1. Introduction
Anchomanes difformis (forest anchomanes) belongs to the Araceae family and it regarded as a herbaceous plant. It is sometimes referred to as the forest ‘Anchomanes’ in English (Croat and Ortiz, 2020). However, it is known as “Abirisoko” or “igo” among the Yoruba-speaking tribes of southwestern Nigeria. It contains an appreciable starch content of 21%, which is comparable to other starch sources (Oyedele et al., 2020).
Starch is a natural biodegradable bio-polymer widely used in many industries (Afolayan et al., 2020). Its diverse industrial usage is a result of its availability at low cost, high calorific value and great functional properties (Diyana et al., 2021; Waterschoot et al., 2015). Starch is a semi-crystalline carbohydrate that can be found in grains, roots, rhizomes, leaves, seeds and tubers of most plants (Phukan and Nongkhlaw, 2022).
Native starches are commonly consumed as food, but unsuitable for many applications due to their poor ability to withstand processing conditions (Singh and Chudasama, 2020), hence, the need for modification. Modified starch is a native starch whose physical and chemical properties have been altered in order to improve its functional characteristics (Özden and Sönmez, 2019; Raji et al., 2020). Interestingly, starch modification is carried out to starch granules’ stability during processing and makes it suitable for various foods and industrial applications (Raji et al., 2020). The properties of starch that are altered during modification are retrogradation behavior, association behavior, viscosity, gelatinization properties, pasting properties, and shelf-life stability of final products (Raji et al., 2020). Modified starch can resist amylase digestion in the small intestine to some varying degrees, and it is broken down to short-chain fatty acids by the activities of microflora in the large intestine (Sorndech et al., 2018). The derivatives obtained from the modification of starch are useful in plastic, food, cosmetics, textiles, adhesives, pharmaceutical, and paper industries (Adewale et al., 2022).
On the other hand, succinylation is a chemical modification process of reacting starch with succinic anhydride (Raji et al., 2020). However, succinylated starch possesses high viscosity, low gelatinization temperature, high paste clarity, better paste stability, high thickening power and good film-forming properties (Chakraborty et al., 2022). Noteworthily, the addition of starch succinate to the diet reduces dietary energy density and depletes the digestion of foods, thereby making food products developed from stanch succinate desirable for people suffering from diabetes (Ahmad et al., 2021; Wang et al., 2020). Since systematic studies on the succinylation of Anchomanes difformis starch are scanty, this study is designed to examine the effects of succinic anhydride on Anchomanes difformis starch and to evaluate the effect of succinylation on the modified starches’ qualities.
2. Materials and methodology
Anchomanes difformis tubers were collected from Omu-aran, an Area of Kwara state, Nigeria. The samples were kept in an open space in the laboratory prior to starch extraction.
The method of Emeje et al. (2012) with slight modifications was used for the starch isolation as described in Fig. 1. Anchomanes difformis tubers were peeled, washed with clean water and chopped into smaller sizes (1.0 cm thick). The slices were blended in a hammer mill for about 2-3 mins. The slurry obtained was sieved with a muslin cloth and further soaked in 1.5 L of 0.03 M NaOH solution for 1 h. The supernatant was decanted and the residue was neutralized with 0.5 M HCL solution to a pH of 6.0. The neutralized residue was washed three times with distilled water and centrifuged at 1,500 rpm for 5 min. The wet starch obtained was thinly spread on trays and dried in a cabinet drier at 50°C for 12 h.
A simple lattice design of the Design Expert version 6.0 was used to investigate the effects of two independent process variables namely, the percentage of succinic anhydride (A) and percentage of starch (B) on the responses (FTIR, functional properties and pasting properties of the samples). The variable ranges, as shown in Table 1, were selected based on the literature (Raji et al., 2020). However, succinylated starches were prepared using the method of Awokoya et al. (2011) with some modifications as described below:
Component A succinic acid (%) | Component B starch (%) |
---|---|
0.00 | 100.00 |
3.00 | 97.00 |
6.00 | 94.00 |
9.00 | 91.00 |
12.00 | 88.00 |
The purified Anchomanes difformis starch was succinylated at ambient temperature (30°C) using the method of Awokoya et al. (2011). Starch (100 g) was dispersed in 300 mL of distilled water and stirred magnetically for 1 h, after which it was adjusted to a pH 11.0 using 0.03 M NaOH solutions. The samples were succinylated using succinic anhydride at 0, 3, 6, 9, and 12% and the mixtures were stirred gently using a magnetic stirrer for 2 h. At the end of the reaction, the pH of each slurry was adjusted to pH 6.0 using 0.03 M NaOH solution, filtered, washed with distilled water six times and dried in a cabinet drier at 50°C for 12 h, milled and kept in an airtight jar to prevent re-absorption of moisture (Fig. 2).
The swelling power, water absorption capacity, oil absorption capacity, bulk density, solubility index and gelation temperature of both purified and succilynated Anchomanes difformis starch samples were determined using Afolayan et al. (2020) methods.
The pasting properties of the starch samples were determined using a Rapid Visco Analyzer (RVA model RVA 3D+, Newport Scientific PTY Ltd, Warriewood, Australia) as described by Badejo et al. (2017).
The IR spectra of the starch samples were run as KBr pellets on the FTIR system (Spectrum BX PerkinElmer, Pittsburgh, England) in the frequency range 350-40,000 c/m as described by Gbenga et al. (2014).
The moisture contents, fat contents, ash contents, crude fiber contents, protein contents and carbohydrate contents, and both the purified and succilynated starch samples were determined following AOAC (2005) methods.
The tannin contents, oxalate contents, phytate contents, and saponin contents of both the purified and succinylated starch samples were determined following AOAC (2005) methods.
The total amylose content of both the purified and succinylated starch samples was determined iodimetrically following the standard procedure described by Morrison and Laignelet (1983), while the percentage of amylopectin was determined by subtracting the percentage of amylose content from the amount of carbohydrate in the sample.
The procedure described by Kwon et al. (2008) was adopted for the determination of α-amylase inhibition assay with slight modification.
Kwon et al. (2008) method with slight modification was used for the determination of α-glucosidase inhibition assay with slight modification.
The pancreatic lipase inhibitory activity of samples was determined according to the protocol reported by Eom et al. (2013), in which Anchomanes difformis starch was used as a reference inhibitor of pancreatic lipase.
3. Results and discussion
The gelatinization temperature, solubility index, bulk density, swelling power, oil absorption capacity and water absorption capacity ranged from 62.55-80.50°C, 1.08-2.22%, 0.54-0.73%, 2.07-4.12%; 113-176% and 206-259%, respectively (Table 2). The water absorption capacity (WAC) can be expressed as the ability of a sample to absorb water and it is an important property of starch that determines its hydration potential in hot water or during gelatinization (Awoyale et al., 2020). The highest WAC (259%) observed in the succinylated starch showed that it might absorb more water during its utilization than native starch which had the lowest WAC (206%). Succinylation of Anchomanes difformis starch in this study resulted in an increase in WAC of its starch, and this might be due to a reduction in starch crystallinity as a result of succinylation, which allowed water to migrate into the starch granules (Raji et al., 2020). Similar findings were reported by (Arueya and Oyewale, 2015) who observed an increase in WAC of succinylated acha starch samples (155-205%). The observed higher oil absorption capacities in the Anchomanes difformis starch succinates implied that they might have better emulsion stability than the native starch. However, the oil absorption capacity of starch can be improved by chemical modification and this improvement is due to the incorporation of functional groups in the starch molecules, thereby giving it a higher binding capacity than the native starch (Olu-Owolabi et al., 2014; Raji et al., 2020).
A slight increase in the swelling capacities of the samples was observed. The native starch possessed the lowest swelling capacity, while the sample that was succinylated with 12% succinic anhydride had the highest swelling capacity. The increment in the swelling capacity of the modified sample might be a result of the formation of the succinyl group which reduced the intermolecular hydrogen bonds and also strengthened the long chains of amylopectin with the simultaneous decline in amylose content (Ikegwu et al., 2009; Raji et al., 2020). However, higher swelling capacities of the starch succinates depict that they may reconstitute better in water compared to the native starch.
The bulk density (BD) is an indicator of food product porosity and it determines both the choice of packaging materials and packaging design (Majhi et al., 2019). It was observed that succinylation significantly influenced the bulk densities of the starch succinate samples. Lower bulk density values were recorded in the succinylated samples when compared with the purified starch that was not succinylated. However, lower bulk densities are advantageous and economical, as it will reduce the cost of distribution during marketing (Falade and Oyeyinka, 2015). Interestingly, a diet possessing lower bulk density is needed by infants for easy swallowing without experiencing choking or suffocation (Raji et al., 2022). This shows that both infants and adults can consume the starch succinate. Therefore, the findings from this research will provide adequate information on how best to package Anchomanes difformis starch succinate.
It was revealed that the solubility index of the starch samples decreased up till 6% succinylation level and increased moderately thereafter. Interestingly, less water solubility values were exhibited by starch succinates than the native starch. However, the behavior might be a result of starch structure reorganization, which contributed to the weakness of the granules of starch during succinylation. A similar finding was reported by Arueya and Oyewale (2015) who researched the effect of succinylation degree on acha starch. It was observed that as the succinylation levels increased, the gelatinization temperatures decreased. The gelatinization temperatures (62.55-66.57°C) reported for 9% and 12% levels of succinylation fell within the ranges Segura-Campos et al. (2008) reported for octenylsuccinic starch from Phaseolus lunatus and Raji et al. (2020) established for starch succinates from Dioscoria villosa. The lower gelatinization temperature is advantageous, as such a sample can be used in food processing operations where a lower temperature is needed for thickener to gel. Also, energy might be conserved in this type of process.
The detection of the functional group of active components can be achieved via the FT-IR spectrum (Raji et al., 2020). Anchomanes difformis modified starch samples exhibited typical Fourier Transform Infrared (FT-IR) spectra absorption bands of starch backbones. A similar spectrum was also observed in the native starch FT-IR spectrum. However, characteristics bonds occurring at 3,383.03 cm−1, 3,382.47 cm−1, 3,382.59 cm−1, 3,383.56 cm−1 and 3,382.77 cm−1, which corresponded to the O-H stretching vibration were obtained at succinylation levels of 0%, 3%, 6%, 9%, and 12% respectively. The band ranges in Anchomanes difformis starch samples were slightly lower than the absorption range for O-H vibration which was 3,500-3,700 cm−1. A shift in the O-H stretching peaks to a lower wavelength was noticed and this might be a result of intermolecular hydrogen bonds in the glycosidic ring that weakened the O-H bond. In addition, the presence of carbohydrates in the samples was confirmed through the O-H stretching. Similar results were reported by Arueya and Oyewale et al. (2015) who evaluated the FT-IR spectrum characteristics of succinylated acha starch and Raji et al. (2020) who researched the FT-IR spectrum characteristics of succinylated wild yam starch.
The peak viscosity, which is the maximum viscosity developed during or soon after the heating portion (Sanni et al., 2001), ranged from 189.08 to 270.58 RVU (Table 3). The succinylation level had a significant effect on the pasting properties of the samples (p≤0.05). The peak viscosity values variation might have a direct link with the starch samples amylose contents. High starch content is an indication of high peak viscosity and high water binding capacity of starch (Oyeyinka et al., 2019; Raji et al., 2020). Native starch displayed a lower peak viscosity when compared with the starch succinates. The values reported for the modified starch samples were within the range of values (195.17-399.58 RVU) established by Ezeocha and Okafor (2016) for D. dumetorum. The moderately high peak viscosities of the succinylated starch samples showed that the succinylated granules might likely become more rigid or less elastic and be able to withstand the shearing influence during food processing.
The trough viscosity of the native and succinylated starches varied from 102.71-114.04 RVU (Table 3). The sample that was succinylated at 3% had the lowest value, while the native starch sample had the highest value. The level of succinylation significantly influenced the trough viscosity of the starch samples. However, the values obtained fell below the range of values (133.45-206.60 RVU) established by Arueya and Oyewale (2015) for acha starch succinates. Their paste might possess a slightly lesser ability to withstand breakdown during cooling due to their moderate trough viscosity values (Raji et al., 2020). Worthily, the low trough viscosity recorded in some starch samples implied that the starch samples might be applicable to food systems where moderate paste stability during cooling is required (Adegunwa et al., 2015).
The breakdown viscosity ranged from 75.04-161.45 RVU. The highest value was observed in the sample that was succinylated at 3%, while the lowest breakdown viscosity was recorded in the native starch (Table 3). The level of succinylation significantly influenced the breakdown viscosity. Adebowale et al. (2005) reported that the lower the sample`s ability to withstand shear stress and heating during cooking, the higher the breakdown viscosity. Hence, it is indicative that the native starch might possess the ability to withstand heating and shear stress than the starch succinates due to their low breakdown viscosity values.
The final viscosity values varied from 141.04-194.92 RVU, with the sample that was succinylated at 9% having the lowest value, while the highest value was recorded in the native starch sample. The final viscosity is an indicative parameter that is used to determine starch paste stability (Raji et al., 2022). Amoo et al.(2014) reported that starch with high final viscosity would possess high pasting quality. High final viscosities reported for the succinylated starch samples affirmed that they might have appreciable pasting quality. However, the influence of cooling kinetics on the viscosity and the re-association of molecules of starch in the sample might be responsible for the variation in the final viscosity (Raji et al., 2020).
The setback viscosity values varied from 38.33-80.88 RVU. The sample that was succinylated at 9% had the lowest value, while the native starch sample had the highest value. Setback viscosity determines the re-association of starch molecules, and it measures paste cohesiveness (Ezeocha and Okafor, 2016). However, the low setback viscosities in the succinylated samples showed that they might have a low tendency to retrograde. This also shows that succinylated starch might be useful in food processing and allied industries where low retrograding ability is required, such as bread and other confectionaries (Sanful and Engmann, 2016). High setback value is also associated with syneresis (Adebowale et al., 2005). The high setback viscosity of the native starch sample makes it suitable for the production of gluten-free confectioneries where a high level of retrogradation or staling is required (Ezeocha and Okafor, 2016; Sanful and Engmann, 2016). Clearly, the setback viscosity of the starch samples was reduced by succinylation, thus supporting the claim of Arueya and Oyewale (2015) whose study reported a decrease in setback values of succinylated starch from acha.
The peak time value ranged from 4.17-5.00 minutes and the pasting temperature values ranged from 74.25-84.05°C (Table 3). The lowest peak time was recorded in the sample that was succinylated at 9%, while the native starch possessed the highest peak time. The peak time is a pointer to cooking convenience; however, the shorter the peak time is a reflection of the greater ease of cooking (Adegunwa et al., 2015). Interestingly, it could be inferred that the native starch might experience longer cooking time than the succinylated starch samples. The succinylated starch samples low peak time values implied that they might be good for the production of foods beacuse their processing times are shorter (Raji et al., 2020).
Pasting temperature determines the minimum temperature that is needed to cook any food sample (Olumurewa et al., 2019). It was observed that the native starch had the highest pasting temperature, while the lowest pasting temperature was recorded in the starch sample succinylated at 6%. It is clear from the results that succinylation shortened the pasting temperature of the starch samples, suggesting that starch succinates might cook faster with less energy than the native starch, thereby saving cost and time. However, the lowest pasting temperature at 6% succinylation level suggested that the starch succinate might form paste more easily than other products, and be applicable to food and non-food industrial processes due to conservation of energy and reduced cost (Awoyale et al., 2016).
The results for the proximate composition of the native and modified Anchomanes difformis starches are presented in Table 4. The moisture contents of the modified starch ranged from 7.17 to 12.5% with the native starch having the highest moisture (12.5%), while the least value (7.17%) was observed in the sample that was succinylated at 9%. The moisture contents values were in agreement with the values (7.22-11.22%) reported by Raji et al. (2020) for succinylated wild yam starch samples and (Adewumi et al., 2019) for Anchomanes difformis starch (8.10-10.40%). In addition, the moisture content of both native and succinylated Anchomanes difformis starch samples in this study are in line with the range (10-20%) recommended by Lawal and Adebowale (2005) for commercial starch. Therefore, the starch samples obtained in this study might have long shelf stability due to lower moisture contents which might limit microbial proliferation (Raji et al., 2022).
The fat contents of the native and modified Anchomanes difformis starches ranged from 0.92 to 1.51%, with the native starch having the highest value (1.51%), In comparison, the least fat content (0.92%) was obtained in sample succinylated at 12%. Succinylation of the Anchomanes difformis starch reduced the fat contents of the modified starches. Similar finding was reported by Raji et al. (2020), who observed a decrease in fat contents of succinylated wild yam starch samples (0.18-0.45%). Contrarily, the fat contents (1.01-7.38%) of Anchomanes difformis rhizome starch reported by Abe and Lajide (2014) were slightly higher than those reported for starch samples in this investigation.
The protein contents of the native and modified Anchomanes difformis starches ranged from 3.37 to 3.77%. The native starch sample had the highest protein content (3.77%) while the lowest value (3.37%) was observed in the starch sample whose succinylation was carried out at 12%. Anchomanes difformis starch succinylation decreased the protein contents of the modified starch samples. The non-starch components (fat, protein, and ash contents) of native starch are reduced by succinylation and this makes it greatly useful in some industrial applications (Raji et al., 2020). The native and modified starch samples obtained in this study are less-proteinous with values that are less than 5%. This is in agreement with the findings of Abe and Lajide (2014) who reported protein contents ranging from 1.33-5.33% for hot water treated and untreated Anchomanes difformis starch and also in line with the values (2.30-6.11%) reported by Raji et al. (2020) for modified wild yam starch.
Ash content is expressed as the residue that remains after destroying combustible organic matter (Edima-Nyah et al., 2019). The ash contents of the starch samples varied from 0.57 to 3.67 % with the native Anchomanes difformis starch having the highest value (3.67%), while the least value (0.57%) was observed in the sample that was succinylated at 12%. There was a reduction in the ash contents of the modified starch samples as the level of succinylation increased. The highest ash content in native starch is indicative that it might possess more mineral content than the succinylated starch samples since ash is an index of the mineral content of a food material (Ijeh et al., 2010). The reduction in ash content of the starch succinates might be a result of leaching during succinylation. The ash contents of the starch samples reported in this study are in line with the range of values (1.02-7.38%) reported in the findings of Abe and Lajide (2014) who researched starch from hot water-treated and untreated anchomanes difformis rhizome, but higher than those reported for Icacinatrichantha (0.80%) and (0.30%) Anchomanes difformis starch by Adewumi et al. (2019).
Fibre helps to prevent the re-absorption of bile acids, lowers cholesterol, and prevents the formation of plaques whose components are cholesterol, some fats, and protein (Islam et al., 2021). The crude fiber contents of the native and modified Anchomanes difformis starches ranged from 0.85 to 1.37% with the native starch sample significantly (p<0.05) having the highest value (1.37%). In comparison, the least value (0.91%) was noted in modified starch succinated with 12% succinic anhydride. Succinylation of the Anchomanes difformis starch reduced the crude fiber contents of all the modified starch samples. The crude fiber contents (0.09-0.23%) of wild yam starch reported by Raji et al. (2020) were lower than the values obtained for native and modified starches in this study. The reduction in the crude fiber contents of succinylated starch samples is in agreement with the previous reports (Lawal and Adebowale, 2005).
The carbohydrate contents of the native and modified Anchomanes difformis starches ranged from 75.44 to 86.10%, with modified starch from the sample that was succinylated at 12% having the highest carbohydrate (86.10%). In comparison, the least value (75.44%) from the native starch differed significantly (p≤0.05) from other starch samples. High carbohydrates (>80%) noted in the modified starches showed that succinylation increased the carbohydrate contents of the native starch. The carbohydrate contents of the starches in this study are in agreement with the values (85.53-86.28%) reported for modified wild yam starch in the work of Raji et al. (2020) and values (70.09-88.87%) reported for Anchomanes difformis starch by Abe and Lajide (2014). High carbohydrate contents of the starches in this investigation are advantageous as they may help to provide the energy needed to do work (Ijeh et al., 2010).
Table 5 presents the results for the anti-nutritional factors of native and modified Anchomanes difformis starch samples. Anti-nutrients or anti-nutritional factors can be expressed as those substances that occur naturally in feedstuffs that prevent normal metabolism and absorption of nutrients (Soetan and Oyewole, 2009).
The saponin contents of the native and modified starches ranged from 0.82 to 1.00 mg/100 g. Modified starch from 9% succinylation significantly (p≤0.05) had the highest saponin (1.00 mg/100 g). In comparison, the least value (0.83 mg/100 g) was observed in samples that were succinylated at 3% and 12% and this showed that succinylation at 3 and 12% had no significant change on the saponin content of Anchomanes difformis starch. Succinylation of the native starch reduced the saponin contents of the modified starch but slightly increased the saponin level at 9% succinylation. The saponin contents (5.36-11.76 mg/100 g) of native and modified Anchomanes difformis starches reported by Abe and Lajide (2014) and the values (13.11-20.1 mg/100 g) observed by Senanayake et al. (2013) who worked on four cultivars of yam and cocoyam were higher than those obtained for starch samples in this investigation. The low saponin contents of the starches in this study indicate that they might be less capable of red blood cell damage, enzyme inhibition, and thyroid function intervention (Elekofehinti et al., 2021).
The oxalate contents of the starches ranged between 0.25-0.31 mg/100 g with the modified starch from 9% succinylation significantly (p≤0.05) having the highest oxalate content (0.31 mg/100 g). In comparison, the same least value (0.25 mg/100 g) was noted in samples that were succinylated at 3%, 6%, and 12%. Succinylation of the native starch reduced the oxalate contents of the modified starch samples, but slightly increased the oxalate level at 9% succinylation. Our report corroborates the findings of Raji et al. (2020) who reported a reduction in oxalate contents of succinylated wild yam starch (0.21- 0.31 mg/100 g). The low oxalate contents of the starches in this study infer that they may be incapable of preventing calcium absorption in the body (Kaushik and Cuervo, 2018).
The tannin contents of the native and modified Anchomanes difformis starches ranged from 0.03 to 0.32 mg/100 g. The highest tannin was significantly (p<0.05) noted in modified starch (0.32 mg/100 g) from 3% succinylation, while the least tannin (0.03 mg) was observed in the native starch sample. The tannin contents of the starches in this study conformed with those reported for wild yam starches (0.04-0.08) by Raji et al. (2020). On the flip side, Abe and Lajide (2014) reported (1.0-1.3 mg/100 g) for hot water treated and untreated Anchomanes difformis which were higher than the values obtained for starch samples in this study. Report had shown that tannins might have potential value as cytotoxic and/or antineoplastic agents and also improve the ferment-ability of meal nitrogen in the rumen (Macabeo et al., 2005)). When tannins are consumed excessively, enzymes responsible for protein absorption may be inactivated (Gupta et al., 2015); therefore, the starch samples produced in this study are safe for utilization in food products.
Phytate consumption may lead to lower mineral absorption (Gupta et al., 2015). They may also have a negative impact on the activity of digestive enzymes and act through chelation of mineral cofactors or interaction with protein (Reddy, 2001). The phytates contents of the native and modified starches varied from 0.07-0.59 mg/100 g. Succinylation of the native starch increased the phytate contents of the modified starch. The phytate contents of wild yam starch (0.08-0.13 mg/g) reported by Raji et al. (2020) and (0.08-0.40 mg/100 g) obtained for Anchomanes difformis by Abe and Lajide (2014) corroborate the findings of this study.
The results for the amylose and amylopectin contents of native and modified Anchomanes difformis starches are presented in Table 6. Amylose and amylopectin are polysaccharides contained in starches, with amylose occupying approximately 15-30% of starch, is a linear molecule with α-1,4 glucan linkage, while amylopectin, the major component of starch with 70-85% is a highly branched molecule with α-1,6 glucan linkage (Adejumo et al., 2013; Mayer et al., 2015). The amylose contents of the native and modified starches varied from 11.77-17.18% with the native starch having the highest level of amylose (17.18%). In comparison, the modified starch from 9% succinylation had the least amylose (11.77%). The result showed that succinylation reduced the amylose contents of the native starch. The amylose contents obtained for starches in this study are in agreement with the findings of Adewumi et al. (2019) who researched starches from Icacinatrichantha Oliv and Anchomanes difformis (11.2-15.0%) but lower than the values (49.89-59.70%) reported by Raji et al. (2020) for native and modified wild yam starches. The modified starches moderate amylose contents observed in this study are indicative that their swelling ability might be appreciable if used in food systems (Ikegwu et al., 2009).
Samples (%) | Amylose (%) | Amylopectin (%) |
---|---|---|
0.0 | 17.18±3.781)a | 82.82±3.78b |
3.0 | 16.88±1.82a | 83.12±1.82b |
6.0 | 12.82±1.04b | 87.18±1.04a |
9.0 | 11.77±1.13b | 88.23±1.13a |
12.0 | 14.62±1.13ab | 85.38±1.13ab |
The amylopectin contents of the native and modified Anchomanes difformis ranged from 82.82 to 88.23% with modified starch from 9% succinylation having the highest value (88.23%). In comparison, the lowest value (82.82%) was observed in the native starch. Succinylation of the native starch increased the amylopectin contents of the modified starches. The amylopectin contents of native and modified wild yam starch (40.29-50.11%) reported by Raji et al. (2020) were lower than the values obtained for starch samples in this study. Contrarily, the amylopectin content (92.52%) of Dioscoreadregeana reported by (Afolayan et al., 2021) was slightly higher than those obtained for starch samples in this study. High amylopectin contents of the starch samples directly imply the high swelling power of the respective starch samples (Klunklin and Savage, 2018).
The values obtained for α-amylase, α-glucosidase activities and pancreatic lipase of the starch samples ranged from 16.68-72.12, 49.22-72.87 and -11.52-45.02%, respectively. However, their mathematical expressions, as well as their plots against succinylation levels are revealed in Figs. 3-5 respectively. It was observed that there was an inverse relationship between the α-amylase activity and succinylation levels up to 3% succinylation level. Contrarily, the α-glucosidase activity exhibited a positive linear effect with the succinylation levels up to 3% succinylation level, but declined thereafter. Reasonably, the introduction of the succinyl groups disrupted the starch molecules, thereby limiting the enzymatic activities for starch structural degradation. This is highly desirable particularly for slow-digestible food products production, such as snacks for people suffering from diabetes. A similar finding was established by Marcazzan et al. (1999), who worked on lower α-amylase activity toward succinylated starch, amylase, and amylopectin.
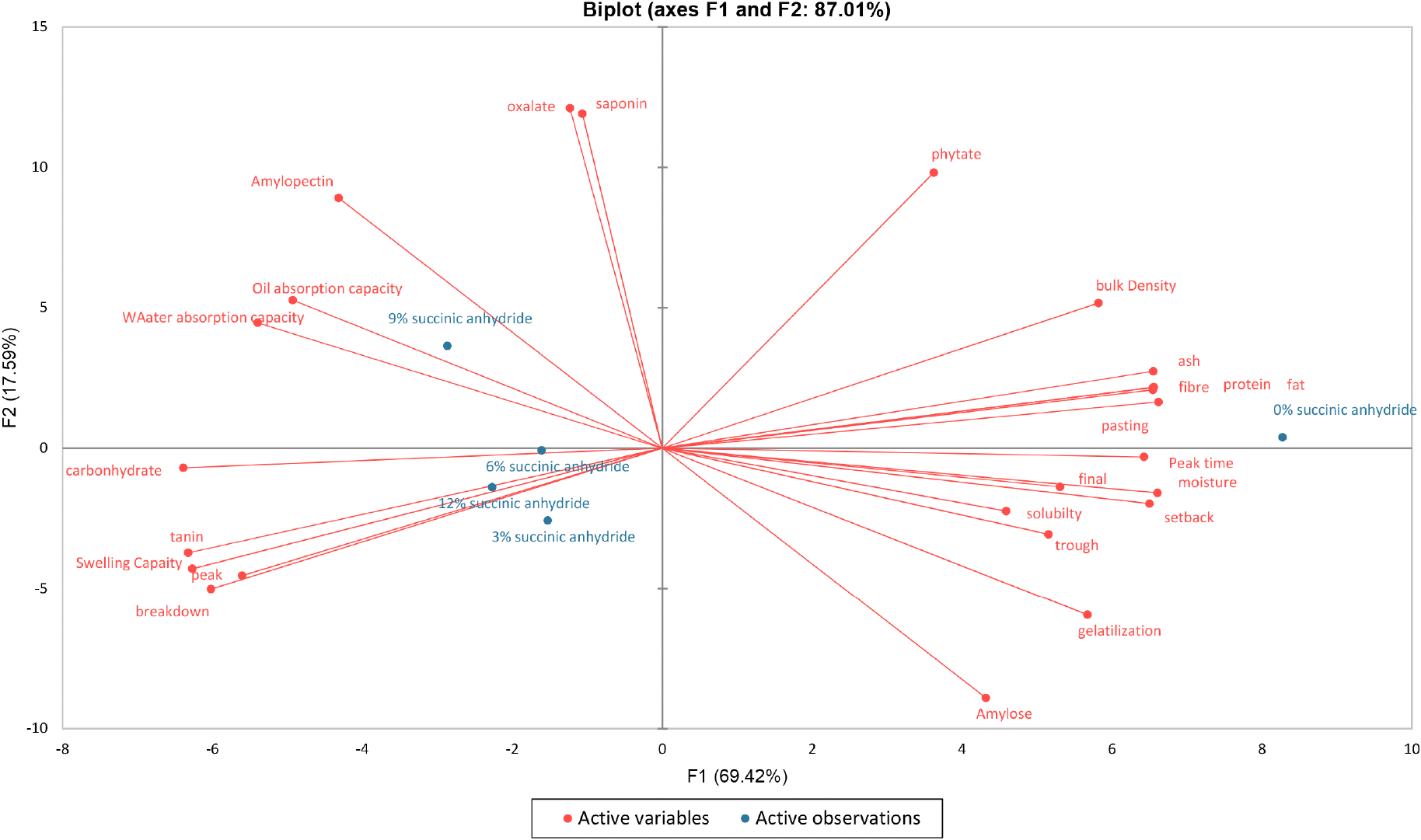
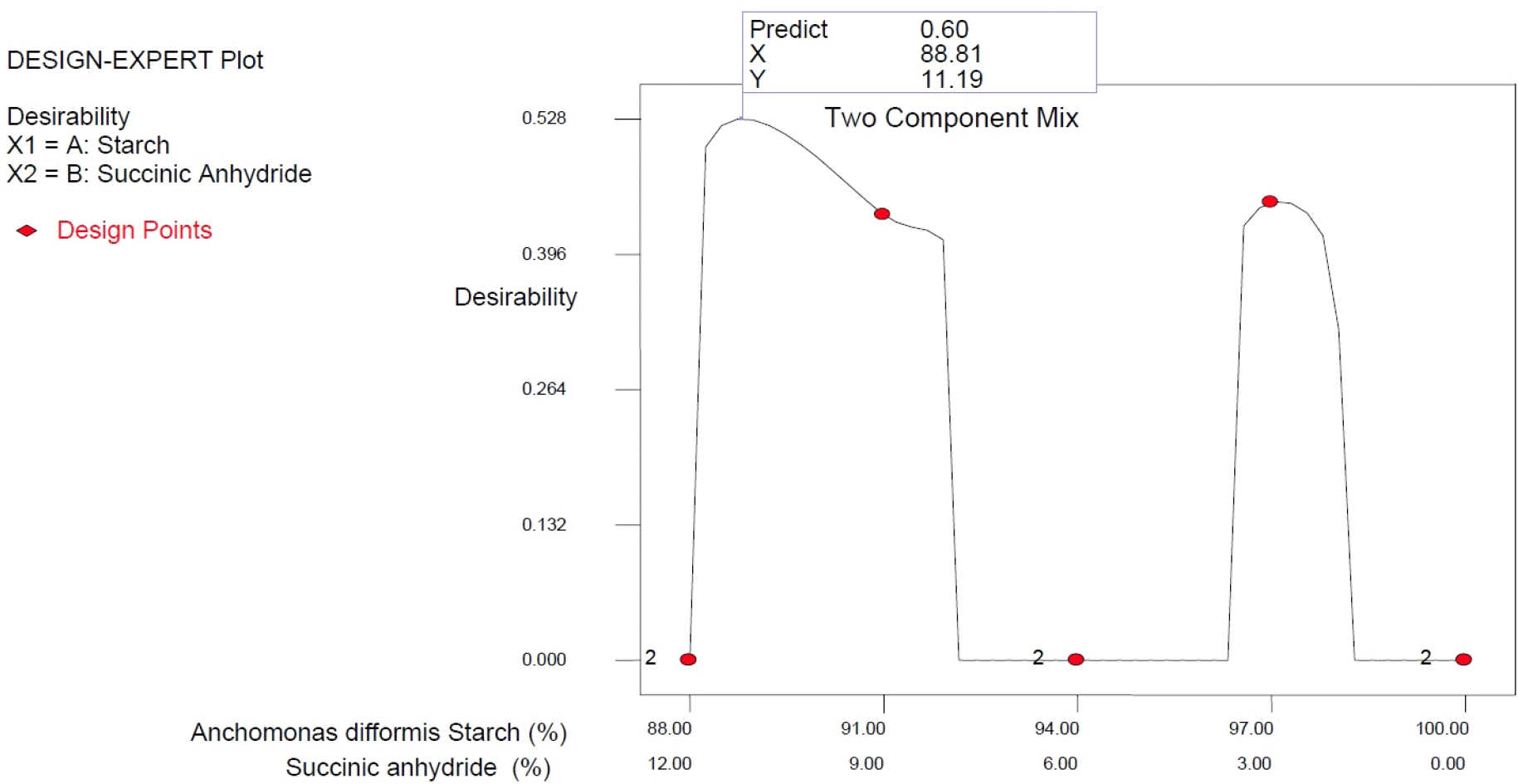
Table 7 reveals the correlation loading of the Anchomanes difformis starch quality determinants. The result implied that the principal component 1 (PC1) was correlated positively with bulk density, gelatinization temperature, and all proximate and pasting properties except carbohydrate, peak viscosity, and breakdown viscosity but negatively correlated with water absorption capacity, oil absorption capacity, swelling capacity, peak viscosity, breakdown viscosity, and tannin content. The PCA biplot, as shown in Fig. 4 allowed us to separate the samples based on the starch quality determinants. The result revealed a data variance of about 87.01%, with PC1 contributing 69.42% and PC2 contributing 17.59%. The Anchomanes difformis starch succinylated at 9% was in the same quadrant with the water absorption capacity, oil absorption capacity, amylopectin contents, saponin and oxalate. Similarly, the starch samples succinylated at 3, 9, and 12% were in the same quadrant with carbohydrate, swelling index, tannin, peak viscosity and breakdown viscosity. Interestingly, the native starch sample was in the quadrant with the fat, protein, ash, crude fibre, pasting time, bulk density and phytate. Therefore, the preference for the starch samples succinylated at 12% might be attributed to the high carbohydrate content, high swelling index, low tannin content, low peak viscosity, and low breakdown viscosity.
Based on the overlay plot of the responses using a simple lattice design for process optimization, Anchomanes starch succinate produced from the combined effect of purified Anchomanes starch of 88.81% and succinic anhydride of 11.19% gave the best mixing combination for producing high-quality starch succinate (Table 8) with the desirability of 60% (Fig. 5).
4. Conclusions
The results revealed that the level of succinylation significantly influenced the Anchomanes difformis starch quality determinants. For the correlation loading results, the predominant principal component of the quality determinants showed a positive correlation with bulk density, gelatinization temperature, and all proximate and pasting properties except carbohydrate, peak viscosity, and breakdown viscosity, but negatively correlated with water absorption and oil absorption capacities, swelling capacity, peak viscosity, breakdown viscosity, and tannin content. However, the preference for the starch samples succinylated at 12% might be attributed to the high carbohydrate content, high swelling index, low tannin content, low peak viscosity, and low breakdown viscosity. Conclusively, the overlay plot of the responses using a simple lattice design for process optimization revealed that Anchomanes starch succinate produced from the combined effect of purified Anchomanes starch of 88.81% and succinic anhydride of 11.19% would give the high-quality Anchomanes difformis starch succinate.