1. Introduction
Patulin, 4-hydroxy-4H-furan (3,2C)-pyran-2 (6H)-1, is an organic compound classified as a polyketide. It is a mycotoxin produced by approximately 30 fungal genera, including Aspergillus, Penicillium, and Byssochlamys (Boonzaaijer et al., 2005; Shephard and Leggott, 2000; Varga et al., 2003).
Patulin is found extensively in foods, such as fruits, apples, and grains. A typical symptom of patulin poisoning is acute digestive disease. It occurs mainly in infants (Drusch et al., 2007; Lai et al., 2000). Patulin has been known as a mutagen affecting the nervous and immune systems (Llewelly et al., 1998).
Especially it is reported that patulin contamination occurs most often in apples and apple juice. Studies have reported that patulin contamination in commercial apple juice was 10-350 μg/L (Ritieni, 2003; Sewram et al., 2000). Hence, patulin is an important safety factor in apples and apple juice (Mahfoud et al., 2002; Rychlik et al., 2004). The quarantine standard of patulin in apple juice is regulated at <50 μg/L (Bullerman, 1979; WHO, 1995).
Therefore, lowering patulin levels is necessary as a major risk factor for apple juice. WHO (1995) proposes that the standard for patulin content in apple juice be 50 ppb or less (Bullerman, 1979). Patulin contamination can be reduced through fermentation, leading to a negligible level in both vinegar and alcoholic fruit beverages from fruit juices. However, heat treatment rarely reduces patulin contamination, so the patulin present in apple juice survives pasteurization (WHO, 1990).
Aspergillus spp. on feeds could be controlled by 5 kGy gamma irradiation but detoxification of AFB1 demands a higher dose of gamma irradiation (≥10 kGy) (Nam et al., 2010). As gamma irradiation dose increases, the concentration of aflatoxin B1 decreases, especially at low concentrations (1 ng/ mL), aflatoxin B1 decreased by 50% to 0.5 ng/mL with 3 kGy irradiation. It was completely removed by irradiation of more than 5 kGy. Yun et al. (2008) reported the effect of gamma irradiation on the growth and patulin production of Penicillium griseofulvum in an apple model system. Zegota et al. (1988) also reported radiation-induced disappearance of the mycotoxin in relation to the absorbed dose. Ahn et al. (2022) reported that N-nitrosodimethylamine of the sausage irradiated at 10 kGy or above reduced in aerobic packaging, while a dose of 20 kGy was needed in vacuum packaging.
It is possible to control the patulin content to some extent in apple juice by different treatments, but the most practicable method is to remove rotted and damaged fruit by hand before producing the juice (Sydenham et al., 1995). According to the FDA, controlling patulin levels to ≤50 μg/kg using processors can be mainly achieved by removing damaged and/or spoiled raw apples from the product stream to produce apple juice. Other methods like water treatment can be useful for reducing patulin levels. Also, Sydenham et al. (1995) confirmed an apparent reduction in patulin levels in juice production by both initial water treatment and removal of rotted and damaged fruit by hand.
Response surface methodology (RSM) is a useful tool for optimizing chemical reaction conditions and industrial processes (Barros Neto et al., 2003; Gontard, 1992; Mayers and Montagomery, 1995). The main advantage of this RSM is to understand how the independent variables (process variables) affect the selected response variables and further to statistically determine the possible correlations between the independent variables in the responses of the process variables.
This study was conducted to monitor the changes in polyphenolic content and residual patulin content affected by gamma irradiation-combined subsequent storage by applying response surface methodology to evaluate the most influential parameters for reducing patulin content in apple juice.
2. Materials and methods
Patulin standard and chemical reagents, such as acetonitrile, ethyl acetate, formic acid, toluene, and Folin-Ciocalteau reagent, were procured from Sigma Chemical Co. (St. Louis, MO, USA). The stock solution of patulin (1,000 ppb in distilled water) was prepared and diluted for the calibration curve. Apple juice (N Co., Seoul, Korea) was purchased from a supermarket in Jeongeup City, Korea.
To monitor the conditions for both reducing patulin content and minimizing the changes in the functional components of juice samples, RSM (Gontard et al., 1992) was used to observe the changes in the corresponding parameters (dependent variables) as affected by treatment conditions (independent variables), which were based on a central composite design (Kim et al., 2005; Lee et al., 2000) and the statistical analysis system (SAS, 1990). Independent variables, including storage temperature (X1, varying between −20°C and 20°C,), irradiation dose (X2, varying between 0 and 2 kGy), and storage period (X3, varying between 0 and 20 days), were coded at five levels (−2, −1, 0, 1, 2), and their values were chosen based on experiments for dependent variables (Yn), such as residual patulin content and polyphenolics content (Table 1). The central complete design consisted of 16 experimental points, including two points of center position (Kim et al., 2005; Lee et al., 2000).
Apple juice sample (50 mL) of a commercial product (N company) was bottled in a conical tube (polyethylene, PE), patulin (675 ppb) was injected and then irradiated with a cobalt-60 gamma irradiator (point source, AECL, IR-79, Nordion, Canada) at absorbed doses of 0, 0.5, 1.0, 1.5, and 2.0 kGy. The cobalt-60 source strength was approximately 100 kCi with 70 Gy/min dose rate at ambient conditions. The absorbed doses were monitored using free-radical and ceric/cerous dosimeters, and the actual dose ranges were within ±5.4% of the target doses. Apple juice samples were stored at −20, −10, 0, 10, and 20°C for 2 h before irradiation treatment, and then stored at the same temperature for 20 days after irradiation treatment and used in the experiment.
Analysis of patulin was carried out using the AOAC method (1996). In a tube, each sample from the gamma-irradiated and control groups was extracted by adding 50 mL of ethyl acetate. The extracts were mixed with 20 g of anhydrous sodium sulfate, allowed to stand at room temperature for 30 min, and filtered using a Whatman No. 4 filter paper (Sigma-Aldrich). The filtrate was concentrated at 40°C under a nitrogen stream, cooled at room temperature, and stored at −70°C in distilled water (pH 4.0).
Quantitative analysis of patulin was performed by high-performance liquid chromatography (HPLC) using a Waters Alliance 2690 (Waters Co., Milford, MA, USA) system equipped with a photodiode array detector (PAD, Waters 996, Waters Co.) HPLC was performed using a Shiseido column (3.9 mm i.d., 300 mm length), and the extracts were separated using a distilled water–acetonitrile (95:5) solvent at a flow rate of 0.7 mL/min. The column temperature was maintained constant at 25°C. The patulin peak was identified using a patulin standard by comparing its retention time. Detection was conducted by acquiring the PAD spectra of eluted compounds (200-400 nm). Based on chromatograms obtained at 276 nm. The entire UV spectra for all positive samples were compared with those of the external patulin standard solution analyzed under the same conditions. Control samples, along with the samples and calibration standards, were analyzed for each matrix.
The polyphenolic content was determined by the Folin-Ciocalteau colorimetric method (Gao et al., 2000). The extracts (0.9 mL) were mixed with 0.1 mL of 50 units/mL of ascorbic oxidase and then incubated for 90 min at 23°C to remove ascorbic acid. Next, the ascorbic acid-free extract (0.1 mL) was mixed with 0.2 mL of Folin-Ciocalteau reagent and incubated for 1 min at 23°C. After adding 3 mL of 5% Na2CO3 and incubating for 2 h at 23°C, the absorbance of the mixtures was recorded at 765 nm. The polyphenolic content was expressed as gallic acid equivalents.
Triplicate determinations were conducted at all design points in a randomized order. The corresponding extracts were subjected to analysis for dependent variables (responses), such as residual patulin content (Y1) and polyphenolics content (Y2). The mean values of triplicate determinations were analyzed to fit the following second-order polynomial models to all dependent Y variables. The following model was proposed for each response of Y:
where X1, X2, and X3 correspond to independent variables, i.e., irradiation/storage temperature, irradiation dose, and storage period; b0 is the intercept; and bn represents the corresponding regression coefficients (Gontard, 1992). A statistical analysis system from the SAS Institute (Cary, NC, USA) (SAS, 1990) was applied to predict models through regression analysis and analysis of variance. Response surfaces were developed using fitted polynomial equations. When the results demonstrated a saddle point in the response surfaces, the optimal conditions were determined using ridge analysis. Four-dimensional response surfaces were obtained using the Mathematica software (Wolfram Research, Champaign, IL, USA, 17).
3. Results and discussion
Table 1 shows the residual patulin content under the 16 experimental conditions set by the central composite design. The regression equations for the response variables (Yn) are listed in Table 2. R2 for the regression equation of Y1 was 0.9163, with a significance of <5% being recognized (p<0.05). The predicted peak point resulted in the lowest residual patulin content of 58.42 ppb with the corresponding independent parameters conditions, such as 18.19°C of storage temperature, 1.24 kGy of irradiation dose, and 13.42 days of storage period (Table 3). Patulin content of 58 ppb was desirable, representing a 91% decrease compared to the prediction of 675 ppb from the nonirradiated control sample (Table 3). Four-dimensional response surfaces for residual patulin content as affected by treatment conditions are depicted in Fig. 1, indicating that the residual patulin content decreased by the irradiation dose with the most predominant effect (p<0.01), whereas the effects of storage temperature and period on its reduction were nonsignificant (Table 4).
Condition | F-value | |
---|---|---|
Residual patulin content | Polyphenolics content | |
Storage temperature (°C) | 0.03 | 2.46 |
Irradiation dose (kGy) | 12.95** | 6.24* |
Storage period (day) | 1.27 | 6.87* |
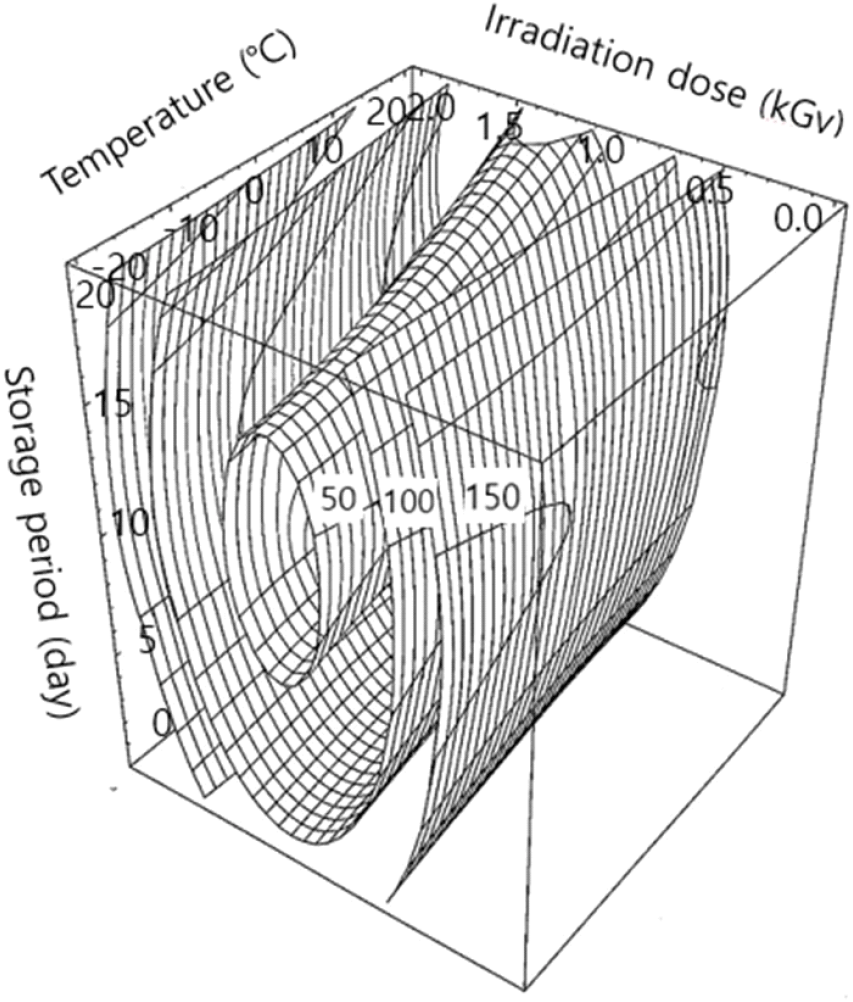
Patulin has been detected in apples and other fruits, such as strawberries, mangoes, grapes, bananas, and tomatoes. Damage to fruit skin, rotten raw fruits, or inappropriate technological processing steps results in fungal growth and the consequent patulin production. Physicochemical methods, including clarification, washing, filtration, and chemical treatment, were reported to reduce patulin content in apple products, including apple juice, effectively. However, gamma irradiation and subsequent storage is one of the most efficient methods to reduce patulin content in apple juice because it can be applied to the final packaged products in an automatic conveyor system without further contamination during the drying and/or packaging processes of the treated products. UV radiation inactivates primary microorganisms and reduces patulin content (Kitipong et al., 2012). If the initial patulin contamination level is approximately 1,000 ppb (mg/liter), the UV exposure, ranging from 14.2 mJ/cm2 (one pass) to 99.4 mJ/cm2 (seven passes), was successful in reducing patulin levels by 72.57% to 5.14%, respectively.
Polyphenolic compounds in apples are health-beneficial ingredients with antioxidant and antibacterial functions (Gardner et al., 2000). The conditions for minimizing the changes in polyphenolic content were investigated when applying gamma irradiation and subsequent storage at various temperature conditions.
The polyphenolic content of apple juice according to the designed conditions is shown in Table 1. The changes in polyphenolic content under three different treatment conditions are illustrated in Fig. 2. The regression equation for polyphenolic content (Y2) revealed an R2 of 0.9026; its significance was confirmed within a level of 5% (Table 2). The maximum polyphenolics content of apple juice was 11.98 mg/g under irradiation/storage temperature of 14.40°C, irradiation dose of 0.78 kGy, and storage period of 3.4 days (Table 3). The polyphenolic content was reduced to 9.79 mg/g when the storage period exceeded 15.13 days, even at a low gamma irradiation dose of 0.22 kGy (Table 3). The polyphenolic content of apple juice was significantly influenced by the irradiation dose and storage period (p<0.05, Table 4).
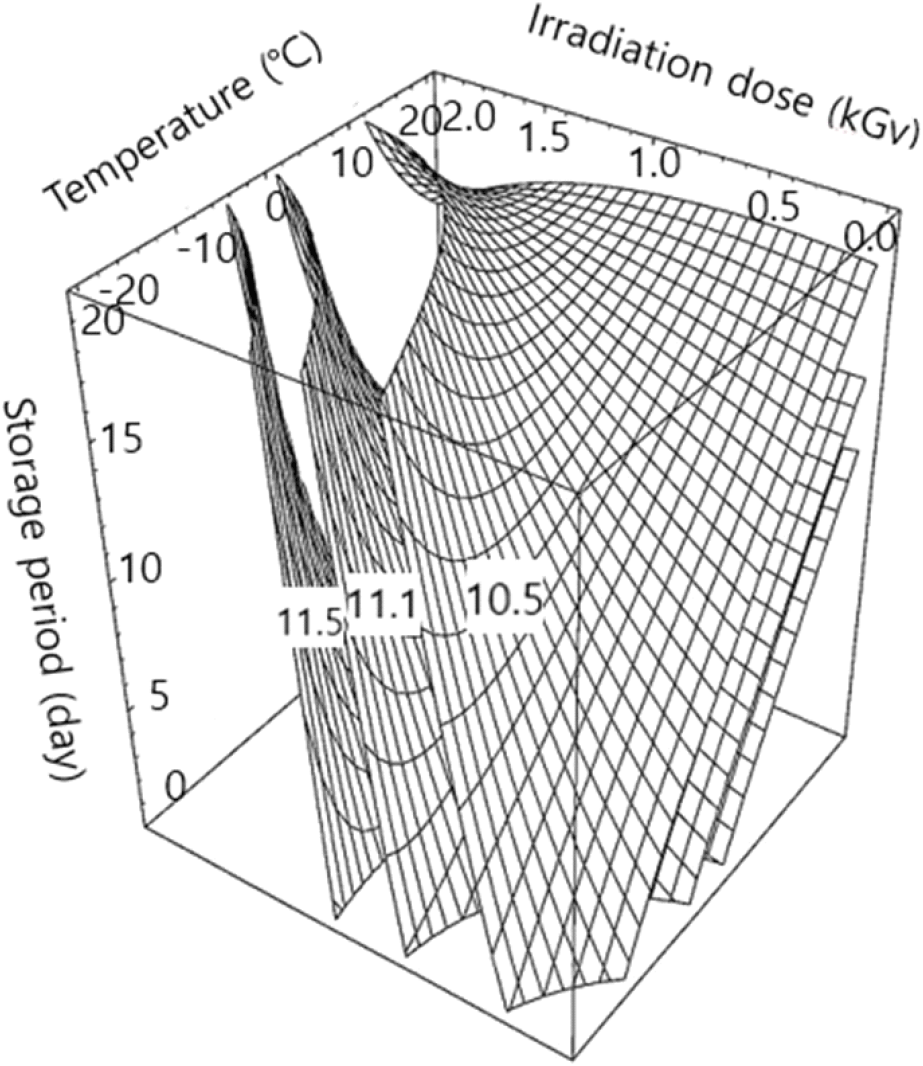
Possible mechanisms of the effect of radiation processing on phenolic compounds in mature, dry seeds of cereal and legumes are proposed, and higher irradiation doses also cause the breakage of the glycosidic bonds of phenolic compounds with carbohydrates (Shi et al., 2022). However, applied doses for apples or apple juices are too low to affect the glycosidic bonds of phenolic compounds.
4. Conclusions
Residual patulin and polyphenolic contents were monitored in apple juice under gamma irradiation and subsequent storage conditions to predict the most influential parameters for reducing patulin content in apple juice while maintaining its polyphenolic content by applying RSM. Based on the response surface models, irradiation dose (kGy) was predicted to be the most influential variable, followed by storage periods. The residual patulin content of 58.42 ppb is the minimum desirable level, representing a 91% reduction compared to the non-irradiated control (675 ppb). A maximum polyphenolics content (11.98 mg/g) was obtained under the predicted maximum conditions of 14.40°C, 0.78 kGy, and 3.4 days. Although further studies are needed on the mechanisms of patulin reduction in foods upon gamma irradiation, our results suggest that gamma irradiation may have potential applications in controlling patulin levels in foods, including apple juice.