1. Introduction
Aflatoxins, toxic metabolites produced by Aspergillus flavus, Aspergillus parasiticus, and Aspergillus nomius, pose severe health risks, notably contributing to hepatocellular carcinoma (HCC) (Fishbein et al., 2024; Wang et al., 2024). Beyond their carcinogenic effects, aflatoxins are mutagenic, teratogenic, neurotoxic, and immunosuppressive, impacting human health significantly (Cao et al., 2022; Jallow et al., 2021; Joint, 2011; Liu et al., 2012). In Nigeria, aflatoxin contamination is common due to factors like high moisture, temperature, and pest infestation, which create optimal growth conditions for aflatoxigenic fungi (Al-Zaban et al., 2023; Šarkanj et al., 2020). These conditions promote aflatoxin production in staple crops such as maize and groundnuts (Mutungi et al., 2016; Yemisi et al., 2023). Ayeni et al. (2021) and Oyebamiji et al. (2024) cited several studies which stated that in Nigeria, aflatoxins frequently contaminate cereals such as rice (Makun et al., 2011; Rofiat et al., 2015); maize (Adetunji et al., 2014; Adetunji et al., 2017; Ogara et al., 2017), millet (Anthony et al., 2014; Ezekiel et al., 2012a) sorghum and groundnut (Ezekiel et al., 2012b; Oyedele et al., 2017; Vabi et al., 2018). In recent years, maize and groundnuts have consistently exhibited high aflatoxin levels. 51% of groundnut samples in Nigeria have been found to exceed the EU aflatoxin limit of 4 ppb for raw food, and many surpass the US limit of 20 ppb (Abass et al., 2017; Ogungbemile et al., 2020; Olaitan et al., 2024; Otsuki et al., 2001).
Several studies in Nigeria, including Yemisi et al. (2023), identified ideal conditions for fungal growth and the production of aflatoxins, which include moisture levels exceeding 14%, an optimal temperature range of 28-30°C, and water activity (aw) values between 0.83 and 0.97 (Adefunke et al., 2023; Alemayehu et al., 2023; Mutungi et al., 2016). Conditions that promote high fungal growth and aflatoxin B1 (AFB1) production were further highlighted in a study by Al-Zaban et al. (2023), to be 28°C and 0.96 aw, with no growth or toxin production at 20°C and lower water activity levels. The degree of aflatoxin contamination of food products is also determined by the integrity of the grains, mold infection levels, activity of pests, and ratio of oxygen to carbon (Dubal et al., 2024; Kutasi et al., 2021; Medina et al., 2015). Aflatoxigenic strains grow mostly on grains in the field and storage. However, methods of aflatoxin contamination control are utilized in the post-harvest stage of crops, but fungal infection occurs mainly at the pre-harvest stage of crops (Callicott et al., 2018; Jallow et al., 2021; Shabeer et al., 2022). As a result, various processes are being explored for their potential to reduce aflatoxins in contaminated crops and their products (Gong et al., 2024; Song et al., 2024). Pre-harvest strategies for reducing aflatoxin levels include selecting resistant varieties, adhering to proper field management practices, and minimizing kernel damage during harvest (Mahuku et al., 2019; Mannaa and Kim, 2017; Nazareth et al., 2024). In Nigeria, a range of processing techniques methods include physical cleaning, heat treatment, solvent extraction, mechanical separation, density segregation, chemical additives, and irradiation, as highlighted by Ayeni et al. (2021). However, complete aflatoxin decontamination cannot be achieved with a single method, and the effectiveness of a combination of methods employed in most traditional food processing has not been thoroughly investigated (Daba et al., 2024; Dahal et al., 2016; Lee et al., 2024; Park et al., 2005; Pleadin et al., 2019; Rodriguez-Amaya and Amaya-Farfan, 2023).
Aflatoxin detection methods, including thin-layer chromatography (TLC), high-performance liquid chromatography (HPLC), and enzyme-linked immunosorbent assay (ELISA), are widely used analytical techniques, each with unique advantages for sample screening and analysis (Iqbal et al., 2014; Reddy et al., 2005). TLC is a versatile method that separates compounds on a thin layer of adsorbent material, making it suitable for rapid, cost-effective screening of many samples (Shekhar et al., 2017). On the other hand, HPLC is a more sophisticated form of column chromatography, where a high-pressure system forces a solvent through a column, resulting in faster and more efficient separation of organic compounds (Kos et al., 2016). This technique offers high precision and resolution for identifying complex mixtures.
ELISA is an immunological method that relies on the specific interaction between antibodies and antigens to detect aflatoxins (Pei et al., 2009). Unlike TLC and HPLC, ELISA is particularly advantageous for screening large numbers of samples due to its simplicity with minimal preparation, speed, and low sample volume requirements (Daba et al., 2024; Poopola and Ehichioya, 2024). ELISA test kits are designed for high-throughput analysis and typically require less sample preparation, streamlining the detection process (Bai et al., 2024). Furthermore, ELISA offers high sensitivity and specificity, making it a reliable option for detecting mycotoxins in foods and feeds (Singh et al., 2024). Although ELISA can be affected by matrix interference, where compounds with similar chemical groups may interact with antibodies and lead to inaccurate results, its overall efficiency, ease of use, and rapid turnaround time make it superior to TLC and HPLC for large-scale screening purposes (Daou et al., 2021; Zhang et al., 2018). Moreover, most chromatographic techniques necessitate solid-phase pre-treatment and immunoaffinity methods to minimize interference and enhance detection efficiency (Wang et al., 2022).
The objectives of this study were to evaluate aflatoxin content and moisture in unprocessed crop and food crop processed by drying using a multi-purpose dryer from Lagos markets, validate the ELISA method for total aflatoxin analysis, and compare aflatoxin levels to permissible limits set by regulatory authorities.
2. Materials and methods
Grains were obtained from Iddo, White Sand, and Mushin markets in Lagos, Nigeria. These markets are the major grain crop wholesale markets in Lagos.
Thirty samples of maize, cowpea, melon, groundnut, and yam chips were randomly purchased from thirty different retail stalls in the three different markets. Samples weighed approximately 650-700 g. Maize, cowpea, melon, and groundnut samples were cleaned by sieving and handpicking to remove stones, discolored grains, and other extraneous materials. After sorting, ten samples weighing 300 g were bulked separately and mixed thoroughly to obtain a homogenous mixture. The conning and quartering method was employed to get an appropriate representative sample for analysis (Campos-M and Campos-C, 2017). Samples were washed and dried (processed) below 50°C for six hours using a Nigerian Stored Products Research Institute (NSPRI) multi-purpose dryer. The representative samples of each sample weighing 10 g were subjected to proximate and aflatoxin analysis.
The unprocessed crop samples were analyzed to assess the impact of moisture content on aflatoxin levels using the gravimetric method (hot-air oven) as outlined by AOAC (2000). Two (2) grams of each sample were weighed and placed into a dish. The dish and the sample were then heated in an oven at 105°C for three hours. Moisture content was calculated using the formula:
Where W1 represents the sample weight (in grams) before drying, and W2 represents the sample weight (in grams) after drying.
Total aflatoxin content was determined in all samples without prior processing (unprocessed) and processed samples. Ten grams of the representatives, all unprocessed and processed, were milled. About 2 g of each disintegrated sample was weighed to ±0.0001 g, extracted with 10 mL of 70% methanol (Sigma-Aldrich, St. Louis, MO, USA) using the method as described by Atehnkeng et al. (2008) then homogenized using Silverson L4R mixer at speed of 7,000 rpm for 10 min at room temperature (25±2°C) using a shaker and filtered through Whatman No. 1 paper. The resultant filtrate was then diluted by mixing 100 μL of the filtrate with 600 μL distilled water in an Eppendorf tube.
The validation of ELISA methods was carried out by determining the recoveries, limit of detection (LOD), and limit of quantification (LOQ), by spiking 2 g of a representative sample at known concentrations of aflatoxins (1, 2, and 6 μg/kg).
The assay followed the protocol outlined in the RIDASCREEN® aflatoxin Total kit (R-Biopharm AG, Darmstadt, Germany). The ELISA kit includes a 96-well plate coated with antibodies, total aflatoxin standards with concentrations 0 (blank), 0.05, 0.15, 0.45, 1.35, and 4.05 ppb, a wash buffer salt (PBS); a conjugate; a solution of monoclonal antibodies to aflatoxin; a substrate/chromogen; a reagent for process termination: stop solution. A 50 μL aliquot of both the diluted sample solution and standard solutions was pipetted into microtiter wells in duplicate. To each well containing either a sample or a standard, 50 μL of aflatoxin-peroxidase conjugate and 50 μL of mouse antibody solution specific to aflatoxin were added. The plate was gently mixed manually and incubated in a dark environment at room temperature for 30 min. During incubation, free aflatoxins and peroxidase-conjugated aflatoxins competed for binding sites on antibodies immobilized on the plate. After incubation, the wells were emptied and washed five times using a wash buffer prepared from the salt-tween solution in the ELISA kit. The residual buffer was removed by tapping the wells upside down on absorbent material. One hundred (100) μL of substrate/chromogen was added to each well, followed by a second 30 min incubation in the dark. The reaction was stopped by adding 100 μL of stop reagent, causing the color to change from blue to yellow. The optical density (OD) was read at 450 nm using a RIDA Chromate ELISA reader (model BDSL, Immunoscan Plus, Lab Systems, Vantaa, Finland). Calibration curves for total aflatoxin were created using standard solutions. Standard curves were constructed based on triplicate analyses of the standard solutions provided in the ELISA kit (Fig. 1) per the manufacturer’s instructions. For the repeatability assessment, total aflatoxin concentrations in six crops were measured in triplicate on two separate days. Samples with concentrations exceeding the highest standard were diluted, and the ELISA procedure was repeated.
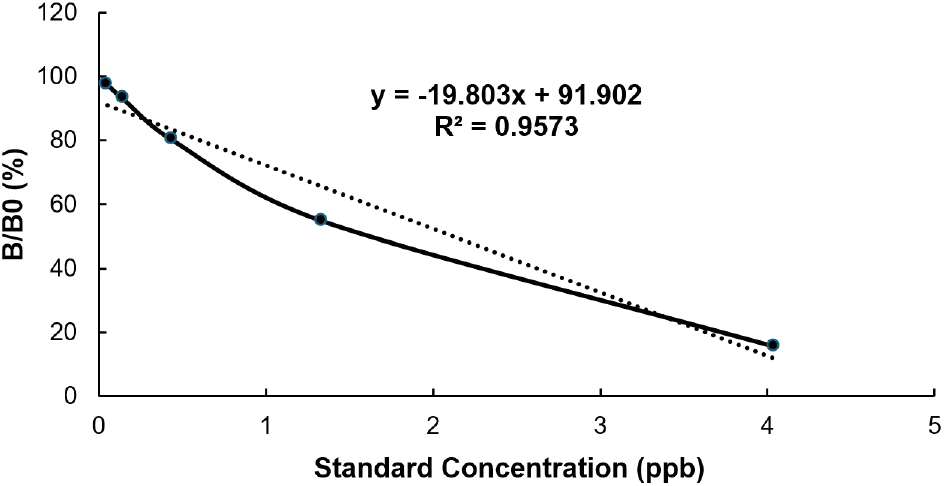
The total aflatoxin data was analyzed using the RIDA® SOFT Win.net software. The aflatoxin content of each sample was determined by extrapolation from the standard curve. Experimental analyses were performed in triplicate, with results expressed as means±SD. Pearson correlation analysis was used to examine the relationship between total aflatoxin content and the moisture content of unprocessed samples. Data was further analyzed using one-way analysis of variance (ANOVA) followed by Tukey’s post-hoc test, conducted with SPSS version 29. A p<0.05 was considered indicative of statistically significant differences.
3. Results and discussion
Melon seeds and maize had the highest moisture content, as shown in Table 1. This does not align with a study by Saka et al. (2022) conducted in the Maiduguri metropolis to survey aflatoxin contamination levels in cowpea, maize, melon, groundnut, yam chips, and fish with cowpea and fish having the highest moisture content. However, similar moisture content was reported in a study by Omohimi et al. (2019) on yam chips collected from markets in Saki Lagos State and Bodija in Oyo State. According to the research “Keep Stored Grain Cool, Dry during Summer (NDSU Agriculture, 2024)”, the recommended moisture content for maize at 13.5% for optimal long-term storage. In this study, the moisture content in the maize was lower than the recommended moisture content. However, the moisture content of the melon seeds is consistent with that reported in a study by Okokon (2002), with a value of 7.4%. Also, the moisture content of groundnuts was far below 44.8%, as cited by Obi and Offorha (2015). These indicated the effectiveness of using the multi-purpose dryer. High moisture levels could be attributed to inadequate drying of crops before bagging, which is one of the significant causes of aflatoxin contamination in grains.
Samples | Moisture content (%) |
---|---|
Cowpea1) | 5.18±0.10a3) |
Maize1) | 6.31±0.12b |
Dried melon seeds1) | 7.70±0.10c |
Groundnut1) | 4.01±0.12d |
Yam chips2) | 6.20±0.10b |
Results showed no significant variation among the groups. Based on these findings, the mean concentrations, standard deviations, and recovery rates of the spiked samples were calculated. LOD and LOQ were determined by analyzing 25 blank mixture samples and calculating the average concentration measured and the standard deviation (SD). The following equations were used:
The method demonstrated satisfactory accuracy and repeatability at concentrations of spiked sample crops. The Recoveries in this study were between 66-80%, with an LOD parameter of 1.87 μg/kg and LOQ of 3.41 μg/kg (Table 2). The LOD and LOQ in this study were higher than that of several studies by Han et al. (2024), 62.7 ng/kg and 121 ng/kg, respectively, which analyzed aflatoxin using ELISA, while recoveries in this study by Han et al. (2024) 72-94%. However, these results were sufficient to detect aflatoxin levels above the legal permissible limit in the staple crops. The standard curve obtained from the results demonstrated a clear correlation between absorbance and the prepared concentrations of the standards. This correlation of the recovery and validation indicates the precision and accuracy of the ELISA method.
The aflatoxin contents of the unprocessed samples ranged from 3.10-10.20 μg/kg (Table 3). In contrast, samples selected for processing (maize, cowpea, dried melon seeds, and ground nut) ranged from 2.00-5.10 μg/kg. This indicated that processing such as washing and drying can be applied as a way of managing aflatoxin contamination as the results indicated aflatoxin levels significantly decreased, p<0.05 (Fig. 2). The yam chips were not processed as its initial production requires the processing of fresh yam by drying into yam chips. Total aflatoxin contents in unprocessed samples were higher than in the corresponding processed whole grains. Different governing bodies set different legislations on the permissible limit of aflatoxin. The EU has firm requirements that require items for direct human consumption to have a total aflatoxins content of less than 4 ppb in both unprocessed foods and processed foods (European Commission, 2009; European Commission, 2010) except for maize which is usually subjected to sorting or other physical treatment before human consumption for which a maximum level of 5 μg/kg for aflatoxin B1 and 10 μg/kg for aflatoxin total has been established.
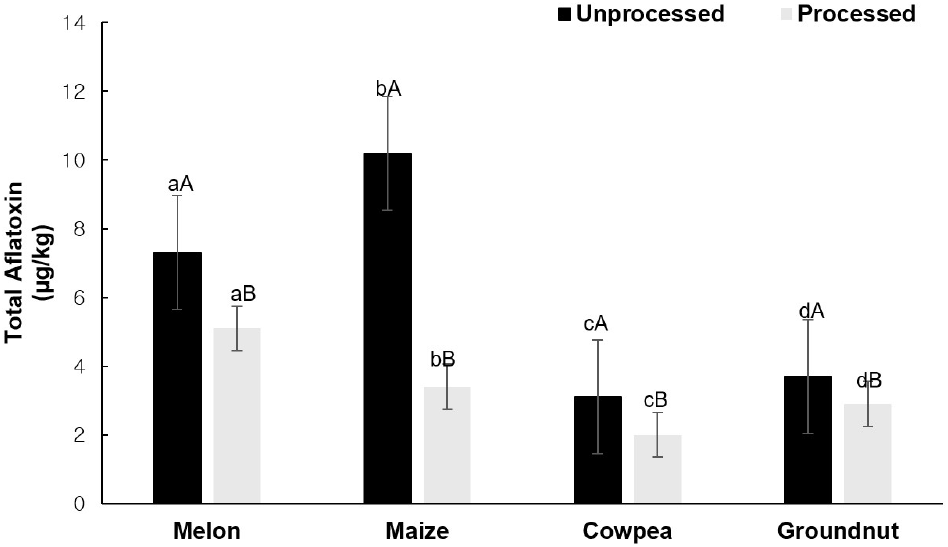
On the other hand, the United States Food and Drug Administration has established the allowed limit for total aflatoxin intake not to exceed 20 μg/kg (FAO, 2004). While in Nigeria, acceptable thresholds are given by NAFDAC for raw food (yet to be prepared) of 10 μg/kg and 4 μg/kg for cooked/ready-to-eat food (Felagha et al., 2016).
The total aflatoxin content of unprocessed and processed crop samples (Fig. 2) indicated in this study was highest in maize (Table 3) and above the acceptable threshold as directed by the EU and NAFDAC (Awuchi et al., 2020; Johnson et al., 2020) The total aflatoxin content of the unprocessed cowpea and processed cowpea was below the permissible limit for consumption set by all legislations.
There is no significant relationship between the moisture content and the total aflatoxin content of each processed sample at p<0.05 (Table 4) after using the multi-purpose dryer except in dried melon seeds, which have a strong correlation between the moisture content and the total aflatoxin content. High levels of aflatoxin have been cited in several studies; a study by Obani et al. (2019) analyzed six types of Colocynthis citrullus (L.) traditionally called “egusi” from Southwestern Nigeria, collected between 2012 and 2013, for aflatoxin levels and presence of Aspergillus. A. flavus was the most common contaminant, with an average prevalence of 51.1±2.4%, and aflatoxin levels ranging from 6.9-109.5 μg/kg for aflatoxin-B and 0.9-35.8 μg/kg for aflatoxin G, often exceeding permissible limits. The results indicate that “egusi” is highly susceptible to aflatoxin contamination, posing a potential health risk to consumers.
The average aflatoxin contamination was compared to those of other studies. Among the unprocessed grain samples, high concentrations of total aflatoxins were detected in maize. This was in correlation with a study by Kaaya and Kyamuhangire (2006), which reported aflatoxin levels above 20 μg/kg in maize kernels from Uganda after 6 months of storage. Similarly, Daniel et al. (2011) observed a considerably higher concentration of aflatoxins in domestically cultivated maize in Kenya than in maize imported as relief.
The Total aflatoxin present in unprocessed cowpea samples was 3.7 μg/kg, which was <4 μg/kg. According to Telles et al. (2017), phenolic chemicals, especially gallic and chlorogenic acids, can potentially inhibit fungal amylase activities, which might explain the low amount of aflatoxin contamination seen in the bean samples. Pagnussatt et al. (2013) reported that the combined influence of several chemicals in beans can provide a protective barrier against the growth of toxic species. A limited number of cases have been reported in literature where aflatoxins have been found in red kidney beans, split peas, chickpeas, and cowpeas (Lutfullah and Hussain, 2012).
Aflatoxin contamination in food commodities from these markets in Lagos can be said to be due to increased temperatures and dry conditions driven by climate change, resulting in conditions in crops that favor Aspergillus flavus infection in the farm and further proliferation during the post-harvest period (Bandyopadhyay et al., 2016; Kamika et al., 2016). In addition, high aflatoxin contamination levels can be compounded by other farm practice factors, including high planting densities, infertile soils, poor weeding, poor crop rotation, and delays in harvesting time. Poor storage of agricultural produce can also cause rapid aflatoxin contamination because of the development of aflatoxin-producing fungi, which has been demonstrated by several authors (Azziz-Baumgartner et al., 2005; Mwalwayo and Thole, 2016). Certain socioeconomic circumstances, such as inadequate understanding of suitable pre- and post-harvest management techniques, inadequate transportation modes, unavailability of needed materials, poor government regulations, legislations, informal marketing systems tools and equipment, may also cause contamination by aflatoxins (Asemoloye et al., 2017; Christiana et al., 2022). In recent times, nanoparticles and nanocomposites have been cited to reduce aflatoxin contamination, according to a study by Zhang et al. (2024), which used magnetic reduced graphene oxide composite (Fe3O4@rGO) synthesized through hydrothermal fabrication to exhibit high efficiency in adsorbing aflatoxin B1, following the Langmuir model and preserves the nutritional quality of treated foods, making it a promising solution for food safety. The properties of nanocomposites that make them effective in mitigating aflatoxin contamination have been cited in several studies (Akinniyi, 2023; Akinniyi et al., 2024; Sun et al., 2021).
The application of the multi-purpose dryer in controlling total aflatoxin levels in essential crops has shown promising results in this study. Beyond its effectiveness, the efficiency of the dryer makes it accessible to retailers and farmers alike, spanning various scales of operation. Its adaptability to rural settings, where kerosene or gas can serve as heat sources, underscores its practicality and widespread applicability (Ntwali et al., 2021). This versatility positions the dryer as a food safety solution and a sustainable agricultural practice, aligning with global efforts towards safer and economically viable food production. Farmers and most retailers can integrate this technology into their operations, ensuring safer food products reach consumers while optimizing economic viability (Romuli et al., 2019).
The preparation procedure in immune-enzymatic methods is very simple. It consists mainly of extraction, and there is no need for purification or isolation of the tested component (Daou et al., 2021). The low number of ELISA methods found in the literature indicates its low application in Nigeria, making literature comparison difficult. The ELISA method presents a more economical means of monitoring aflatoxin contamination in Nigeria.
The occurrence of aflatoxin in indigenous food items from Lagos might challenge food security, public health, and economic advantages. To substantially decrease aflatoxin contamination in agricultural commodities, it is crucial to encourage the implementation of suitable techniques for managing crops before and after harvest (El-Sebaii et al., 2012). These strategies should be promoted among all participants in the food value chains, particularly farmers and processors. However, much like crop commodities, mitigation strategies must be protected by further knowledge about the sources of contamination and potential regional variations in contamination levels (Gowda et al., 2007). The multi-purpose dryer employed in this study fabricated by NSPRI operates through regulated heat supplied by a stove with either kerosene as a fuel source and airflow, promoting uniform drying across all crop parts which are usually placed on a net racking system with at least four layers in an enclosed system and thus, mitigates contamination risks associated with traditional sun drying (Kamaldeen and Okedokun, 2022). Unlike sun drying, the multi-purpose dryer significantly reduces drying time, lowering the crop’s exposure to environmental contaminants, pests, and fungal spores that increase aflatoxin production. Its adaptability to renewable energy sources, like solar power, also enhances sustainability, making it a viable option in regions with limited access to conventional energy (Nagle et al., 2008). Though the initial cost may be higher, the multi-purpose dryer’s durability and efficiency yield long-term economic benefits by reducing crop losses, improving product quality, and increasing farmer income potential. The multi-purpose dryer ability to operate with alternative energy sources, such as kerosene or solar panels, reduces its environmental footprint (Asemu et al., 2020). Lowering crop spoilage rates conserves land, water, and other agricultural resources, ultimately supporting sustainable agricultural practices.
Additionally, by minimizing aflatoxin contamination, the dryer helps prevent foodborne health risks, contributing to food safety and economic security in Nigeria agricultural sector (Oyebamiji et al., 2024). Addressing aflatoxin contamination in Nigeria is crucial to public health and food security. ELISA provides a rapid, cost-effective method for monitoring aflatoxin levels across crops, complementing the multi-purpose dryer role in reducing contamination during drying (Nguyen-Van-Hung et al., 2019). This enhances the country’s ability to ensure safer food supplies while supporting sustainable agricultural practices.
4. Conclusions
In conclusion, integrating the multi-purpose dryer represents a pivotal step in managing aflatoxin levels in food crops in Lagos, Nigeria. While ELISA-based testing is highly effective for detecting aflatoxin contamination, its large-scale application is costly and time-consuming. Distributing portable ELISA kits to farmers and vendors could offer a more practical, cost-effective solution, enabling early, on-site detection and faster responses to contamination risks. A coordinated framework involving key stakeholders like local authorities, farmers, and NAFDAC would further enhance monitoring, with regular testing along the value chain. Establishing data hubs to centralize aflatoxin information and linking ELISA kits to mobile apps could allow real-time reporting, facilitating rapid response. Although the multi-purpose dryer offers significant benefits, its initial cost and energy demands present challenges, especially for smallholder farmers. Subsidies, loans, and cooperative use could make this technology more accessible, and training programs for local technicians would address maintenance needs in rural areas. Integrating alternative energy sources, like solar or biofuels, could further enhance its reach.
Additionally, processing crops before market entry significantly reduces aflatoxin exposure by degrading toxic forms, thus improving food safety. However, considering the regional focus of this study on Lagos, Nigeria, and the sample size involved, caution is necessary when extrapolating these findings to a national level. Future research with more prominent and representative samples is essential to validate the applicability of these conclusions across Nigeria. To safeguard public health, government, and agricultural stakeholders must collaborate to integrate ELISA into food safety practices, ensuring sustainable aflatoxin management tailored to regional contexts and, where appropriate, extending insights globally.