locally and is still not widely available. Studies on the toxicity of any source of raw materials are important in determining food safety. However, C. oleifera has been used for a long time, but until now, there have been no clear, in-depth studies on its toxicity. Currently, clinical toxicity research on humans has not been carried out; dosage research has only been tested on mice, and no toxicity or mutagenicity has been observed. Therefore, it can be partly confirmed that C. oleifera is safe and a potential food that can be used daily (Zhang et al., 2011). To use C. oleifera oil safely, we must conduct comprehensive studies on its toxicity to ensure consumer safety. This is also a new research direction that scientists need to consider.
Although many pharmacological effects have been proposed for C. oleifera, reports on this plant’s safety and side effects and its bioactive components still need to be made available. Information should be provided regarding its reliability for safe future use.
5. Other applications
In addition to applications in food and medicine, C. oleifera seed oil is also used as a raw material in industries, such as soap, margarine, paint, and anti-rust oil. At the same time, it is also used to synthesize highly biologically active compounds (Chen et al., 2010). According to research by Chen et al. (2010), saponin compounds extracted from C. oleifera seed oil can be used as foam stabilizers and emulsifiers and can be used to produce detergents. The crude saponin extract obtained from defatted C. oleifera seed powder has a moderate foaming ability.
According to research by Quan et al. (2022), the seed coat of C. oleifera is an excellent raw material consisting of cellulose, hemicellulose, and lignin. It has been used to produce activated carbon, and this product possesses a high absorption capacity, good yield, low cost, and guaranteed raw material supply. It is widely used in water decolorization and filtration. This product has many outstanding aspects compared to conventional activated carbon.
These studies show that C. oleifera is an excellent source of raw materials with high applicability. Parts from the shell to the seeds and seed residue are used to produce many products for the medical, food, and industrial sectors. This potential raw material source can replace other oil-producing materials such as peanuts, soybeans, olives, canola seeds, etc.
6. Conclusions
In general, this article gives a comprehensive overview of the nutritional and chemical composition, biological properties, and industrial applications of C. oleifera based on recent findings. Research on the chemical composition of C. oleifera has revealed the presence of fatty acids, triterpenoids, saponins, flavonoids, and polyphenols, which exhibit a wide range of beneficial properties. These include anti-cancer, anti-inflammatory, antioxidant, anti-hypertensive, antibacterial, liver, and stomach protective effects and the ability to reduce LDL cholesterol. However, a few points also need to be noted and further researched. For example, using C. oleifera by-products can be a new approach to waste management from an economic and environmental perspective. Recovering and using valuable compounds from C. oleifera is also a significant challenge for scientists.