1. Introduction
As modern dietary habits increasingly emphasize meat consumption and the global population is projected to exceed 9 billion by 2050, the demand for meat is expected to rise correspondingly (Cohen, 2001). To meet the growing demand, the livestock industry must increase meat production. However, livestock farming significantly impacts the environment, as cattle grazing occupies 24% of the Earth’s land and consumes one-third of the world’s grain production. Additionally, livestock manure releases methane, carbon dioxide, and nitrous oxide, contributing to environmental pollution (KBSA, 2005). The livestock industry also faces ethical concerns, as practices such as overcrowded farming, the use of antibiotics, and growth hormones often neglect animal welfare, causing physical and psychological stress to animals (Egon et al., 2023).
These environmental and ethical issues have led some individuals to adopt veganism (Oliver, 2024), with many vegan consumers citing unethical treatment of animals as a primary motivation (Pearce et al., 2024). From an environmental perspective, veganism is chosen for its potential to decrease greenhouse gas emissions, water usage, etc. (Asano and Biermann, 2019). Furthermore, studies indicate that individuals who consume meat have a higher risk of heart disease (6.67% for men, 2.62% for women) and stroke (8.06% for men, 4.05% for women) compared to vegetarians (Jeong, 2011). Plant-based proteins not only aid appetite regulation and improve blood triglyceride levels (Kim, 2018) but are also lower in fat and calories and contain various bioactive compounds such as polyphenols (Cho and Ryu, 2022). Plant-based meat analog is increasingly recognized as sustainable protein sources and are regarded as a viable food option for the future (Jones, 2016).
In this context, meat analog, which replicate the sensory and nutritional properties of conventional meat using sustainable protein sources, has gained attention (Delgado-Pando et al., 2023). Production technologies for meat analog include extrusion process, 3D printing, and cultured meat (Calton et al., 2023; McClements, 2023). The extrusion process integrates multiple processing stages—mixing, kneading, grinding, heat treatment, protein structuring, shaping, and drying—into a single machine, reducing operational costs and improving cost efficiency (Abilmazhinov et al., 2023). It enables rapid production of meat-like fibrous structures from plant proteins through high-temperature, short-time processing, leveraging heat, pressure, and shear forces (Gu et al., 2017; Ryu, 1995). The fiber structure of proteins can be tailored by adjusting raw material composition and extrusion process variables (Ryu, 2003). Additionally, extrusion process allows the use of food by-products, reducing wastewater, minimizing environmental impact, and enhancing nutritional quality (Abdullahi et al., 2021).
Global coffee consumption has consistently increased, with approximately 140 million bags (1 bag=60 kg) consumed in 2011, 150 million bags in 2014 (ICO, 2015), 165 million bags in 2017, 168 million bags in 2020, and 179 million bags in 2022 (ICO, 2023). However, only 0.2% of coffee beans are used to produce beverages, leaving 99.8% as spent coffee grounds (Choi et al., 2021). With rising coffee consumption, the amount of spent coffee grounds has also increased. Spent coffee grounds contain high carbon content, producing methane—21 times more potent as a greenhouse gas than carbon dioxide—during decomposition (Roychand et al., 2023). Discarded spent coffee grounds require significant oxygen for decomposition and contain caffeine, tannins, and polyphenols that can be toxic to soil (Mussatto et al., 2011). Rich in carbohydrates (approximately 45%), lipids (13%), and proteins (13%), spent coffee grounds also contain antioxidants like chlorogenic acid, caffeine, and melanoidin, offering health benefits such as antioxidant, antimicrobial, and anticancer properties. They are also a source of dietary fiber and minerals like potassium and magnesium, making them suitable for enhancing food nutritional quality (Cavanagh et al., 2023; Andrade et al., 2022). According to Mitra et al. (2024), the addition of spent coffee grounds (SCGs) to bread and pasta significantly increased dietary fiber content while enhancing protein and mineral density. Similarly, SCGs have been valorized through supercritical carbon dioxide extraction of oil, with the defatted residue being used to develop functional cookies exhibiting bioactive and prebiotic properties (Sharma et al., 2021). In terms of physical properties, the abundant fiber in SCGs also improves physical characteristics by retaining moisture, which reduces water loss in products and extends shelf life. Moreover, SCGs have been utilized as a food-grade ingredient in bakery products, demonstrating antioxidant dietary fiber, thermal stability, and potential to mitigate chronic disease risks (Martinez-Saez et al., 2017). From a chemical perspective, Silva et al. (2018) highlighted that SCGs exhibit antioxidant activity, as demonstrated by DPPH and ABTS assays, making them excellent candidates as antioxidants in the food industry. Despite these advancements, research on SCGs has primarily focused on their application in bakery or functional food products, leaving a significant gap in understanding their potential use in meat analogs. Additionally, the Food Code provided by the Ministry of Food and Drug Safety (MFDS, 2024) specifies that parts of the coffee fruit, excluding the pulp, are permissible for use as food ingredients. While numerous studies have explored upcycling spent coffee grounds for industrial applications, such as biodiesel production (Caetano et al., 2012) and use as adsorbents (Lee et al., 1998), limited research has focused on utilizing spent coffee grounds to enhance food value (Kim, 2008). By incorporating spent coffee grounds, this study evaluated the physicochemical properties of low-moisture extruded meat analogs to explore their upcycling potential.
2. Materials and methods
The raw materials used in this study were isolated soy protein (Pingdingshan TianJing Plant Albumen Co., Ltd., Henan, China), wheat gluten (Roquette Freres, Lestrem, France), corn starch (Samyang Co., Ulsan, Korea), and spent coffee grounds (Gravity Factory Co., Cheonan, Korea).
An inline co-rotating twin-screw extruder (THK31-No. 5, Incheon Machinery Co., Incheon, Korea) was employed for this experiment. The screw diameter was 30 mm, with a length-to-diameter ratio (L/D) of 23:1. The screw configuration of the extruder is shown in Fig. 1. The processing parameters were set as follows: feed rate of 100 g/min, moisture content of 35%, screw speed of 200 rpm, and barrel temperature of 150°C. The formulation of raw materials for low-moisture extruded meat analog with varying levels of spent coffee grounds is presented in Table 1.
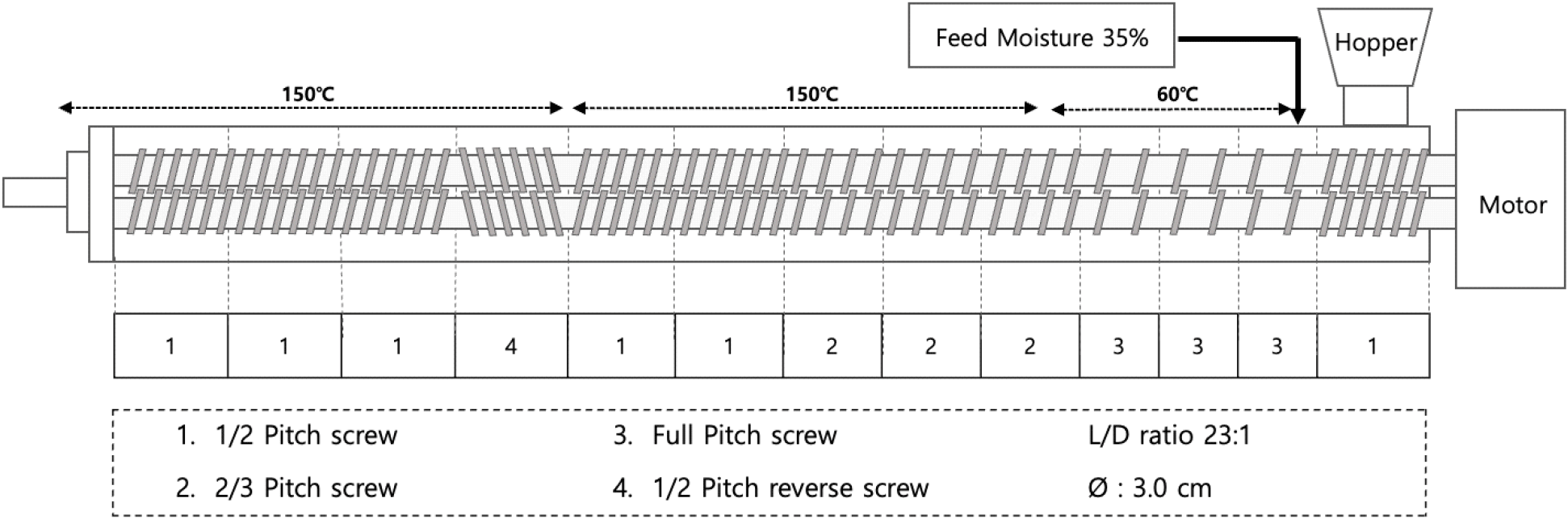
SCGs content (%) | ISP2) | WG3) | CS4) |
---|---|---|---|
0 | 50 | 40 | 10 |
5 | 47.5 | 38 | 9.5 |
10 | 45 | 36 | 9 |
The ground low-moisture meat analog analyzed for color using a colorimeter (Chroma Meter CR-300, Minolta Co., LTD., Osaka, Japan). The lightness (L), redness (a), and yellowness (b) values were measured three times, and the average values were reported. The color difference (ΔE) was calculated using Equation (1), based on the standard color plate values of L*=87.01, a*=0.67 and b*=18.20.
To measure the water holding capacity (WHC) and oil holding capacity (OHC) of low-moisture meat analog, the method described by Gu and Ryu (2017) was adapted. Alternative meat samples cut into 1×1 cm cubes were hydrated in a water bath (SHWB-45, Lab House, Pocheon, Korea) set to 90°C for 30 min. After removing excess water, the weight was recorded, and WHC was calculated using Equation (2). Similarly, for OHC, the alternative meat was immersed in oil in the same water bath (90°C) for 30 min, after which excess oil was removed at room temperature. The weight was then measured, and OHC was calculated using Equation (3).
The texture properties of hydrated low-moisture meat analog were measured using a texture analyzer (Z0.5 TS, Zwick Roell, Ulm, Germany). Samples cut into 1×1 cm cubes were hydrated in a water bath (SHWB-45, Lab House) set to 90°C, and excess water was removed before testing. The parameters of springiness, cohesiveness, and chewiness were measured with a 10 cm diameter probe under a maximum force of 600 N, repeated 10 times. These properties were calculated using Equations (4), (5), and (6), respectively, based on the formulas adapted from Trinh and Glasgow (2012).
Shear force was measured using a probe (70×3 mm) to evaluate both the parallel and vertical cross-sections of the samples under a maximum force of 600 N, repeated 10 times. Shear force values were calculated using Equation (7), and the degree of texturization, based on shear force, was calculated using Equation (8).
D1: Distance of first occurred maximum stress
D2: Distance of second occurred maximum stress
A1: Area of first occurred maximum stress
A2: Area of second occurred maximum stress
CSV: Cutting strength of vertical direction
CSP: Cutting strength of parallel direction
To quantify the structural integrity of low-moisture meat analog under high-temperature and high-pressure conditions, the integrity index was measured by adapting the method of Gu and Ryu (2017). Four grams of meat analog samples were added to 100 mL of distilled water and hydrated in a water bath (SHWB-45) set to 90°C for 30 min. The samples were then pressurized and heated at 121°C for 15 min. After cooling under running water, the samples were homogenized at 17,450 rpm for 1 min using a homogenizer (IKA-T10B, IKA Co., Seoul, Korea). The homogenized samples were filtered through a 20-mesh sieve, and the retained residues were dried at 105°C for 10 h. The weight of the dried residues and the sample weight were used to calculate the integrity index using Equation (9).
The nitrogen solubility index (NSI) of the low-moisture meat analog samples was measured using a modified method from Căpriță et al. (2010). To measure soluble nitrogen content, 0.1 g of ground meat analog sample was added to 5 mL of 0.5% KOH solution and stirred at 120 rpm in a shaking incubator (SI-300R, Jeiotech, Seoul, Korea) set to 30°C for 30 min. The mixture was centrifuged at 3,000 rpm for 30 min, and 0.05 mL of the supernatant was collected to determine soluble nitrogen content using the ninhydrin method described by Starcher (2001).
For total nitrogen content, 0.1 g of the sample was hydrolyzed in 5 mL of 6 N HCl at 100°C for 24 h. The hydrolyzed sample was then dissolved in 5 mL of distilled water, centrifuged at 3,000 rpm for 30 min, and 0.05 mL of the supernatant was used to measure total nitrogen content using the ninhydrin method. NSI was calculated using Equation (10).
The DPPH radical scavenging activity of the low-moisture meat analog samples was assessed using a modified method from Brand-Williams et al. (1995). One gram of the meat analog sample was extracted with 10 mL of 80% ethanol for 2 h and centrifuged at 3,000 rpm for 30 min. Subsequently, 0.1 mL of the supernatant was mixed with 3.9 mL of methanolic DPPH solution in the dark for 30 min. The absorbance was measured at 515 nm, and DPPH radical scavenging activity was calculated using Equation (11).
A0: Absorbance of the blank
A: Absorbance of the sample
ABTS radical scavenging activity was determined using a modified method from Re et al. (1999). One gram of ground meat analog sample was mixed with 10 mL of 80% methanol and extracted at 30°C in a shaking incubator (SI-300R, Jeiotech) set at 200 rpm for 2 h. The extract was centrifuged at 3,000 rpm for 30 min, and 20 μL of the supernatant was collected.
An ABTS radical solution was prepared by reacting 7 mM ABTS with 140 mM potassium persulfate (Sigma Aldrich Co.) for 12 h in the dark. The solution was diluted with distilled water to achieve an absorbance of 0.6-0.8 at 760 nm. Diluted ABTS solution (100 μL) was reacted with 20 μL of the sample extract for 15 min. The absorbance was measured at 760 nm using a microplate reader. ABTS radical scavenging activity was calculated using Equation (12), where Asample represents the absorbance of the sample reacting with ABTS solution.
The sensory evaluation of meatballs containing spent coffee grounds (SCGs) was conducted with 40 undergraduate students from the Department of Food Science and Technology at Kongju National University. The study was approved by the Institutional Review Board (IRB) of Kongju National University (IRB approval number: KNU_IRB_2024-018). To minimize bias, meat analog meatballs without spent coffee grounds, those with added spent coffee grounds, and meatballs made with pork were each assigned random numbers. They were served in identical paper cups along with bottled water. The sensory evaluation criteria included appearance (1=not appetizing, 9=very appetizing), color (1=not appealing, 9=very appealing), flavor (1=very bad, 9=very good), texture (1=very bad, 9= very good), taste (1=very bad, 9=very good), and overall acceptability (1=very bad, 9=very good). A 9-point hedonic scale was used, with pork meatballs serving as the control group. Three types of meatballs were prepared (low-moisture plant-based meatballs without SCGs (0%), low-moisture plant-based meatballs with 5% SCGs, and pork-based meatballs). Breadcrumbs and milk were added in equal amounts to both plant-based and pork meatballs, and the seasoning was adjusted with salt and pepper. The meatballs were then cooked in olive oil over medium-low heat for six minutes.
3. Results and discussion
The cross-sectional and longitudinal views of low-moisture extruded meat analog containing spent coffee grounds are shown in Fig. 2. During extrusion, the rapid release of internal pressure to atmospheric pressure leads to steam formation and puffing due to moisture phase change (Flarup-Knudsen, 2003). As a result, the cross-sections of the meat analog exhibit a porous structure (Cho and Ryu, 2018). As the spent coffee grounds content increased, a reduction in pore size was observed in the meat analog (Fig. 2). This can be attributed to the insoluble dietary fibers such as cellulose, hemicellulose, and lignin present in spent coffee grounds (Wang et al., 2023). The increased viscosity from these fibers reduces puffing, consistent with findings from Deng et al. (2023). This study also suggests that the insoluble dietary fibers in spent coffee grounds contributed to the reduction in pore size of the low-moisture meat analog.
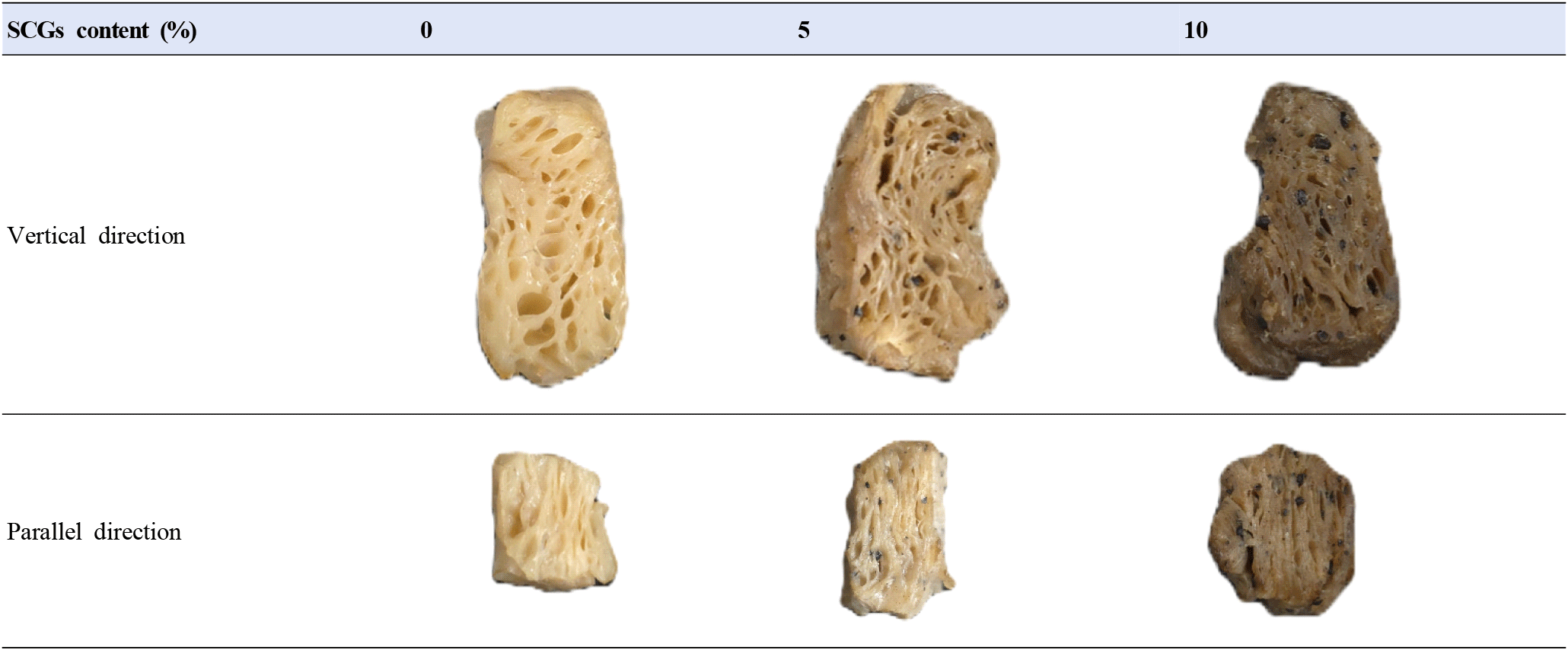
The lightness (L), redness (a), and yellowness (b) of the meat analog containing spent coffee grounds are presented in Table 2. The control sample without spent coffee grounds was set as the standard color reference, and the ΔE values for each sample are shown in Table 2.
Comparing the color properties before and after extrusion, the lightness (L) decreased, while the redness (a) and yellowness (b) increased. This change is attributed to the Maillard reaction between amino acids in soy protein isolate and wheat gluten and reducing sugars in corn starch during high-temperature extrusion (Iwe et al., 2004). Additionally, the dark brown color of roasted coffee is due to non-enzymatic browning reactions, oxidation of phenolic compounds to quinones, and subsequent oxidation, polymerization, and condensation reactions forming melanin (Seong et al., 2014). When spent coffee grounds content increased from 0% to 10%, the lightness and blueness decreased due to these properties of spent coffee grounds. The ΔE values confirmed that extrusion and increased spent coffee grounds content led to greater browning and higher color change rates.
The WHC of the meat analog, closely related to their structure (Gu and Ryu, 2019), is shown in Table 3. As the spent coffee grounds content increased from 0 to 10%, the WHC decreased from 6.60±0.08 g/g to 4.01±0.11 g/g. This reduction is attributed to the decreased pore size observed in the appearance section, which reduced the available surface area for water absorption (Hwang et al., 2023). The insoluble dietary fibers in spent coffee grounds, including cellulose, hemicellulose, and lignin, reduced puffing and decreased the pores capable of retaining water (Deng et al., 2023; Wang et al., 2023).
SCGs content (%) | Water holding capacity (g/g) | Oil holding capacity (g/g) |
---|---|---|
0 | 6.60±0.081)a | 1.90±0.20a |
5 | 4.80±0.27b | 1.68±0.05a |
10 | 4.01±0.11c | 1.70±0.08a |
The OHC values of the meat analog are also shown in Table 3. No significant differences in OHC were observed between the control and samples with added spent coffee grounds. Despite reduced puffing and surface area in higher spent coffee grounds content, the high lipid content in spent coffee grounds absorbed oil, resulting in no significant differences in oil absorption (Sakouhi et al., 2024). Additionally, based on studies linking OHC to lipid oxidation (Sarabandi et al., 2023), it was determined that spent coffee grounds did not influence lipid oxidation or rancidity in the meat analog.
The texture properties of meat analog with added spent coffee grounds are presented in Table 4. Springiness and cohesiveness showed no significant differences with increasing spent coffee grounds content, while chewiness exhibited an increasing trend. Soluble dietary fibers absorb water to form hydrophilic colloids, increasing dough viscosity and promoting interactions among water, starch, and gluten proteins (Guadarrama-Lezama et al., 2016). These interactions enhance gluten matrix formation, encapsulate starch molecules, and strengthen the gluten network (Li et al., 2024). In this study, the soluble dietary fibers in spent coffee grounds interacted with gluten proteins and starch, strengthening the bonds and increasing chewiness. Chewiness, influenced by springiness, cohesiveness, and hardness, increased primarily due to heightened hardness with added spent coffee grounds. These findings align with previous studies reporting increased hardness with higher spent coffee grounds content (Jung and Kang, 2011).
Cutting strength also showed an increasing trend with higher spent coffee grounds content. Since cutting strength represents the force per unit area required for cutting, its increase was attributed to the higher chewiness of meat analogs. These results are consistent with prior research showing a positive correlation between chewiness and cutting strength (Jeon et al., 2022; Sin et al., 2024). Texturization degree presented in Table 4 increases with the addition of spent coffee grounds. (0%: 1.23±0.02, 5%: 1.73±0.11, 10%: 1.74±0.07). This suggests that spent coffee grounds promotes protein alignment and structure formation. Spent coffee grounds, rich in dietary fibers such as cellulose and hemicellulose (Campos-Vega et al., 2015), serve as a substrate for reinforcing fibrous structures. The porous structure of spent coffee grounds also acts as a scaffold, enhancing the formation of denser fibrous structures in the meat analog (Jang and Lee, 2024; Yasemin, 2025).
The Integrity Index is a parameter that measures the degree of internal bonding in meat analog subjected to high-pressure and high-temperature conditions (Ning and Villota, 1994). The Integrity Index of meat analog containing spent coffee grounds is presented in Table 5. The Integrity Index was 64.96±3.18 for 0% spent coffee grounds, 67.31±2.07 for 5%, and 67.09±0.54 for 10%, showing no significant differences between the samples with spent coffee grounds and the control. This result suggests that spent coffee grounds did not significantly affect the binding or structuring of low-moisture meat analog. Although textural properties such as chewiness and cutting strength increased, the spent coffee grounds were not effective in maintaining structural binding in high-temperature and high-pressure conditions.
SCGs content (%) | Integrity index (%) | NSI (%) |
---|---|---|
0 | 64.96±3.18a1) | 23.63±2.99a |
5 | 67.31±2.07a | 16.43±2.26b |
10 | 67.09±0.54a | 16.77±2.15b |
The nitrogen solubility index (NSI) quantifies the degree of protein denaturation in extrudates by measuring the soluble protein content in water (Park et al., 2016). Table 5 shows the NSI values for meat analog with spent coffee grounds. NSI decreased from 23.64±2.99% (0% coffee grounds) to 16.43±2.26% (5%) and 16.77±2.15% (10%). Unlike previous findings that NSI is inversely correlated with the Integrity Index (Gu and Ryu, 2019), no such correlation was observed in this study. The reduction in NSI may be due to the binding of proteins, polysaccharides, and dietary fibers in spent coffee grounds during extrusion, which likely reduced the nitrogen solubility by limiting its leaching.
To assess antioxidant activity, DPPH and ABTS assays were performed. The DPPH radical scavenging ability is widely used to detect antioxidants by neutralizing free radicals that cause oxidation (Jeon et al., 2022). ABTS radical scavenging ability measures antioxidant potential over a broad range by reacting with both hydrophilic and lipophilic compounds (Munteanu and Apetrei, 2021). The antioxidant activities of low-moisture meat analog with spent coffee grounds are summarized in Table 6. The results showed an increasing trend in DPPH and ABTS radical scavenging abilities with higher spent coffee grounds content, attributed to the presence of caffeine and chlorogenic acid in spent coffee grounds (Brzezińska et al., 2023). Chlorogenic acid is known for its potent antioxidant effects, including inhibition of lipid oxidation and reduction of malondialdehyde levels in plasma and LDL (low-density lipoprotein), thus reducing cardiovascular disease risk. Furthermore, chlorogenic acid exhibits antiviral effects against pathogens like herpes and HIV (Kaplia et al., 2024). These results suggest that spent coffee grounds can serve as a valuable antioxidant source in functional food applications.
SCGs content (%) | DPPH radical scavenging activity (%) | ABTS radical scavenging activity (%) |
---|---|---|
0 | 10.62±2.141)c | 5.09±0.43c |
5 | 53.10±3.28b | 21.25±0.57b |
10 | 84.31±0.43a | 44.58±1.15a |
The sensory evaluation of meatballs made with spent coffee grounds is shown in Table 7. No significant differences were observed in color and appearance between the control and meat analog, indicating that spent coffee grounds-added meat analog closely mimics real meat. In terms of taste and texture, the control group had the highest values. While spent coffee grounds addition improved these attributes, the differences were not significant. The higher scores in the control group are likely due to the inclusion of fats that enhance binding and provide characteristic meat flavor. Overall acceptability was highest in the control group at 6.98±1.69, with no significant difference between the 0% and 5% spent coffee grounds meatballs.
4. Conclusions
This study analyzed the physicochemical properties of low-moisture meat analog with varying spent coffee grounds content and conducted sensory evaluations of meatballs made from them. The base formulation consisted of 50% isolated soy protein, 40% wheat gluten, and 10% corn starch, with spent coffee grounds added at 0, 5, and 10%. The extrusion parameters were set at 100 g/min feed rate, 35% moisture content, and 200 rpm screw speed. Increasing spent coffee grounds content decreased pore size, thereby reducing water holding capacity, but had no significant effect on oil absorption, indicating no influence on lipid oxidation or rancidity. The color measurement results showed that as the amount of spent coffee grounds increased, the dark brown color became more intense, and the lightness decreased. Antioxidant activities increased with higher spent coffee grounds content, suggesting their potential as a functional ingredient due to the presence of bioactive compounds commonly found in spent coffee grounds. Texture analysis results show that as the amount of spent coffee grounds increased, chewiness and cutting strength improved, and the addition of spent coffee grounds enhanced the degree of texturization. However, Integrity Index values showed no significant differences, likely due to the inability to maintain structural binding under high-temperature, high-pressure conditions. NSI decreased significantly with spent coffee grounds addition, indicating increased protein binding. Sensory evaluation showed no significant differences in color, appearance, or flavor between the control and spent coffee grounds meat analog, supporting their potential as meat mimics. The findings suggest that spent coffee grounds can enhance the physicochemical properties of low-moisture meat analog and serve as a sustainable raw material, reducing waste and environmental impact. The spent coffee grounds used in this study were a blend of Brazil Cerrado, Guatemala Antigua, Colombia Huila, and Kenya AA beans. Therefore, the findings cannot be generalized to all coffee varieties, and further research on spent coffee grounds from various coffee beans is required.