1. Introduction
Cnidium officinale Makino (COM) is a perennial herb of the Apiaceae family that is widely cultivated in China, Japan, and Korea due to its pharmacological properties (Choi et al., 2002; Ma et al., 2024). It has long been recognized in traditional medicine for the treatment of various ailments, such as pain, inflammation, menstrual disturbances, and hypertension (Jeong et al., 2009). In traditional oriental medicine, COM has been used as a folk remedy to alleviate female genital inflammatory diseases (Cho et al., 1996). Additionally, volatile phthalide derivatives of COM have shown diverse pharmacological activities, including sedative, immunomodulatory, anti-anemia, antifungal, smooth muscle relaxant, and anticomplementary properties (Bae et al., 2011; de la Cruz et al., 2013). Recently, Yang et al. (2023) suggested that polysaccharides from COM have the potential to be used as adjuvants in cancer immunotherapy. Furthermore, COM extract has been shown to reduce hypersensitivity in in vivo models of postoperative, neuropathic, and menopausal pain (Lim et al., 2019). Therefore, COM is widely used in Asia in food processing, pharmaceutical, and cosmetic industries due to its diverse pharmacological properties.
Drying herbal plants is a fundamental procedure in postharvest processing that is crucial for preserving the levels of health-beneficial compounds and prolonging storage while maintaining product quality (Kim et al., 2023; Schaarschmidt, 2016; Zhu et al., 2014). However, inappropriate drying processes or conditions may lead to the deterioration of the quality attributes of the final products. For instance, uncontrolled drying conditions, such as natural drying, do not guarantee the quality of the final products or the preservation of health-beneficial compounds (Zhu et al., 2014). Thermal drying is commonly applied to herbal plants in various industrial sectors because of its high efficiency and versatility. However, optimal thermal drying conditions or combinations with other drying technologies are required to ensure the quality of the final products and active levels of health-beneficial compounds (Chumroenphat et al., 2011; Ghafoor et al., 2020; Zagórska and Jaworowska, 2023; Zagórska et al., 2022). In the case of the pharmacological compound ‘paeoniflorin’ in Paeonia lactiflora Pall, higher drying temperatures were found to be detrimental to preserving the compound in the rhizome (Kang and Choung, 1996; Kim et al., 1997). This indicates that appropriate thermal drying conditions should be applied to each herbal plant, especially those containing thermolabile health-beneficial compounds. With the growing interest in the pharmaceutical industry, maintaining the levels of pharmacological compounds in herbal plants has become increasingly important in postharvest processing.
The COM rhizome contains chlorogenic acid, ferulic acid, and ligustilide, which are considered major pharmacological compounds (Baek et al., 2016). Although the rhizomes are generally dried immediately after harvesting and stored for subsequent processing, the effects of thermal drying conditions on these compounds have not been extensively examined. We, therefore, evaluated the impact of various drying temperatures on the levels of pharmacological compounds and antioxidants in COM rhizomes. The results of this study contribute to the optimization of the drying process for herbal plants intended for pharmaceutical use.
2. Materials and methods
COM rhizomes were obtained from a farm in Pyeongchang, Kangwon-state, Korea. The samples were then washed with tap water and cut to 3-5 mm thickness. The cut samples were dried at 30, 40, 50, 60, and 70°C using a thermal air dryer (DY-330H, Lassele, Ansan, Korea) for two days or a freeze dryer (MG-VFD20, MG industry, Gunpo, Korea) for five days. A control sample was dried in the shade at room temperature (RT; approx. 23-26°C) for seven days. The dried samples were finely ground by IKA Multidrive Basic (IKA Korea, Seoul, Korea). The powdered samples were then used to extract pharmacological compounds and antioxidants.
To extract chlorogenic acid, ferulic acid, and ligustilide, 0.1 g of the powdered sample was homogenized in MeOH (10 mL). The mixture was sonicated for 1 h and then centrifuged at 4,000 rpm for 10 min at 4°C. The supernatant was filtered using a syringe filter (0.45-μm pore size) prior to analysis. The filtrated sample was employed to determine the concentrations of chlorogenic acid, ferulic acid, and ligustilide, which were analyzed by ultra-high performance liquid chromatography (UHPLC; Ultimate 3000 UHPLC system, Thermo Fisher Scientific, Waltham, MA, USA) equipped with a Luna®C18 (150×3.0 mm, 3.0 μm) column (Phenomenex, Torrance, CA, USA) maintained at 35°C and UV detection at 284 nm. Isocratic separation was carried out with 0.1% formic acid in water and 0.1% formic acid in acetonitrile at a ratio of 60:40 (v/v) at a flow rate of 0.3 mL/min. The amounts of chlorogenic acid, ferulic acid, and ligustilide in the extract were quantified using a calibration curve constructed using chlorogenic acid, ferulic acid, and ligustilide standards (Sigma-Aldrich, St. Louis, MO, USA).
Total flavonoids and phenolics were extracted from the rhizomes of COM using a previously described method with slight modifications (Ghasemzadeh et al., 2011). The powdered sample (0.2 g) was homogenized in 20 mL of MeOH for 2 h prior to filtration using a filter paper No. 51 (Hyundai Micro, Seoul, Korea) to extract the total flavonoids and phenolics. The level of total flavonoids of the filtered extracts was measured using the method described by Zhishen et al. (1999). A spectrophotometer (Epoch 2, Agilent Technologies, Santa Clara, CA, USA) was used to measure the absorbance at 510 nm of the final solution. The curve of the catechin standard (Sigma-Aldrich) was used to calculate the content of total flavonoids. The level of total phenolics of the filtered extracts was measured using the Folin-Ciocalteu reagent method (Singleton et al., 1999). The absorbance at 735 nm was observed using a spectrophotometer (Epoch 2, Agilent Technologies). The standard curve constructed with gallic acid (Sigma-Aldrich) was used to calculate the total phenolic content.
The powdered sample (0.2 g) was homogenized in MeOH (20 mL) to extract antioxidants. The homogenized mixture was filtered with No. 51 filter paper (Hyundai Micro). The filtrated final extract was used to determine the antioxidant activity of the COM rhizome samples. The DPPH scavenging activity of COM rhizome was examined according to the method described by Stoilova et al. (2007), with slight modifications. The absorbance at 517 nm of the final mixture was recorded using a spectrophotometer (Epoch 2, Agilent Technologies), and the value was calculated to DPPH scavenging activity (%). The ABTS scavenging activity of the COM rhizome was measured according to the method described by Biglari et al. (2008), with slight modifications. The absorbance at 734 nm was measured using a spectrophotometer (Epoch 2, Agilent Technologies), and the value was calculated to ABTS scavenging activity (%).
3. Results and discussion
The levels of major pharmacological compounds (chlorogenic acid, ferulic acid, and ligustilide) in COM rhizomes were previously analyzed simultaneously using HPLC-PDA (Baek et al., 2016). To investigate the effect of the drying conditions on the major pharmacological compounds in the COM rhizome, chlorogenic acid, ferulic acid, and ligustilide were analyzed in the dried rhizomes under each drying condition (Table 1). The highest level of chlorogenic acid was observed at a drying temperature of 40°C, which was significantly higher than that at 60°C. Furthermore, drying at 50°C and RT resulted in lower levels of chlorogenic acid (8.32 and 8.21 mg/g DW, respectively) compared to drying at 40°C, although the differences were not statistically significant. In the case of ferulic acid, the highest level was observed at 40°C; however, no significant differences were found between the treatments. The level of ligustilide in the rhizomes dried at 40°C was significantly higher (3.36 mg/g DW) than that in the other treatments, except for RT. These results indicate that the drying temperature significantly influences the final levels of pharmacological compounds in COM. In this study, drying at 40°C appears to be the most suitable temperature for maintaining the levels of these pharmacological compounds.
Plant phenolics, including flavonoids, are secondary metabolites and antioxidants (Lattanzio, 2003; Panche et al., 2016). To investigate the effect of drying conditions on the levels of total phenolics, total flavonoids, and antioxidant activities, we first tested the effect of different extraction solvents on these compounds and their antioxidant activities (Fig. S1). The results showed that MeOH extract contained significantly higher or comparable levels of these compounds and antioxidant activities compared to 80% acetone, 80% and 50% EtOH, and acidic MeOH (99:1=MeOH:HCl). Therefore, MeOH extract was used for subsequent analyses. The highest level of total phenolics and flavonoids was observed in drying at 40°C and was significantly higher than drying at 30, 50, 60, and 70°C (Fig. 1). Drying at RT presented significantly lower total phenolics and flavonoids compared to 40°C.
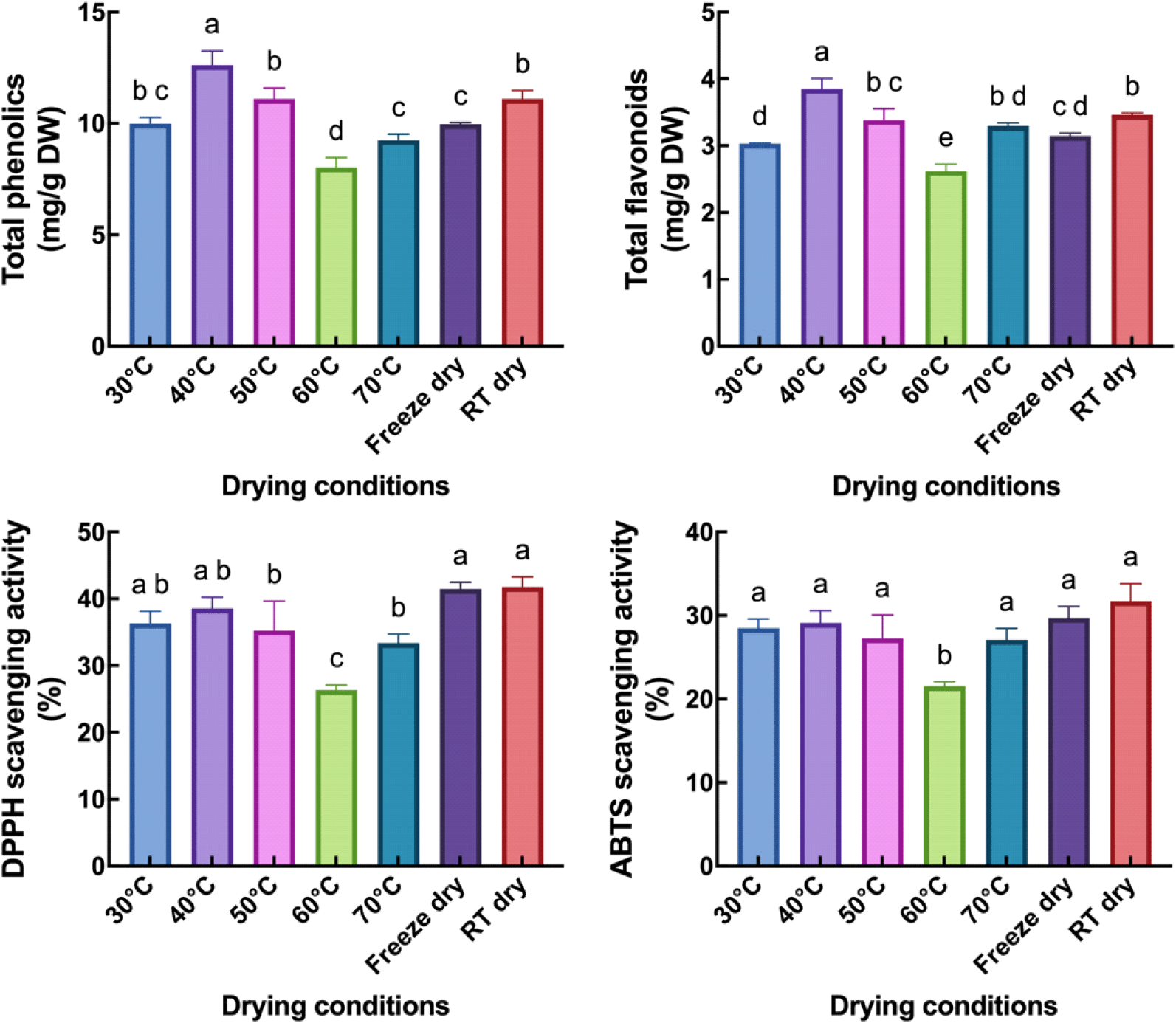
The antioxidant activity was measured using DPPH and ABTS scavenging assays. Interestingly, the highest levels of DPPH and ABTS scavenging activities were observed when drying at RT, although there was no significant difference compared to drying at 30°C, 40°C, and freeze-drying. However, drying at 60°C resulted in a significantly lower antioxidant activity than the other treatments. These findings suggest that health-beneficial antioxidants are unstable at drying temperatures at 60°C. It, therefore, appears that the responses of health-beneficial compounds to drying conditions differ from those of pharmacological compounds.
To evaluate the relationship between the levels of the analyzed compounds, including antioxidant activity, a Pearson’s correlation coefficient test was conducted (Fig. 2). The total phenolics showed a highly positive correlation with total flavonoids, chlorogenic acid, and ferulic acid, which is reasonable because these compounds are phenolic derivatives. Furthermore, the level of ligustilide was positively correlated with the total phenolics. In addition, a strong positive correlation was observed between pharmacological compounds. These findings suggest that the responses of these compounds to drying conditions were similar. However, the antioxidant activity was not highly positively correlated with phenolics, chlorogenic acid, ferulic acid, or ligustilide, suggesting that the level of antioxidant activity may be primarily attributed to other antioxidants that were not analyzed in the present study.
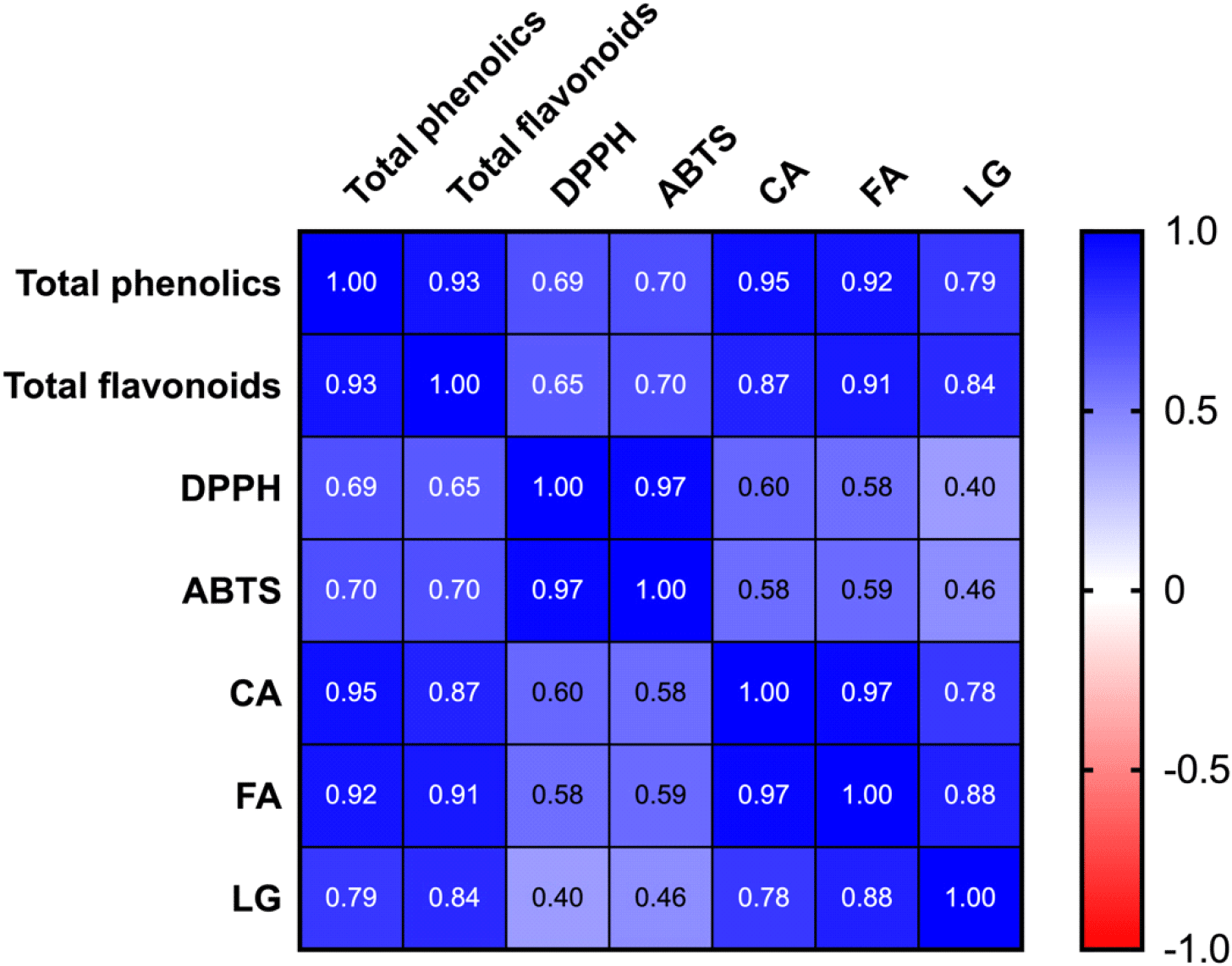
The thermostability of phenolics compounds depends on the type of plant, extract, and processing method. In some cases, thermal processing does not have a detrimental effect on the levels of lycopene, total phenolics, or total flavonoids in tomato paste; in fact, the levels of these compounds increase (Jacob et al., 2010). However, prolonged extraction at higher temperatures reduces the levels of total phenolics and antioxidant activity in jaboticaba peel (de Lima Marsiglia et al., 2023). In addition, the thermolability of individual phenolic constituents in cloudy apple juice varies with increasing thermal treatment time (De Paepe et al., 2014). In this study, increasing the drying temperature negatively affected the levels of chlorogenic and ferulic acids, both of which are phenolic acid derivatives. This suggests that these compounds may be unstable under thermal treatment, even in sliced rhizomes, rather than in the extracts. Ligustilide, a thermolabile phthalide compound (Zhang et al., 2003), also demonstrated thermolabile properties as its levels decreased in rhizome slices with increasing drying temperatures. These results suggest that appropriate drying conditions are essential to maintain the levels of the compounds and produce high-quality final products when drying the COM rhizome during processing. However, the reason for the decrease in the levels of the analyzed compounds after drying at 30°C requires further investigation.
Various primary and secondary plant metabolites exhibit antioxidant properties, including vitamin C, phenolics, flavonoids, and terpenoids (Hounsome et al., 2008). Oh et al. (2010) reported that the aerial parts of COM contain higher levels of total phenolics and flavonoids with antioxidant activity than the rhizomes, indicating that large amounts of secondary metabolites with antioxidant properties are present in the aerial parts of COM. However, although most of the essential oil components are phthalides (approximately 64%), including ligustilide (Choi et al., 2002), γ-terpinene may play a major role in antioxidant activity (Lee et al., 2002). The correlation coefficient values between antioxidant activity and pharmacological compounds were relatively low in this study, even though antioxidant activity tended to decrease with increasing drying temperatures, except at 70°C. Based on the results, it may be inferred that chlorogenic acid, ferulic acid, and ligustilide extracted from the rhizome of COM have lower antioxidant potential, and the levels of other antioxidants and their activities in the rhizome seem to exhibit relatively low responsiveness to varying drying temperatures.
4. Conclusions
Drying of COM is a crucial step in the processing of its final products. In the present study, the effect of drying temperature on pharmacological and antioxidant compounds in COM rhizomes was evaluated, demonstrating the importance of appropriate drying temperature for maintaining the levels of these compounds. Although the levels of these compounds and the antioxidant activity in the freeze-dried and room temperature (RT) dried rhizomes were not markedly different from those in the thermally dried samples, since freeze and RT drying require longer times (about 5-7 days) compared to thermal drying (2 days), thermal drying appears to be a more time-efficient method for processing COM rhizomes. Therefore, the results of this study provide valuable insights for establishing an optimal drying process for COM intended for pharmaceutical use.
The response of pharmacological compounds to temperature variation may vary depending on factors such as drying equipment, sample volume and drying time. In addition, the color and odor characteristics of COM after drying are critical factors in determining the final marketable quality of the dried product. Therefore, further research should focus on practical applications on an industrial scale to ensure consistent quality and efficiency.