1. Introduction
Ogi, a fermented product from cereal grain with traditional recognition as a weaning formula, is used as breakfast cereal when boiled into a thick paste called akamu, agidi. It is a popular staple product in Nigeria. The preparation of cereal such as maize into ogi involves united processes. During these processes, macro and microelements and proteins are lost, which affects the robust nutritional profile of the products (Adeyemi and Beckley, 1986; Akingbola et al., 1981). Due to the loss of essential nutrients, fortification of ogi has been extensively researched with okra, cowpea, soybean, egg, and crayfish (Aminigo and Akingbola, 2004; Oyarekua, 2010). The use of Aduwa protein or Balanites aegyptiaca proteins and hydrolysate as fortificants is limited, but Balanites aegyptiaca is a browse plant that bears seeds with a myriad of bioactive properties such as anti-hypertensive, anti-diabetic, and antioxidant properties (Datti et al., 2020; Ogori et al., 2022). The leaves, seeds, and fruit of Balanites aegyptiaca have been reported as edible (Datti et al., 2020; Ogori et al., 2018; Okia et al., 2016). Balanites aegyptiaca seed products may be an alternative to soybeans, moringa leaves, and their seed supplementation in managing malnutrition. The menu of many rural and urban dwellers is lacking in functional nutrients nowadays with increasing economic reality in developing countries. Balanites aegyptiaca plant seeds have been reported to have hypotensive activity and antioxidant properties (Datti et al., 2020; Ogori et al., 2022; Ogori et al., 2023). Seeds and leaves from Balanites aegyptiaca are widely used as oral antidiabetic and anticancer remedies in traditional medicine. Generally, seed nut protein has been documented to alleviate COVID-19 infection and diabetes and act as an antioxidant agent (Asen et al., 2021; De-cargo et al., 2017; Ogori et al., 2022; Rusu et al., 2019; Sathe, 1994). The biologically active saponin and flavonoid in Balanites aegyptiaca contribute significantly to nutraceutical applications, thus the wide consumption of meal and seed flour by humans as food. Aduwa or Balanites aegyptiaca seeds have been reported as anti-helminthic, purgative, and of high food value (Chapagain et al., 2009; Dwivide et al., 2009). The seed has a wide range of ethnomedicinal value and excellent nutritional qualities. Some mineral elements have been attributed to modulate diabetes. Macro and microelements have been recognized in diabetes progression (Khalid et al., 2014). These elements could be furnished by Balanites aegyptiaca seed proteins. Macro elements, when combined with vitamins, can ignite hormone production as well as awaken metabolic processes. They have been attributed to cellular and subcellular functions (Khalid et al., 2014). The use of Mg, Ca, K, Na, Zn, Mn, and Cu in diabetes treatment and management has been published (Candilish, 2000; Nourmahammadi et al., 2000), and this was done by the activation of insulin receptor sites (cofactor components of enzymes) and through insulin sensitivity (Khalid et al., 2014). The pasting dynamics of starch food contribute to the food aesthetic in vivo biomaterial leaching and quality and aid in industrial settings or product processing and application. These attributes of starch are also extended to texture, digestibility, and final use of starch. The result of this study will provide a choice menu that will increase the utilization, selectivity, and rheological behavior of the ogi functional mix for both adults and growing children. Therefore, the aim of this study was to assess the mineral profile and pasting behavior of ogi mix with Balanites aegyptiaca protein meal, concentrate, and hydrolysate with the view of improving the utilization of this traditional breakfast and weaning meal food.
2. Materials and methods
All the reagents used were provided by the Food Science Department Obafemi Awolowo University, Ile Ife. One kilogram (1 kg) of mature B. aegyptiaca fruits were bought from the local Gashua market in Yobe State of Nigeria. They were conveyed to the biochemistry laboratory of the Federal University Gashua. One kilogram (1 kg) of cracked seed kernels of Balanites aegyptiaca was weighted using a weight balance, and moisture value was ascertained at 10% using prob. The weighted samples were given toasting treatments under dry heat at 70°C for 30 minutes to enable oil expulsion by mechanical way and to reduce anti-nutrient contents.
Fortification of Maize ogi with B. aeqyptiaca seed concentrate and hydrolysate by Material complexation method was adopted Echezarreta et al. (2000) (Balanites aegptiaaca) Aduwa samples and ogi samples at (16%) protein target inclusion was weighed and transferred to a crucible. The mixture was mixed with a small volume of water to form a homogenous flow meal. The meals were mixed thoroughly and mildly dried. The final product was packaged and kept for analysis.
Preparation of defatted B. aeqyptiaca seed protein meal was prepared from toasted seed. The meal sample was defatted with cold (−4°C) acetone using a flour-meal-to-solvent ratio of 1:5 w/v. The mixture was stirred over a magnetizing stirrer for 4 hrs and filtered through a Whatman No. 1 filter paper. The residue was re-extracted twice for proper fat extraction from the meal sample. The defatted flour meal was put to drying in a fume hood at room temperature, and the dried flour meal was finely ground in a blender to obtain homogeneous defatted Aduwa meal -flour and stored in an air-tight plastic bottle for use.
Protein concentrate (APC) from Aduwa was prepared by a modified method (Gbadamosi et al., 2012). A known weight (100 g) of the defatted meal flour was dispersed in distilled water (1,000) mL to give a final meal-flour-to-water ratio of 1:10. The dispersion was gently stirred on a magnetic stirrer for 10 min to form a suspension, after which the pH of the resultant slurry was adjusted with 0.1 M HCl to pH 4. The precipitation process was allowed to proceed with gentle stirring for 2 h keeping the pH constant. Soluble material was removed by centrifugation for 30 min using a centrifuge. The precipitate or concentrate was washed with distilled water to remove the residual minerals and soluble carbohydrates, and the pH was later adjusted with 0.1 M NaOH to 7.0 for neutralization and then centrifuged for 10 min. The resultant precipitate or concentrate was freeze-dried and kept in an air-tight container for further analysis.
Combine enzyme hydrolysate PP was carried out using the method of Aluko and McIntosh (2006) with slight modification by Girgih et al. (2015). The APC was dispersed in water (2%, w/v) and was adjusted to pH 9.0 using 1 M NaOH solution for pancreatin, while pH 2.0 was used for pepsin digestion. The dispersion was heated to 60°C under a continuous stirrer on a hotplate with an electronic thermometer. The pancreatin and pepsin enzymes (4%, w/w), respectively, were added based on the protein content of the APC and incubated at a constant temperature of 60°C for 10 min. The reaction mixture was maintained at pH 9.0 using 1 M NaOH solution or pH 2.0 with 1 M HCl after adding pepsin enzyme. At the end of the incubation period, the hydrolysates were transferred into a boiling water bath for 5 min to inactivate the enzymes. The hydrolysate was cooled to room temperature (22±1°C) and adjusted to pH 7.0 with 1 M HCl solution (for pancreatin) or 1 M NaOH (for pepsin digest), and finally freeze-dried.
Five grams (5g) of (B. aeqyptiaca) derived sample was heated gently over a Bunsen burner flame until most of the organic matter was destroyed. This was further heated strongly in a muffle furnace for several hours until white-grey ash was obtained. The ash material was cooled. Twenty milliliters of distilled water and 10 mL of dilute hydrochloric acid were added to the ached material. The mixture was boiled, filtered into a 250 mL volumetric flask, washed thoroughly with hot water, cooled, and made up to volume. The mineral content of each sample was analyzed using the spectrophotometric method (AOAC, 2012). Samples were analyzed for sodium (Na), potassium (K), calcium (Ca), iron (Fe), magnesium (Mg), zinc (Zn), copper (Cu) and phosphorus (P).
The pasting properties were measured using a Rapid Visco Analyser (Newport Scientific Australia) as described by (Oladiji et al., 2018). Derived samples (2.5 g) each were weighed into a dried empty canister; 25 mL of distilled water was dispensed into the sample. The solution was thoroughly mixed, and the canister fitted well into the RVA, as recommended. The slurry was heated from 50-95°C with a holding time of 2 mins followed by cooling to 50°C with 2 mins holding time. The rate of heating and cooling was at a constant rate of 11.25°C/min. Peak viscosity, trough, breakdown, final viscosity, set back, peak time and pasting temperature were read from the pasting profile with the aid of thermocline for Windows Software connected to a computer.
3. Results and discussion
The mineral profile of fortified ogi from (B. aegyptiaca Del) proteins and hydrolysate is presented in Table 1. There was a significant difference in the calcium content of the samples. DAM-ogi and APC-ogi had the highest calcium content, while PP-ogi had the lowest. The magnesium content in the samples revealed that DAM-ogi was significantly higher. However, there was a significant (p<0.05) difference between the control-ogi and PP-ogi samples. The least magnesium content was found in the control-ogi (5.70g/L), but it was above the recommended values by RDA and within the normal serum range of 0.8-1.2 mmol/L. The APC-ogi sample revealed the highest potassium content, significantly (p<0.05) different among the samples. However, the control-ogi had the lowest value of potassium (5.46g/L, which was above the serum range of 136-145 mmol/L (Khalid et al., 2014). The sodium content of PP-ogi [185 g/L was significantly (p<0.05)] higher, but the control-ogi, DAM-ogi, and APC-ogi samples were not significantly different (p<0.05) but were above 3.4-5 mmol/L. The manganese content in DAM-ogi and APC-ogi was significantly higher compared to control-ogi, PP-ogi, and the RDA values. The low value of manganese in PP-ogi may be due to the hydrolysis of the sample. There was no significant (p<0.05) difference in iron mineral contents between DAM-ogi and APC-ogi, but there was a low value in the control-ogi sample (1.30g/L). The serum range was put at 60-70 μg/dL, which was below the value from this study. Copper was higher in APC-ogi compared to DAM-ogi, PP-ogi sample, and the referral mineral copper RDA values, however, within the serum range of 70-140 μg/dL. The zinc content of the sample differs with fortification as APC-ogi was significantly (p<0.05) higher compared to the control-ogi, DAM-ogi, PP-ogi samples, and RDA values, respectively. The result reflected that APC and DAM samples, when used as fortificants, could improve the macro and micro elements of calcium, potassium magnesium, iron copper, and zinc compared to the hydrolysate samples with the incremental impact of sodium on the ogi sample.
All values are mean±SD (n=2). Different superscript letter (a-d) in each column indicate significant differences (p<0.05).
Keys: control, DAM-ogi = defatted Aduwa protein meal and ogi, APC-ogi = Aduwa protein concentrate and ogi. PP-ogi = Aduwa protein hydrolysate by pancreatin+pepsin and ogi.
The changes in the mineral profile may be due to the extraction protocols of (B. aegyptiaca Del) proteins. Calcium is essential for maintaining a healthy body and could help as an indicator in insulin resistance in humans, with a recommended value between 0.34-0.45 mg/dL and a serum range of 2.2-2.6 mmol/L. Our body needs calcium every day not just to keep our bones and teeth strong but also to ensure the proper functioning of muscles and nerves and to help our blood clot properly. Calcium deficiency in adults and children can be handled with functional ogi mix from (B. aegyptiaca Del) protein meal concentrate and hydrolysate. Sodium elements in PP-ogi, compared to other samples, revealed good biomaterial for electrolytic and ionic balance. Magnesium is the fourth most common mineral in the human body after calcium, sodium, and potassium, as well as the second most common intracellular cation after potassium. Magnesium is a cofactor in many enzyme systems and is also required for such fundamental processes as energy production and nucleic acid synthesis and plays an important role in biomaterial synthesis such as the influence on insulin secretion and its binding activities (Gerry and Stephen 2017; Khalid et al., 2014). Magnesium content was high in DAM-ogi compared to the other samples under study and the 0.142/100 g/g value reported by Detti et al. (2020). The normal serum magnesium levels of 1.8 and 3.0 mg/dL have been reported by Khalid et al. (2014), and these recommended values were below DAM-ogi and hydrolysate-ogi, indicating a potential vasodilator and neurotransmitter bioactive material. Magnesium is important in improving insulin resistance (Volp, 2008). APC-ogi (0.18/100 g/g) was significantly (p<0.05) rich in copper compared to DAM-ogi (0.15/100 g/g) and hydrolysate-ogi (0.14/100 g/g) respectively. Copper plays a significant role in enzyme catalysis and could be a dangerous reactant in producing a hydroxyl radical when in excess. The standard serum range in the body was between (70-140) μg/dL below the functional-ogi range samples. The copper mineral has been linked to lipogenesis, hypercholesterolemia, and arteriosclerosis, associated with diabetes Khalid et al. (2014). The iron value in DAM-ogi and APC-ogi was higher than in the other samples. Iron is a mineral that serves several important functions; its main function is to carry oxygen throughout the body and to make red blood cells (Beard and Dawson 1997). The normal iron range in human serum is 70-140 μg/dL. This value was below the recorded value from this study and the reference RDA value recommended. Impaired glucose metabolism and diabetes mellitus are common clinical iron overload in patients with hemochromatosis. Ferritins are protein iron-like structures that have been implicated in tissue iron storage level, which is like, as well as diabetes prevalence Eshed et al. (2011). Zinc plays a role in enzyme activities, in wound healing, and in the treatment of diarrhea. It has been implicated as a cofactor for intracellular enzyme activities, regulating insulin receptor signal-modulating mechanisms Khalid et al. (2014). The zinc and copper values from APC-ogi samples significantly revealed that these samples could be better preferred for child food fortification to aid in growth and sexual maturity in growing children and in controlling diabetes in adults. The results show that functional ogi samples are rich in macro and microelements, and these values agree with the values reported by RDA (USFDA, 2022). Fortification with these protein materials could curb child and adult tetany, osteomalacia, and related diseases due to the lack of calcium, potassium, calcium, and sodium which are electrolytes needed for the nerves, myocardial regions, and neurons.
The pasting properties of fortified ogi from B. aegyptiaca proteins and hydrolysates presented in Table 2 showed that the Control-ogi showed significantly higher peak viscosity than APC-ogi and PP-ogi. Similar observations were seen in breakdown viscosity, but the trough viscosity was significantly high in control-ogi and PP-ogi samples compared to APC-ogi under this study (p<0.05). The final viscosity, setback viscosity, and pasting temperature of PP-ogi functional ogi were significantly higher than DAM-ogi, Control-ogi, and APC-ogi samples (p<0.05). The peak time of DAM-ogi and APC-ogi was higher than that of Control-ogi and PP-ogi samples. The result revealed that the rheological behavior of the ogi was improved when PP hydrolysate was used compared to DAM and APC samples. However, the peak time of the sample was significantly improved when DAM was used, and peak temperature was favored when the APC sample was incorporated into the ogi mix products (Fig. 1C).
All values are mean±SD (n=2). Different superscript letter (a-d) in each column indicate significant differences (p<0.05).
Keys: control, DAPM-ogi = defatted Aduwa protein meal and ogi, APC-ogi = Aduwa protein concentrate and ogi. PP-ogi = Aduwa protein hydrolysate by pancreatin + pepsin and ogi.
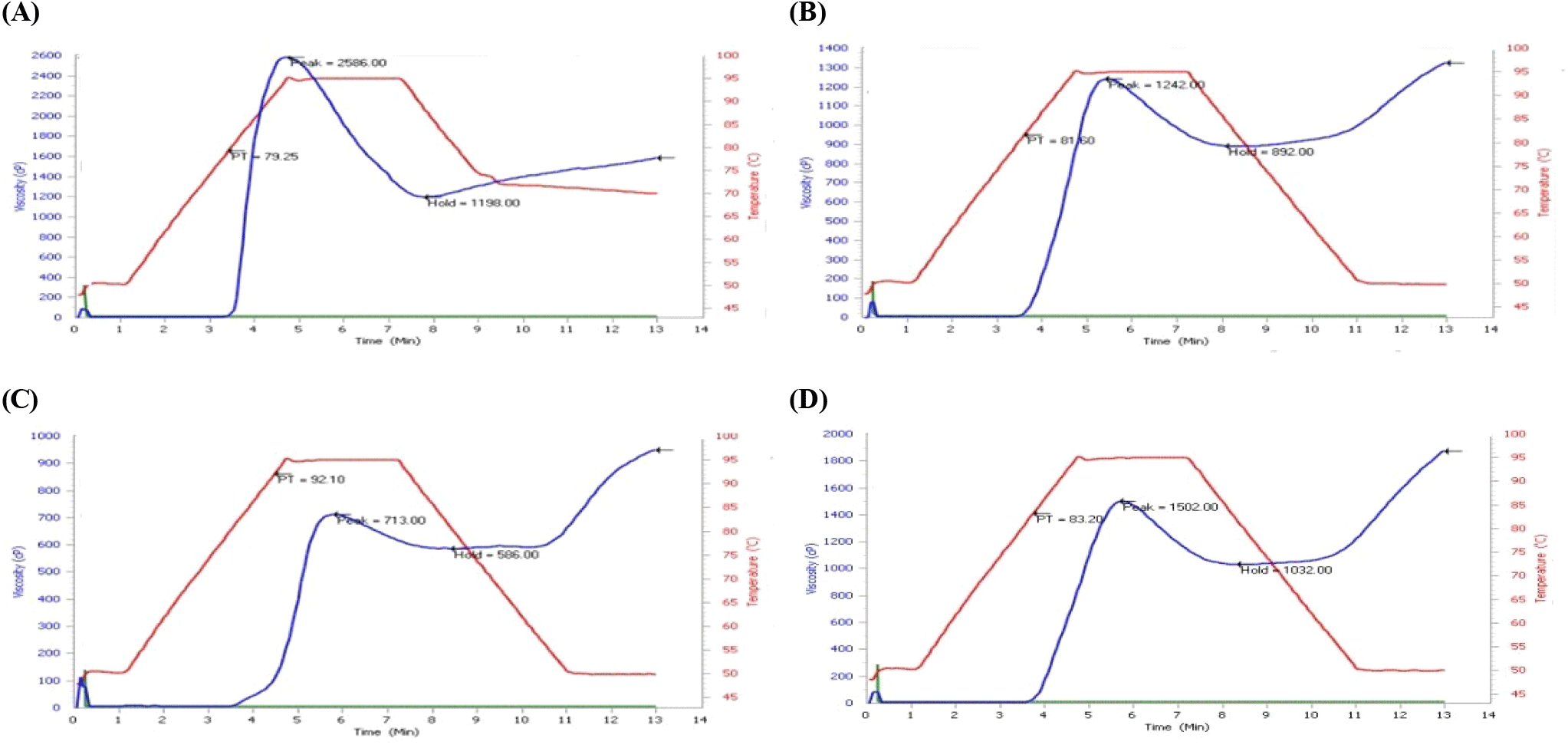
The pasting properties of the fortified ogi from B. aegyptiaca protein and hydrolysate samples, such as peak viscosity, trough, breakdown, final viscosity, setback viscosity, pasting time, and pasting temperature were generally dependent on the protein/peptide and starch granules interactions. But in this study, it was about the hydrophobic amino acid residues versus amylose molecular integrity. These, in turn, influenced the granule swelling potentials and the extent of amylose or protein leaching. The pasting profile decreased with protein and peptide enrichment, and a low value was observed in DAM-ogi and APC-ogi compared to hydrolysate-ogi samples (Fig. 1B-1D). This indicates that proteins/peptides reduce gelling ability. A similar observation was noticed when okra seed was substituted with ogi and bambara nut with ogi (Amingo and Akingbola, 2004; Theodore et al., 2009). Peak viscosity had a good range in sample PP-ogi but was low compared to the control-ogi sample. The trough and breakdown viscosities had similar trends as the peak viscosity. Both final and setback viscosities increased in the PP-ogi sample but decreased in APC-ogi, DAM-ogi, and control-ogi samples. The pasting time and temperature increased in the APC-ogi sample, and the least was in the control-ogi sample. Peak viscosity usually reveals the strength of the paste in relation to the thickening effect. This could be generated from gelatinization steps during the process of food application. High peak viscosity reflects the thickening or swelling power of the mix. Hence, peak viscosity is the maximum viscosity developed during or soon after heating. Peak viscosity generally predicts the biomaterial load of the sample. The hydrolysate-ogi has the highest peak viscosity compared to APC-ogi, however, lower than DAM-ogi and control-ogi. This was not reflected due to the low swelling properties of PP-ogi, which may be due to the peptide-starch binding nature of the sample. The trough viscosity came from the differences between the peak viscosity and the breakdown viscosity; this defined the past capacity or the ability of the starch to break down slowly, revealing the conformational strength of the sample projected on the peak viscosity. The hydrolysate-ogi had a better trough viscosity value compared to the APC-ogi sample, however, lower than the control-ogi. This implies that hydrolysate-ogi has a higher material holding capacity due to the peptide/starch matrices giving the ability to withstand breakdown during cooling. The trough viscosity is important to check critical processing stages during paste-making in food-related processes in the industry. Breakdown viscosity (BV) measures the extent the swollen starch disintegrates into smaller fragments. It is the resistance of paste to shearing effects. Breakdown (BV) was very low in APC-ogi compared to hydrolysate-ogi. This revealed that biomaterials from control ogi, DAM-ogi, and PP-ogi samples disintegrate slowly. The breakdown viscosity in the control-ogi was, however, high. Therefore, control-ogi and hydrolysate-ogi samples revealed peak stability during past-related food processing. From this study, the control-ogi had better breakdown viscosity, followed by the hydrolysate-ogi sample revealing ogi starch and peptide-starch molecular integrity. The hydrolysate-enriched ogi sample could hold swollen starch amylose from easy disintegration or sip out and may require lower shear mechanical stress. The final viscosity ranged high in the samples, but the APC-ogi sample had the lowest and the hydrolysate-ogi sample with the highest value of the final viscosity readings. The final viscosity (FV) reflects the starch's ability to form a highly viscous mix; this process defines processing potentials and is an advantage in infant feeding because solid or liquid adjuncts may not be required. The hydrolysate-ogi hydrolysate sample of the enriched-ogi mix increased in final viscosity compared to the control ogi. This may be due to the leaching of biopeptide molecules due to dissociation. According to Miles et al. (1985) increase in final viscosity may be due to the aggregation of the amylose molecules. The Setback viscosity measures synaeresis or liquid sipping, reassociation, or stalling process. The process in starch is observed when the starch paste is cooled. The present study showed that setback viscosity in the APC-ogi sample had the lowest and hydrolysate-ogi had the highest setback viscosity values respectively. The reordering ability of the molecules or gelling in the hydrolysate samples was significantly higher than the control-ogi and APC-ogi as well as the control-ogi sample. In support of the above observation, Niba et al. (2001) defined setback viscosity as the gel stability and potential for starch to retrograde. Hence the gel-forming ability of hydrolysate was high compared to the other samples (Fig. 1D). Pasting time and pasting temperature were low in the control-ogi and PP-ogi samples, but the APC-ogi sample had the highest pasting time and temperature values; this reflects the swelling capacity of the APC-ogi sample. The pasting temperature (PT) is the initial rising temperature of the starch viscosity. The higher pasting temperature of the APC-ogi indicated high biomaterial resistance towards swelling, which in turn affected the pasting time to 5.80 minutes higher (Fig. 1C). This observation could be due to high-fat content, smaller granular size of the sample, or material denaturation of the APC protein molecules. The attainment of the pasting temperature is essential in the swelling, gelatinization, and gel formation of the enriched mix during the past-time-making process and energy requirement. The results of pasting properties showed that the peak, trough, breakdown, final viscosity, and setback viscosity of the APC-ogi sample were low compared to the hydrolysate-ogi sample, meaning that the addition of B.aegyptiaca protein concentrate reduced the sample’s pasting properties. This observation was similar to the report of soy isolate and cassava starch mix (Chiemela et al., 2011), when groundnut meal was added to wheat flour (Ocheme et al., 2018). These observations had a contrary view when the B. aegyptiaca hydrolysates sample was used to fortify ogi. The peak, breakdown, trough, and final viscosities could not translate to high water absorption quality because hydrolysate-ogi is a peptide sample and its molecule would have bound to more amylose molecules by occlusion. A similar observation was reported by Xiaoxue et al. (2022) when the peptide was added to rice starch and there was reduced leaching of amylose resulting from the prevention of water migration into the starch molecules. Peptides prevent the diffusion of amylose during gelatinization which eventually reduces the leachable amylose due to complexes between peptides and ogi-amylose molecules Chi et al. (2018). Chen et al. (2019), attributed this formation of complexes between peptides and amylose to hydrogen bonding and hydrophobic interactions that would have increased the pasting stability of hydrolysate-ogi mix product.
4. Conclusions
The fortification by the complexation approach of Balanites aeqyptiaca Del protein concentrates and hydrolysate from pancreatin and pepsin enzymes to ‘ogi’ increased the mineral profile and pasting properties. The pasting property of B. aeqyptiaca Del hydrolysate improved the rheology of starchy swollen granules in the ogi food sample and allowed possibilities of greater surface area for mineral sipping when ingested. Therefore, it is important to encourage the use of Balanites aeqyptiaca Del protein and peptides to fortify traditional cereals such as maize, millet, or sorghum; by this, the increasing incidences of malnutrition, diabetes, and hypertension in homes would be drastically reduced. There is a need for future research in the use of these samples as bio-preservatives in commercial flour production for confectionaries.