1. Introduction
Sardines constitute a rich source of marine proteins and n-3 essential fatty acids, highly sought after by consumers for their health benefits (Hossain, 2011; Liu and Dave, 2022; Santos et al., 2023; Tsoupras et al., 2022). Generally, except for small quantities sold in a fresh state for direct consumption, sardines are mainly used for further processing. One of the factors limiting their commercial use in the fresh state is the difficulty of their conservation at low temperatures (Barbri et al., 2009). Thus, the short shelf life of fresh sardines is attributed to rapid bacterial spoilage and lipid oxidation, which can cause degradation of organoleptic characteristics, unpleasant odors, and color of the flesh (Tavares et al., 2021).
Consumers are increasingly aware of the benefits of choosing products that have undergone gentle conservation methods that preserve the maximum of nutrients while being practical, safe, permanently available, and of high quality (Guzik et al., 2022). In this sense, canned sardines constitute an ideal solution to respect this compromise. In this process, of great technological importance, sardines are packaged in metal cans or glass jars with different covering juices and condiments, sealed hermetically and sterilized by heat.
On the other hand, sardine oil is currently very appreciated for its prophylactic and therapeutic properties. Fish oil, which was considered a co-product of fish meal intended for animal feed, is now the primary source of polyunsaturated fatty acids (PUFAs), especially eicosapentaenoic acid 20:5 n-3 (EPA) and docosahexaenoic acid 22:6 n-3 (DHA) for human consumption (Bonilla-Méndez and Hoyos-Concha, 2018; Hossain, 2011; Mkadem and Kaanane, 2019; Otero et al., 2021; Rodrigues et al., 2024; Santos et al., 2023).
The objective of this study was the valorization of refined sardine oil as a covering juice in canned sardines. The chemical composition and nutritional quality of this new canned formula were determined.
2. Materials and methods
Sardines (Sardina pilchardus W.) were fished at the port of Dakhla by an RSW pelagic trawler during the month of February. Once recovered, the sardines were kept under ice after landing and during transport at a temperature of 3°C. Upon arrival at the fish cannery in Agadir, a sample of the sardines was used to determine the minimum and maximum size, fat content, temperature, and histamine levels (n=3).
The refined sardine oil was supplied by a company specialized in the production of refined fish oils. The salt used is fine sea salt. Tin cans of 125 mL capacity with Aluminized polyester and gold organosol BPA NIA varnish (104 mm, 59.8 mm, 27.8 mm) were used.
Canned sardine (Sardina pilchardus W.) formula (Fig. 1) was produced using 72% sardines, 27% refined sardine oil, and 1% sea salt. The canned sardines were packaged according to the production diagram illustrated in Fig. 2.
The canned sardines in refined sardine oil were produced in a fish cannery. The sardines were well-headed, gutted and tailed, washed before being placed in metal cans. The sardines were cooked in continuous cookers at 80°C for 30 min. Then, the covering oil and salt were added. The cans were seamed before being passed through the sterilization retorts. Finally, the cans were cooled to ambient air.
Canned sardines in refined sardine oil were analyzed to determine their protein, total lipid, ash, moisture, salt, and fatty acid contents. All these analyses were done for 100 g of net weight. The canned sardines were also analyzed to determine the histamine level and underwent bacteriological stability tests at 37°C and 55°C.
The acidity of the product was determined according to the ISO 660:2009 standard. The method involves dissolving a predetermined amount of fat in hot ethanol and subsequently titrating the resulting free fatty acids with a 0.1 N aqueous solution of sodium hydroxide, with phenolphthalein as an indicator. The resulting value is expressed as g of oleic acid/100 g of the product.
The primary oxidation products, specifically hydroperoxides, were analyzed using a validated method (ISO 3960:2007). The results were expressed in milliequivalents of active oxygen per kilogram. The analysis involved visual titration of released iodine using a starch indicator and a standardized solution of sodium thiosulfate.
The presence of secondary oxidation products was assessed using the para-anisidine method, which is in accordance with the international standard ISO 6885:2016. This analytical technique involves measuring the aldehydes present in the fatty substance. The aldehydic compounds react with para-anisidine, resulting in the formation of yellow-colored compounds that exhibit absorption at a wavelength of 350 nm.
The TOTOX index assesses the overall state of fatty acid oxidation, encompassing various forms of oxidation. This parameter is calculated by combining the peroxide value and the p-anisidine value using a weighted formula:
The fatty acid profile was determined according to the method used by Hammond (1986), previously described by Mkadem and Kaanane (2019).
The fat content was extracted from fish muscle and quantified gravimetrically using the Bligh and Dyer method (1959). Protein content was determined using the Kjeldahl method (AOAC, 2000). Similarly, the moisture level was measured according to the AOAC official method (2000).
The salt content was determined from the amount of chlorine after boiling the sample in nitric acid in the presence of excess silver nitrate, followed by titration with ammonium thiocyanate (Karl et al., 2002). The findings are presented as g of NaCl/100 g of the product.
For the analysis of histamine, 9 cans were tested using fluorometry, as described by the method of Lerk and Bell (1976), which involves the reaction of histamine with o-phthalaldehyde (OPA) to form a highly fluorescent compound, which is then measured using Trilogy Laboratory Fluorometer (Model 7200-002, Turner Designs Instrument, San Jose, CA, USA).
Three replicates were performed for each analysis (n=3). Results are expressed as the mean±standard deviation (mean±SD). In the exception of histamine in canned sardines, where the analysis was performed on nine cans, the average is also expressed as the mean±SD (n=9). Statistical analysis was performed using MiniTab V18.1.0.0 software (MiniTab Inc., State College, PA, USA) (Test version). The results were evaluated using a two-way analysis of variance (ANOVA) with a significance level of α=0.05 (n=3) to analyze the differences in the fatty acid profiles between refined sardine oil and canned sardines.
3. Results and discussion
The physicochemical characteristics of sardines (Sardina pilchardus W.) used are given in Table 1. Sardines were found to have a low-fat content (3.5%). They were caught in the coastal waters of Dakhla in February, which corresponds to the low-fat season. This is in agreement with the work of Mkadem and Kaanane (2020) and Bandarra et al. (1997), who confirmed the effect of season on the fat content of Sardina pilchardus, which could vary from 1.2% to 18.4%(w/w).
Items | Specifications |
---|---|
Species | Sardina pilchardus (Walbaum, 1792) |
Origin | Dakhla/Morocco |
Size range (cm) | 18.6 to 22.1 |
Temperature (°C)1) | 0.9±0.1 |
Histamine (ppm)1) | 21.1±0.7 |
Fat (%)1) | 3.5±0.3 |
The size of sardine individuals ranged from 18.6 cm to 22.1 cm. This size corresponds well with that of mature individuals, as it exceeds the minimum length of 17 cm reported by Cergole (1995). A recent study showed that fishing season and fish size have a significant effect on the lipid content of Sardina pilchardus caught in the Atlantic Ocean, off the coast of Tantan, Morocco. Specifically, sardines from the low season (January-March) had an average lipid content of 4.4 ±0.9% for large sardines and 3.8±1.9% for small ones, while large sardines from the high season (July-September) had a lipid content of 9.2±0.7% and 6.7±1.6% for small ones (Aoua et al., 2024).
Sardines are particularly prone to histamine production due to their high histidine content in their muscles, which can lead to histamine fish poisoning (Afilal et al., 2006; Mahmoud et al., 2023; Soldo et al., 2019). Histamine is a biogenic amine known to trigger various allergic and inflammatory reactions, as documented by several studies (Bose et al., 2023; Khatouf et al., 2024; Oktariani et al., 2022). Upon death, fish stored at temperatures above 4°C for extended periods can undergo histamine production through the activity of histamine-forming bacteria. These microorganisms produce the enzyme called histamine-decarboxylase, which converts histidine amino acid into histamine (Mahmoud et al., 2023; Mohamed et al., 2022; Visciano et al., 2012). The presence of histamine in food is often an indicator of spoilage, highlighting the importance of proper storage and handling practices to prevent its formation (Naila et al., 2012).
In this study, the histamine level in the studied sample was equal to 21.1 ppm. This rate is considered low compared to the limit set by Moroccan Ministerial decree No. 293-19 of 9 Joumada II 1440 (February 15, 2019) and by the European Union, Commission Regulation (EC) No 2073/2005 of 15 November 2005 on microbiological criteria for foodstuffs, which fix concentration limits for nine fish samples. Accordingly, the average content must not exceed 100 mg/kg, two samples may exceed 100 mg/kg but not reach 200 mg/kg, and no sample should exceed 200 mg/kg in canned fish.
This level in fresh sardines is considered low thanks to maintaining transport and storage conditions at a low controlled temperature (0.9°C±0.1 in the heart of the fish). All studies recommend keeping fresh fish at a temperature below 0°C to prevent histamine formation, as it is negligible at this temperature range. However, histamine can form in fish that are exposed to temperatures above 4°C during or after capture, potentially reaching toxic levels (El Marrakchi et al., 1990; Evangelista et al., 2016; Khatouf et al., 2024; Mahmoud et al., 2023; Mohamed et al., 2022; Sardar et al., 2015). Several studies have demonstrated the significant impact of storage temperature and duration on histamine content in fish muscle (Chang et al., 2008; Khatouf et al., 2024; Mohamed et al., 2022; Pacheco-Aguilar et al., 2000; Shakila and Vasundhara 2001; Vijayan et al., 1994). Storing sardines at low temperatures (below 0°C) also improves their shelf life (El Marrakchi et al., 1990; Sardar et al., 2015). Other factors that can influence the histamine content in fresh sardine flesh include species, fishing season, fish size, and storage duration. For example, a strong correlation was found between fishing season and fish size on the histamine content of Sardina pilchardus from the Tantan coast in Morocco, as demonstrated by Aoua et al. (2024). The average histamine levels in fresh sardine flesh ranged from 14.7±4.5 to 17.9±2.1 mg/kg.
The physicochemical characteristics and fatty acid profile of refined sardine oil are given in Table 2. The sardine oil used has an acidity, a peroxide value, and a p-anisidine value equal to 0.28%, 3.07 (mEq active oxygen/kg oil), and 4.19, respectively. The TOTOX value is found to be 10.33. These results comply with CODEX STAN 329-2017 standard for fish oils intended for human consumption.
This oil is also rich in PUFAs, accounting for 36.16%, followed by saturated fatty acids (SFAs) at 31.84% and monounsaturated fatty acids (MUFAs) at 22.95%. The most abundant fatty acids are eicosapentaenoic acid (EPA; C20:5 n-3) at 20.21%, palmitic acid (C16:0) at 19.02%, oleic acid (C18:1 n-9) at 10.16%, docosahexaenoic acid (DHA; C22:6 n-3) at 8.35% and palmitoleic acid (C16:1 n-7) at 8.33%. The fatty acid profile of refined sardine oil is typical and similar to that already obtained by Mkadem and Kaanane (2019) for crude oil extracted from by-products of Sardina pilchardus.
Sardine oil, rich in omega-3 fatty acids, particularly EPA and DHA, has been recognized for its numerous health benefits due to its favorable fatty profile, which is particularly rich in EPA and DHA. This unique fatty acid composition provides numerous advantages, including improved cardiovascular health, reduced inflammation, and enhanced brain function (Balta et al., 2021; Nassar et al., 2023). Additionally, sardine oil has been found to have a higher antioxidant capacity, which can help protect against oxidative stress and cell damage (Borges et al., 2014). Overall, the favorable fatty profile of sardine oil makes it an excellent source of essential nutrients for human health.
The results of histamine analysis of 9 cans of sardines in refined sardine oil are presented in Table 3. The presence of histamine in food items is an indicator of the quality of the food. The average analysis of the histamine level in 9 cans of canned sardines in refined sardine oil is equivalent to 23.90 ppm. This value complies with the above-mentioned regulatory limits for fish products related to a high content of histidine. This value is also in compliance with the regulations applied in the United States, the Food and Drug Administration (FDA), which has proposed a strict standard for histamine levels in fish, aiming to reduce the limit from 50 to 35 ppm. This stricter regulation is more conservative than the internationally recognized limit of 100 ppm (FDA, 2021). The histamine value in canned sardines provides information on the degree of freshness of the sardines used in processing, as there is a positive relationship between the histamine levels and sensory scores (Khatouf et al., 2024; Mohamed et al., 2022).
Samples | Contents |
---|---|
Can 1 | 23.64 |
Can 2 | 24.01 |
Can 3 | 24.16 |
Can 4 | 23.77 |
Can 5 | 23.97 |
Can 6 | 23.84 |
Can 7 | 24.10 |
Can 8 | 23.83 |
Can 9 | 23.78 |
Histamine average1) | 23.90±0.17 |
Our results are consistent with those reported by El Hariri et al. (2017), who evaluated the histamine levels of canned sardines marketed in Morocco from 2013 to 2015. The synthesis of the data revealed that the occurrence of histamine is low, with a non-compliance rate of 2.02% on 323 samples analyzed. The average histamine values of all batches analyzed did not exceed 5.63 mg/100 g. Average values were 5.14 mg/100 g for canned sardines. These levels are lower than the regulatory safety limit (El Hariri et al., 2017).
Whether the histamine level in fresh sardines is influenced by duration and storage temperature. After canning, histamine levels remain stable. Monitoring of histamine in fresh sardines (Sardina pilchardus) directly after fishing was found to be 0.10 ppm. This rate increased to 0.21 ppm after cooking. It was maintained stable at 0.23 ppm in canned sardines after 6 months of storage (Selmi et al., 2008). The same observation was reported by other researchers (López-Sabater et al., 1994; Veciana-Nogués et al., 1997).
According to NM 08.0.144, the limit of pH difference between cans incubated at 37°C and 55°C, compared to the pH of a can incubated at room temperature, must be less than 0.5. In our study, the pH difference did not exceed 0.3 for all the incubations carried out. Likewise, no deformation or visual anomaly was noticed for the different cans tested. Thus, the product is assessed to be bacteriologically stable. According to Heldman and Hartel (1998), all foods with pH greater than 4.5 (case of canned sardines in oil) must undergo a minimum thermal process to ensure the destruction of Clostridium botulinum, a pathogen known for its resistance to heat. The severity of this process depends on the specific thermal resistance characteristics exhibited by the bacterium. The recommended minimum process is determined based on thermal death time versus temperature data, with a commonly used reference process being 2.45 min at 121°C for this purpose. This heat treatment ensures bacteriological stability and commercial sterility regardless of the storage temperature (Heldman and Hartel, 1998).
Thermal treatment guarantees food safety but can have negative effects on nutritional quality. According to Horner (1997), the advantage of effective sterilization is obviously minimal overcooking and maximum heat transfer to the coldest point of the product. According to Kiziltas et al. (2010), the level of heat necessary for optimal sterilization is influenced by various factors such as the dimensions of the container and its type of metal, the type of product and its covering medium (Ali et al., 2005; Heldman and Hartel, 1998; Olusola, 2018) and the pH of the product where the sterilization time of low acid foods can be reduced by heat at a higher temperature, the initial temperature of the product, and by the heating medium (steam or hot water). After the sterilization process, the cooling process should be immediately carried out to avoid overcooking (Ogbulie et al., 2014), thus enabling the rapid return of the seal to its normal state and avoiding micro-leakage.
The results of the biochemical composition of canned sardines in refined sardine oil are given in Table 4. Canned sardines in refined sardine oil consist mainly of water, fat, and then proteins. These results also show their high lipid content, which is due to the use of oil as a covering juice but also to the loss of water by evaporation during cooking (Manthey-Karl et al., 2014; Saguy and Dana, 2003). The same effect has been reported for canned sardines in tomato sauce (Selmi et al., 2008). Cooking causes water loss and, consequently, an increase in dry matter levels, including lipids (Garcia-Arias et al., 2003; Kocatepe et al., 2011). According to Sainclivier (1983), water losses during cooking are proportional to the size of the fish. In addition, these losses are closely linked to the biochemical composition of fish, particularly in proteins and lipids (Garcia Arias et al., 2003).
Components | Contents |
---|---|
Moisture | 51.40±0.921) |
Fat | 27.87±0.39 |
Protein | 17.91±0.29 |
Salt | 1.03±0.05 |
Heat treatments are all known for their role in reducing moisture and increasing protein, fat, and ash content (Garcia-Arias et al., 2003; Weber et al., 2008). In our case, sterilization of cooked sardines, which are hermetically sealed in cans, generates a release of the water contained in the muscles of the sardines into the covering medium. Consequently, it does not influence the moisture of the total net weight of the product since the water is not evaporated, it remains contained in the can. This explains the high level of moisture in studied canned sardines, which is around 51.40%. Compared to other studies, moisture levels of around 52.41 and 53.07% were analyzed in canned sardines (El Shehawy and Farag, 2019). In canned sardines in oil taken from the Turkish market, the average moisture was equal to 60.6 g/100 g of net weight (Manthey-Karl et al., 2014). Higher rates were obtained for canned Brazilian sardines (Sardinella brasiliensis), whose moisture content varied between 63.85 g/100 g to 65.09 g/100 g (Tarley et al., 2004). El-Dengawy et al. (2012) reported that moisture percentages in canned fish range from 52.41 to 78.53%.
The protein content is around 17.91%. This rate is low compared to the percentage of proteins in fresh sardines (Mkadem and Kaanane, 2020). This protein level depends on the percentage of sardines used in the product. Sardines have long been recognized as a valuable source of high-quality protein in the human diet (Manthey-Karl et al., 2014; Puwastien et al., 1999). A similar protein content was found in canned sardines in oil collected from the Turkish market, around 18.6 g/100 g net weight (Manthey-Karl et al., 2014). While higher values were obtained in canned sardines of around 22.0 g/100 g and 24.4 g/100 g by Tarley et al. (2004). Different canned sardines in soybean oil, marketed in Brazil, were the subject of an analysis of their chemical composition. The protein levels were comparable to those typically observed in fresh sardines, with a range of 19.8-24.4%. The American Heart Association (AHA) recommends consuming at least two servings of fish per week for their cardio-protective effects (Krauss et al., 2000). In comparison, the beneficial properties of fish are often attributed to their fatty acid composition (Mataix and Gil, 2004), research has suggested that fish protein may also contribute to these benefits (Vazquez and Sanchez-Muniz, 1994). The proteins in canned sardines are characterized mainly by their high digestibility (Hariyadi, 2000).
In our study, the percentage of salt is equal to 1.03%. This value is considered low, allowing people suffering from high blood pressure and cardiovascular diseases to consume canned fish but in moderation. The levels obtained in our study are close to those evaluated in canned sardines in oil in Turkey (1.2 g/100 g net weight) (Manthey-Karl et al., 2014). Simultaneously, El-Dengawy et al. (2012) reported NaCl contents varying between 0.13% and 1.2% in canned fish. Salt added to canned fish is mainly intended to improve its sensory properties, specifically enhancing its flavor, texture, and color, making it more palatable to consumers (Featherstone, 2015; Hutton, 2002).
Table 5 presents the fatty acid profiles of canned sardines in refined sardine oil. The most abundant fatty acids found in canned sardines are the same ones identified in the covering oil. These are EPA (C20:5 n-3), palmitic acid (C16:0), oleic acid (C18:1 n-9), myristic acid (C14:0) and DHA (C22:6 n-3). These acids are characteristic of sardine fat and sardine oil. The same distribution was obtained in the flesh of fresh Sardina pilchardus by Mkadem and Kaanane (2020) and in the oil recovered from Sardina pilchardus by-products (Mkadem and Kaanane, 2019).
When vegetable oils are used as covering oils in canned fish, the observations are different. The quantity and type of covering oil used affect the fatty acid profile due to the interaction between the lipids of sardines and those of the covering oil (Aubourg et al., 1996; Badolato et al., 1994; Caponio et al., 2003; Rossi et al., 2001; Tarley et al., 2004). The same observation was reported for canned sardines in tomato sauce, where the covering oil was absorbed by the sardine during sterilization, leading to a higher content of oleic acids and linoleic in sardine flesh (Selmi et al., 2008). Different canned sardines (Sardinella brasiliensis) in soybean oil, marketed in Brazil, were the subject of an analysis of their fatty acid composition. The highest levels were noted in C18:2 n-6 and C18:3 n-3 acids. While the concentrations of EPA and DHA ranged from 5.39 to 15.1% and 3.89% to 9.51%, respectively (Tarley et al., 2004). In general, marine fish lipids are characterized by low concentrations of linoleic and linolenic acids, which are characteristics of soybean oil (Ivanov et al., 2010). On the other hand, the detected values of long-chain fatty acids in the covering oils indicate a diffusion of lipids contained in fish flesh towards the covering oils (Caponio et al., 2003; Caponio et al., 2011; Rossi et al., 2001; Tarley et al., 2004). This finding can be attributed to both the proportion of fish to oil and the fat level in fish (Pellegrino and Tortonese, 1982).
The fish species, cooking method, and formulas used are also determining factors for the essential fatty acid content of the products consumed (Gladyshev et al., 2006). Selmi and Sadok. (2007) studied the effect of the canning process on the fatty acid profile of Sardina pilchardus. The results showed that the difference between the lipid composition of the sardine in the fresh state, in the cooked, and in the canned state was related to the initial fatty acid composition of the sardine, the heating process, and the packing medium used. Caponio et al. (2011) investigated the fatty acid composition and degradation level of covering oils used in canned fish products. Their results showed that the covering oils contained fatty acids characteristic of fish, such as EPA and DHA, which was consistent with previous studies (Osman et al., 2001; Selmi et al., 2008; Tarley et al., 2004). This finding confirmed earlier reports on lipid exchange between fish and covering oil (Bizzozzero and Carnelli, 1996; Cavallaro et al., 1996; Rossi et al., 2001; Vitucci et al., 1999). Moreover, the fatty acid composition of the liquid medium was influenced by the type of fish due to fish lipid diffusion into the covering oil, with sardines being the most significant contributor to this variability (Caponio et al., 2011).
In the present study, canned sardines in sardine oil contained 10.35% PUFA, 8.86% SFA, and 6.27% MUFA based on the net weight of the product (Fig. 3). Different distributions of fatty acids have been reported in the literature. Canned sardines in oil were composed of 45.5% PUFA, 25.3 SFA, and 24.6% MUFA (El Shehawy and Farag, 2019).
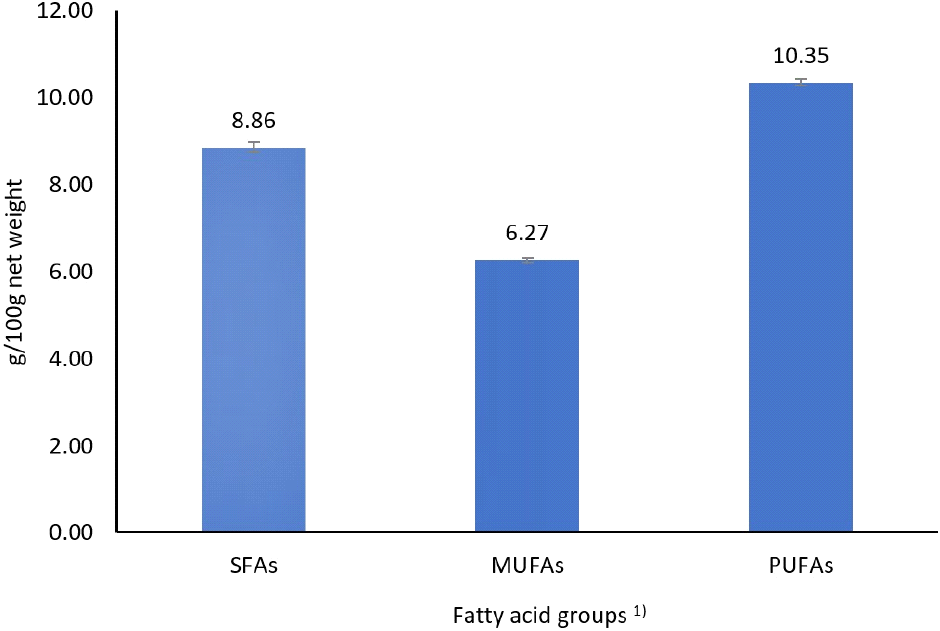
The study by Caponio et al. (2011) on 11 recipes for canned sardines showed an average distribution of 47.80% MUFA, 30.17% PUFA and 21.41% SFA. These differences were mainly explained by the fatty acid characteristics of the covering oil used. The same observation has been reported by several authors (Aberoumand et al., 2011; Caponio et al., 2011; Naseri et al., 2011; Novita Herawati et al., 2016; Selmi et al., 2008; Sengor et al., 2008). Compared to canned tuna, mackerel, and anchovies, canned sardines were the richest in long carbon chain PUFA (Caponio et al., 2011).
Oils used as covering juice in cans that contain neither DHA nor EPA reduces the content of these fatty acids in the total net weight of the product compared to their concentration in the oil alone. Selmi and Sadok. (2007) published that the EPA/DHA ratio decreased from 0.19 in the flesh of fresh sardines to 0.17 in the flesh of sardines canned in tomato sauce. This reduction is also expressed by the drop in EPA and DHA contents, which went from 6.24% to 4.15% and from 33.61% to 24.79%, respectively.
A can of sardines in refined sardine oil provides n-3 PUFA contribution of around 9.26 g/100 g, and a sum of EPA and DHA acids equals to 8.88 g/100 g (net weight). The use of refined sardine oil provides a significant intake of n-3 type essential fatty acids. Fish lipids are known to be beneficial to human health based on their richness in EPA and DHA (Arts et al., 2001; Broadhurst et al., 2002; Calder, 1997; Kinsella, 1986; Leaf and Weber, 1988). According to the American Heart Association, individuals should consume a daily intake of 500 mg of EPA+DHA (Kris-Etherton et al., 2002). Only half a can of sardines in refined sardine oil is sufficient to cover daily needs.
The ratio of PUFA to SFA is a useful indicator for identifying healthier food options. As per the latest recommendations from nutritionists and healthcare professionals, an optimal diet should comprise one-third SFA, one-third MUFA, and one-third PUFA. If this objective is achieved, the PUFA/SFA ratio in dietary lipids should be around 1 (Zdzislaw and Kolakowski, 2003). Kang et al. (2005) recommended a PUFA/SFA ratio of 1.0-1.5 in the diet to reduce the risk of cardiovascular diseases and oxidative stress. The value of PUFA/SFA ratio obtained in the present work was 1.17 for canned sardines in sardine oil. This ratio is considered ideal for health consumers. Selmi and Sadok (2007) reported that following the use of tomato sauce, whose PUFA/SFA ratio is equal to 1.79, the PUFA/SFA ratio increased from 1.19 in the flesh of fresh sardines to 1.52 in the flesh of sardines used in cans with tomato sauce. Although increasing the PUFA/SFA ratio is important, having a generous ratio of n-3 PUFA/n-6 PUFA is highly desired. In our study, this ratio is equal to 18.5 for those in refined sardine oil.
A diet with a high PUFA/SFA ratio is recommended for the prevention of cardiovascular diseases and several health issues (Balta et al., 2021; Nassar et al., 2023). However, this diet may also increase oxidative stress due to the inherent sensitivity of PUFAs to lipid peroxidation (Kang et al., 2005; Lee et al., 1989; Park et al., 1999). To balance these concerns, the proportion of refined sardine oil can be adjusted or blended with other vegetable oils to achieve the desired levels of PUFAs, EPA, and DHA in canned sardines.
4. Conclusions
In a context marked by scarcity of resources, the evolution of consumers’ lifestyles, and the development of a culture that prioritizes products based on their nutritional contribution and their environmental impact, the valorization of refined sardine oil in the production of canned sardines constitutes an ideal, practical and safe solution to achieve good dietary balance, a source of taste pleasure, sustainable availability, and high nutritional quality. The results of this study showed that canned sardines contained 51.40 g of moisture, 27.87 g of fat, 17.91 g of protein, and 1.03 g of salt per 100 g net weight. The fatty acid composition included 10.35% polyunsaturated fatty acids, 8.86% saturated fatty acids, and 6.27% monounsaturated fatty acids, with EPA, palmitic acid, oleic acid, myristic acid, and DHA being the most abundant fatty acids. This recipe provided 8.88 g/100 g (net weight) of EPA and DHA.
The use of refined sardine oil in canned sardines, produced without additives, allows for a high contribution in marine proteins and essential fatty acids type n-3, which are highly appreciated by consumers given their interests for human health. The findings support the interest in using refined fish oil to enrich foods with essential marine fatty acids. This work leads to encouraging prospects for sardine canneries. Indeed, the valorization of refined sardine oil in canned fish turned out to be simple, and the results obtained suggest a promising future for these high-added-value products. The production of canned sardines with refined sardine oil can be a competitive advantage for sardine canneries, allowing them to differentiate themselves from other products on the market.
Overall, this study highlights the potential benefits of using refined sardine oil in canned sardines and suggests further research to explore its applications, consumer acceptance, and potential uses. To achieve this, it’s proposed to explore its use in other food products, conduct sensory evaluations of refined sardine oil in canned sardines, and analyze its economic feasibility and competitiveness in the market.